Abstract
Inchcailloch hosts a small serpentine (ultramafic) site in Loch Lomond that is unusual in the Scottish context in being covered with broad-leaved woodland; along with serpentinite, serpentine breccias and sandstones are also found. In order to look at plant-soil relationships in more detail here, I analysed soil and foliar chemistry from sampling locations on and around the ultramafic outcrop. Soils situated directly on the outcrop had elevated concentrations of metals such as Co, Cr, Mg, Mn, Ni and Zn and a lower Ca-to-Mg ratio. Foliar elemental composition was influenced more by species than sampling location although some metals (Ni and Mn) were at greater concentrations in plants on the ultramafic outcrop. Foxgloves (Digitalis purpurea) had the highest foliar Ni concentration as well as Al and Zn, indicating an ability to accumulate multiple metals. Overall, the high Ca-to-Mg ratio indicating the calcareous nature of the soils due to mixing of soils from contrasting geologies, the low metal concentrations, and the high soil P are all considered to act together to prevent this site from exhibiting some of the more extreme features, such as skeletal soils and sparse vegetation cover, found at other Scottish serpentine sites.
Introduction
Serpentine, or ultramaficFootnote1, soils are derived from ultramafic rocks that have high concentrations of metals such as magnesium, iron and nickel. Such soils are also often lacking in the nutrients most important to plant growth: nitrogen, phosphorus and potassium, and regularly have a poor water holding capacity (Proctor and Woodell Citation1975; Kazakou et al. Citation2008; Harrison and Rajakaruna Citation2011; Konečná et al. Citation2020). Serpentine soils have been described from around the world where their distinctive edaphic features provide unique challenges to the plants of these areas and some sites are spectacularly barren of vegetation (Roberts and Proctor Citation1992). However, a complex range of factors interact to influence serpentine vegetation (Tsiripidis et al. Citation2010; Lazarus et al. Citation2011) and it is not always the case that an ultramafic geology leads to a unique, or even unusual, vegetation composition. There are likely to be a number of reasons for this including the nature of the soil’s physicochemical characteristics that will be influenced by the time since last glaciation, and the diversity and characteristics of the local species pool, among others. For example, D’Amico and Previtali (Citation2012) considered that well-developed Alpine soils with dense forest vegetation had undergone more intense leaching of magnesium and nickel relative to calcium in agreement with the work of Alexander (Citation1988) in California. In contrast, Chiarucci et al. (Citation1998) found that wooded communities had greater metal concentrations than garigue communities in Italy, suggesting that soil metals may not be playing a key role in vegetation development and major plant nutrient concentrations may be more influential. Tyndall (Citation2012) considered that soil depth and particle size distribution were more important factors influencing the distribution of serpentine woodland and grassland than soil metals in eastern North America. An additional reason for the limited expression of characteristics typical of serpentine ecosystems may be where there are closely associated, and contrasting, geologies that reciprocally influence one another (Kanellopoulos et al. Citation2015) or where non-ultramafic inclusions are present in otherwise serpentine ecosystems that influence the mineral content of the soils derived from them (McGahan et al. Citation2008, Citation2009).
The highest density of serpentine outcrops in the UK is found in the Scottish Highlands and islands including Meikle Kilrannoch in Angus, parts of the island of Rhum, and the Keen of Hamar in Shetland where there is poor vegetation development but a preponderance of rare plant species (Proctor Citation1992). However, there are additional Scottish serpentine sites that do not appear to show any particularly unusual vegetation characteristics or plant species with less extreme edaphic differences when compared to non-serpentine sites. Inchcailloch, an island in Loch Lomond, is such a site and is somewhat unusual in that it is a UK serpentine site covered by broadleaved woodland. Two studies were published in the 1970s on the plant communities of the island (Tittensor and Steele Citation1971; Horrill et al. Citation1975) along with a soil report (Hornung and Mew Citation1970), but little work has been done since then. Tittensor and Steele (Citation1971) presented some evidence that there was an association between the plant communities and the serpentine soils on the Loch Lomond islands but this was not found in a more intensive study by Horrill et al. (Citation1975) that focussed on the island of Inchcailloch alone. On Inchcailloch, the soils may have a mixed origin due to the co-existence of serpentine bedrock and other geologies which may go some way towards explaining the clear lack of a serpentine effect on the vegetation; there is, however, little data to support this suggestion.
The aim of this study is to examine the chemistry of the serpentine and associated soils and relate this to patterns of leaf elemental concentrations from a small number of locations on Inchcailloch with a focus on the serpentine soils. I hypothesise that although this serpentine site may have a less extreme soil chemistry than other more barren sites due to the variable and closely located geologies, differences between plants growing on metal-rich serpentine soils and those on adjacent non-serpentine soils will still be evident. Therefore, the goal of this investigation is to study less extreme serpentine sites, such as Inchcailloch, which will allow us to learn more about the ‘serpentine syndrome’ by comparing them with the more barren serpentine sites and thus provide us with an improved knowledge of the relative importance of various factors leading to unique serpentine ecologies.
Study site
Inchcailloch is an island near the south-eastern shore of Loch Lomond within Loch Lomond and the Trossachs National Park in southern Scotland (). Mean annual precipitation is around 1500 mm with a mean annual temperature of 8.5 °C. Inchcailloch is about 56 ha in area and its long axis lies in a south-west to north-east direction having two main ridges along this axis with the highest point 85 m a.s.l. The more northerly of these ridges is called Church Ridge (highest point c. 55 m) and this is where the Highland Border Complex runs through the island leading to the ultramafic geology. Associated with the ultramafic serpentinites are conglomerates of serpentinite and other ophiolitic pebbles enclosed within limestone (breccia). North and south of the fault on the island are sandstones (Goodenough et al. Citation2008; Bluck Citation2015). A geological map of the island is available in Bluck (Citation2015). The majority of the island is covered in deciduous woodland (Quercus petraea (Matt). Liebl., Sorbus aucuparia L., Betula pendula Roth, Alnus glutinosa (L.) Gaertn. etc.; ) with a very small area of Scots pine (Pinus sylvestris L.). The community is closest to W16 in the UK National Vegetation Classification (Hall et al. Citation2004). Although woodland has been found in the area for at least 7000 years, much of Inchcailloch was coppiced in the 18th and 19th Centuries (TittensorCitation1970) and at least some of the island was likely cultivated prior to this indicating its semi-natural nature.
Figure 1. (a) Location of the island of Inchcailloch, in Loch Lomond, within the UK, (b) location of sampling points on the island, and (c) view of the woodland on the island.
Figure Footnote: (a) © Crown copyright and database rights 2020 Ordnance Survey (100025252) (b) Source: Esri, DigitalGlobe, GeoEye, Earthstar Geographies, CNES/Airbus DS, USDA, USGS, AeroGRID, IGN, and the GIS User Community.
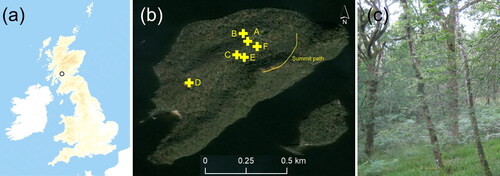
Materials and methods
Geological maps are not explicit as to the exact placement of the ultramafic geology so, in July 2012, soil samples were taken from six locations at least 40 m apart () around this area (to a depth of 10 cm). These were analysed for pH (1:2.5 ratio in deionised water), loss-on-ignition (550 °C for 5 h), and total C and N (LECO TruSpec CN analyser). Total elemental concentrations of P, K, Ca, Mg, Co, Cr, Mn, Ni and Zn were assessed by microwave-aided digestion in nitric acid, dilution in deionised water and analysis on a Thermo iCAP 6300 Duo ICP-OES). ‘River clay sediment’ (LGC6139) was used as the certified reference material with a mean recovery of 75% for the elements of interest. Extractable P was determined by a Mehlich 1 extraction and analysis by ICP-OES as above, and extractable Ni was by a 0.1 M EDTA extraction and analysis on a Varian SpectrAA 220FS AAS, whilst exchangeable Mg and Ca were determined by leaching with 1 M ammonium acetate and analysed by AAS as above.
At each of the soil sampling locations, leaf samples were collected from trees and understorey plants (2–10 per location, 35 in total); all leaves were produced in the current growing season. Their elemental concentrations were determined using a LECO elemental analyser for N (for some tree species only) and a microwave-aided nitric acid digestion and analysis by ICP-OES (as above) for all other elements. Additional samples (12) were taken from along the summit path (yellow line on ) and analysed as above. Leaves were not washed prior to analysis; it is acknowledged that washing can remove soil particles (e.g., Oliva and Valdés Citation2004) and a trial with four species found on Inchcailloch showed reductions in Al, Cr and Fe following washing in deionised water (), however there was no evidence of soil contamination of unwashed samples analysed in the full dataset following the indications of Reeves and Kruckeberg (Citation2018).
Table 1. Soil chemical characteristics of six soil samples (0-10 cm depth) from the island of Inchcailloch, Loch Lomond, Scotland (see for sampling locations).
A principal components analysis (PCA) on the correlation matrix of scaled and centred elemental concentrations was conducted to represent the change in multivariate element concentrations among species and sampling locations. The PCA was supported by a perMANOVA (adonis) including species and sampling location as factors. Individual nutrient concentrations were compared among species using a one-way ANOVA (with log transformation if required) followed by a Tukey’s test. Relationships between plant and soil elemental concentrations were examined with Spearman’s rank correlations.
Results
Soils
Soils were acidic as expected for such woodlands (mean pH of 4.3) and contained about 18% organic matter. Although available soil P concentrations were low (< 22 μg g−1), total P concentrations were high (< 1030 μg g−1; ). The two samples (E and F) that were situated on the top of Church Ridge showed the strongest serpentine properties with elevated total concentrations of Co (< 83 μg g−1), Cr (< 944 μg g−1), Mg (< 18,500 μg g−1), Mn (< 1980 μg g−1), Ni (< 1250 μg g−1) and Zn (< 157 μg g−1) as well as EDTA-extractable Ni (< 23 μg g−1; ). Whilst the exchangeable Ca and Mg concentrations, and the Ca-to-Mg ratio, were very variable, the mean Ca-to-Mg ratio was above one indicating the generally calcareous nature of the soils; one of the Church Ridge samples (F) had notably low Ca leading to a Ca-to-Mg ratio of 0.1 (). Samples A and B were similar to one another and likely derived largely from sandstone, Sample C had high Ca as well as moderately high Ni and was likely derived from serpentinite breccia; all of these samples had higher total metal concentrations than Sample D.
Plants
Most of the foliar elemental concentrations differed between species (except Cr, Co, Fe and Ni), much of which was due to differences between trees and understorey plants with trees having greater Ca and Mn but less K and Zn (; p < 0.05 in all cases). However, there were some remarkably high Mn concentrations with Corylus avellana L., Quercus petraea and Sorbus aucuparia exhibiting at least one individual with over 2000 μg g−1, which was higher than foliar P in some cases. All foliar Cr concentrations were less than 1 μg g−1 and all foliar Co concentrations were less than 3.5 μg g−1 (with about one-third not being detectable; ). Mean foliar Ni was 5.3 μg g−1 and the maximum was 37 μg g−1 in Digitalis purpurea L.; this species also had the greatest foliar Al (; 127 μg g−1) and Zn concentrations (; 294 μg g−1) that was actually greater than the total soil Zn, i.e., having a bioconcentration factor greater than one ( and ). About 90% of the individuals had a Ca-to-Mg ratio greater than unity with a mean value of 1.8.
Table 2. Mean (± standard error) (not for the first three species as these are a single sample only) foliar elemental concentrations of eleven plant species from the island of Inchcailloch, Loch Lomond, Scotland.
Plant-soil relationships
The principal component analysis () showed negative loadings (> 0.3) on the first axis (that explained 25% of the variance) for (in order) Al, Zn, Mg, Ca, Zn and Cr whilst the second axis (that explained 18% of the variance) showed positive loadings for K and P and negative loadings for Ca, Mn and Mg. Foliar elemental composition grouped more by species than by sample location (Species: r2 = 0.69, p < 0.001; Location: r2 = 0.07, p = 0.13; ). However, there were some differences between the locations for two elements: foliar Ni and Mn were higher in the two locations with more notable serpentine characteristics with weak, but statistically significant, positive correlations across all samples between foliar and soil Ni (r2 = 0.38, p < 0.05) and Mn (r2 = 0.35, p < 0.05).
Discussion
The soil and foliar elemental characteristics are described from the island of Inchcailloch, which hosts a little-studied serpentine site in Scotland. At this site, the serpentinite-derived soils are closely located with conglomerates, breccias and sandstone and it appears that truly distinctive serpentine soils are found only on the crest of Church Ridge and, even here, the influence on the vegetation is minimal as a densely wooded community occurs with no clear floristic distinction between the woodland further down the slopes. There are a number of potential reasons for this lack of a noticeable serpentine effect. The main one is likely to be the calcareous nature of the soils, as already noted by Proctor (Citation1992), with a mean soil Ca-to-Mg of more than one. This will be due to the influence of other bedrock types closely associated with the serpentinite and is in contrast to most serpentine soils that contain more Mg than Ca, sometimes an order of magnitude more (Brooks Citation1987). Nevertheless, soil Ca was remarkably variable among the samples and the soil Ca-to-Mg ratio of one of the samples of top of Church Ridge was 0.1, which was very low. This low value compares with Proctor et al. (Citation1991) who found a Ca-to-Mg of 0.1 on the clearly skeletal serpentine debris at Meikle Kilrannoch in Angus, but there the Mg concentration was (much) greater than on Inchcailloch and therefore likely much more toxic (Proctor Citation1971). This variability in Ca is likely due to geological factors (McGahan et al. Citation2008, Citation2009) as associated with the serpentinite is a serpentinite in limestone breccia that will have weathered and been mixed together during soil formation. The second reason for the lack of a noticeable serpentine effect is the moderate-to-high total P concentrations that were comparable to Lime Hill (Stirlingshire) (Johnston and Proctor Citation1979) or Irish grasslands and heathlands (Brearley Citation2018). The third reason is the generally low total concentrations of soil metals such as Ni (< 1250 μg g−1) or Cr (< 944 μg g−1). Although the concentrations of Cr were quite high, Ni was low for serpentine soils, and a much smaller proportion of Cr normally makes it into the soil available phase thus reducing its toxicity (Brooks Citation1987). Proctor and Woodell (Citation1971) found a higher total soil Ni of 1500–3500 μg g−1 on grassland of western Loch Lomond that is a more open site, and there are other sites around the UK also with higher Ni concentrations (Proctor Citation1992).
In terms of foliar elemental concentrations, they were all generally sufficient for adequate plant growth (Pugnaire, Citation2001). Of course, serpentine soils have an unusual chemical composition, but foliar Co, Cr, Mg and Ni were all low when compared with other UK serpentine sites (Proctor Citation1992) and comparable to Irish grasslands and heaths with minimal serpentine effects (Brearley Citation2018; Brearley et al. Citation2020). Proctor (Citation1992) presented an extensive dataset on foliar elemental concentrations in UK serpentine sites, and boreo-temperate ecosystems have a much lower occurrence of hyperaccumulator species than Mediterranean or tropical ecosystems (Teptina et al. Citation2018) so the generally low foliar metals are not unexpected.
There was some indication that soil chemistry influenced foliar chemistry but there was no significant grouping of foliar chemistry by sampling location, although a more balanced sampling design would have been able to test this better. The strongest soil influence on foliar chemistry was for Ni, which was higher in the two clearly serpentine locations on top of Church Ridge – this has also been seen in Irish heathland (Brearley et al. Citation2020) where foliar Ni was the only element to be greater at a site with only a mild serpentine effect. Nevertheless, there were some interesting interspecific patterns emerging in terms of foliar elemental concentrations. The greater foliar Ca concentration in trees compared to other life forms may represent an evolutionary heritage linked to a distinct physiology but, if ubiquitous, a greater Ca requirement for trees may be one of the reasons for their absence in generally Ca-poor serpentine soils. The high foliar Ni, Zn and Al in Digitalis purpurea is interesting and, along with the high Cd found by Barrutia et al. (Citation2011) at an old mining site in Spain, is worthy of further study as these metals are all potentially toxic and this species could be at the early stages of evolving metal accumulation strategies such as some Noccaea species (Hanikenne and Nouet Citation2011). Interestingly, Plantago, within the same family, also has the ability to accumulate a range of metals (Al, Cu, Fe, Pb and Zn) to high concentrations (Serrano et al. Citation2017). The high concentration of foliar Mn (< 3300 μg g−1) in some tree species could be considered toxic in some instances (Lambers et al. Citation2015), but Mn accumulation can be common in temperate tree species. For example, earlier work by Mayer and Gorham (Citation1951) found comparable Mn concentrations but, in common with this study, variability among individuals was high. I have also found similarly high foliar Mn in a woodland in north-west England (FQB, unpubl. data) and Davey et al. (Citation2007) found high Mn in oak litter across a number of sites in Wales. The high Mn in these trees’ leaves is probably due to a combination of highly variable soil Mn concentration (two orders of magnitude by EDTA-extraction; data not shown) combined with an acidic rhizosphere with reducing conditions. Manganese has been shown to exacerbate Ni toxicity (Williams Citation1967) which could be important in controlling serpentine vegetation ecophysiology, but its toxicity can be ameliorated by P (Zemunik et al. Citation2020); the role that Mn might play in influencing the ‘serpentine syndrome’ is therefore worthy of further study.
In conclusion, whilst some of the properties of serpentine ecosystems, such as elevated metal concentrations, were present at this Scottish woodland site, only a few foliar elements (Ni and Mn) were influenced by the soil metal concentrations. Development of an oak woodland over such soils is likely due to the serpentine outcrop being in a mixed geological setting that has ameliorated more extreme soil chemistry (in terms of high Ca-to-Mg ratio, relatively low metal concentrations and high soil P) and prevented attributes of the more barren serpentine sites found elsewhere being expressed.
InchData.xlsx
Download MS Excel (25.2 KB)Acknowledgements
Thanks to the Glasgow Natural History Society for funding this project through the Blodwen Lloyd Binns Bequest Fund; Sarah Eaton of Scottish Natural Heritage for facilitating access permission; Dulce Flores-Rentería, Liam Trethowan, David McKendry, Daniel Tanner and John Stainton for laboratory assistance; and Elias Symeonakis and Giacomo Sellan for help with the figures and analysis. The reviewers are particularly thanked for their comments that markedly improved the manuscript.
Disclosure statement
No potential conflict of interest was reported by the author.
Notes
1 These two terms have been used somewhat interchangeably in ecological literature; here I use ‘serpentine’ when referring to plants/soils and ‘ultramafic’ when referring to rocks.
References
- Alexander EB. 1988. Morphology, fertility and classification of productive soils on serpentinized peridotite in California (U.S.A.). Geoderma. 41(3-4):337–351.
- Barrutia O, Artetxe U, Hernández A, Olano JM, García-Plazaola JI, Garbisu C, Becerril JM. 2011. Native plant communities in an abandoned Pb-Zn mining area of northern Spain: implications for phytoremediation and germplasm preservation. Int J Phytorem. 13(3):256–270.
- Bluck BJ. 2015. The lower old red sandstone at Balmaha—Aberfoyle and its bearing on the nature of the Highland Boundary and Gualann Faults. Scott J Geol. 51(2):165–176.
- Brearley FQ. 2018. Geo-ecological studies on two ultramafic sites in western Ireland. Ecol Res. 33(3):581–591.
- Brearley FQ, Jeffrey DW, Reeves RD. 2020. Minor influence of ultramafic (serpentine) geology on plant foliar chemistry at Errisbeg, Connemara, Ireland. Irish Natural J. 37(1):1–4.
- Brooks RR. 1987. Serpentine and its vegetation: a multidisciplinary approach. Portland (OR): Dioscorides Press.
- Chiarucci A, Robinson BH, Bonini I, Petit D, Brooks RR, De Dominicis V. 1998. Vegetation of Tuscan ultramafic soils in relation to edaphic and physical factors. Folia Geobot. 33(2):113–131.
- Davey MP, Berg B, Emmett BA, Rowland P. 2007. Decomposition of oak leaf litter is related to initial litter Mn concentrations. Can J Bot. 85(1):16–24.
- D’Amico ME, Previtali F. 2012. Edaphic influences of ophiolitic substrates on vegetation in the western Italian Alps. Plant Soil. 351(1-2):73–95.
- Goodenough KM, Finlayson A, Barron HF. 2008. Geodiversity of the Loch Lomond and The Trossachs National Park: statement of significance and identification of opportunities. Keyworth, Nottingham (UK): British Geological Survey.
- Hall JE, Kirby KJ, Whitbread AM. 2004. National vegetation classification: field guide to woodland. Peterborough, (UK): Joint Nature Conservation Committee.
- Hanikenne M, Nouet C. 2011. Metal hyperaccumulation and hypertolerance: a model for plant evolutionary genomics. Curr Opin Plant Biol. 14(3):252–259.
- Harrison S, Rajakaruna N, editors. 2011. Serpentine: the evolution and ecology of a model system. Berkeley (CA): University of California Press.
- Hornung M, Mew G. 1970. Report on the soils of the Island of Inchcailloch. Internal report to Nature Conservancy Council. Bangor, UK: Loch Lomond National Nature Reserve.
- Horrill AD, Sykes JM, Idle ET. 1975. The woodland vegetation of Inchcailloch, Loch Lomond. Trans Botan Soc Edinb. 42(3):307–334.
- Johnston WR, Proctor J. 1979. Ecological studies on the Lime Hill serpentine, Scotland. Trans Botan Soc Edinb. 43(2):145–150.
- Kanellopoulos C, Argyraki A, Mitropoulos P. 2015. Geochemistry of serpentine agricultural soil and associated groundwater chemistry and vegetation in the area of Atalanti, Greece. J Geochem Explor. 158:22–33.
- Kazakou E, Dimitrakopoulos PG, Baker AJM, Reeves RD, Troumbis AY. 2008. Hypotheses, mechanisms and trade-offs of tolerance and adaptation to serpentine soils: from species to ecosystem level. Biol Rev Camb Philos Soc. 83(4):495–508.
- Konečná V, Yant L, Kolář F. 2020. The evolutionary genomics of serpentine adaptation. Front Plant Sci. 11:574616.
- Lambers H, Hayes PE, Laliberté E, Oliveira RS, Turner BL. 2015. Leaf manganese accumulation and phosphorus-acquisition efficiency. Trends Plant Sci. 20(2):83–90.
- Lazarus BE, Richards JH, Claassen VP, O’Dell RE, Ferrell MA. 2011. Species specific plant-soil interactions influence plant distribution on serpentine soils. Plant Soil. 342(1-2):327–344.
- Mayer AM, Gorham E. 1951. The iron and manganese content of plants present in the natural vegetation of the English Lake District. Ann Bot. 15(2):247–263.
- McGahan DG, Southard RJ, Claassen VP. 2008. Tectonic inclusions in serpentinite landscapes contribute to plant nutrient calcium. Soil Sci Soc Am J. 72(3):838–847.
- McGahan DG, Southard RJ, Claassen VP. 2009. Plant-available calcium varies widely in soils on serpentinite landscapes. Soil Sci Soc Am J. 73(6):2087–2095.
- Oliva SR, Valdés B. 2004. Influence of washing on metal concentrations in leaf tissue. Commun Soil Sci Plant Anal. 35(11-12):1543–1552.
- Proctor J. 1971. The plant ecology of serpentine: II. Plant response to serpentine soils. J Ecol. 59(2):397–410.
- Proctor J. 1992. Chemical and ecological studies on the vegetation of ultramafic sites in Britain. In: Roberts BA and Proctor J, editors. The ecology of areas with serpentinized rocks: a world view. Dordrecht, The Netherlands: Kluwer Academic Publishers; p. 135–167.
- Proctor J, Woodell SRJ. 1971. The plant ecology of serpentine: I. Serpentine vegetation of England and Scotland. J Ecol. 59(2):375–395.
- Proctor J, Woodell SRJ. 1975. The ecology of serpentine soils. Adv Ecol Res. 9:255–366.
- Proctor J, Bartlem K, Carter SP, Dare DA, Jarvis SB, Slingsby DR. 1991. Vegetation and soils of the Meikle Kilrannoch ultramafic sites. Botan J Scotl. 46(1):47–64.
- Pugnaire FI. 2001. Variability of inorganic nutrient concentrations in leaves. New Phytol. 150(3):506–507.
- Reeves RD, Kruckeberg AR. 2018. Re-examination of the elemental composition of some Caryophyllaceae on North American ultramafic soils. Ecol Res. 33(4):715–722.
- Roberts BA, Proctor J. editors 1992. The ecology of areas with serpentinized rocks: a world view. Dordrecht, The Netherlands: Kluwer Academic Publishers.
- Serrano HC, Cotrim H, Pinto MJ, Martins-Loução MA, Branquinho C. 2017. Metal hyperaccumulation patterns with Plantago phylogeny (Plantaginaceae). Plant Soil. 411(1-2):227–241.
- Teptina A, Paukov A, Rajakaruna N. 2018. Ultramafic vegetation and soils in the circumboreal region of the northern hemisphere. Ecol Res. 33(3):609–628.
- Tittensor RM. 1970. History of the Loch Lomond Oakwoods. 2. Period of intensive management: 1700-1920. Scott Forest. 24(2):110–118.
- Tittensor RM, Steele RC. 1971. Plant communities of the Loch Lomond Oakwoods. J Ecol. 59(2):561–582.
- Tsiripidis I, Papaioannou A, Sapounidis V, Bergmeier E. 2010. Approaching the serpentine factor at a local scale–a study in an ultramafic area in northern Greece. Plant Soil. 329(1-2):35–50.
- Tyndall RW. 2012. Soil differences between extant serpentine oak savanna and grassland in Soldiers Delight Natural Environment Area, Maryland. Castanea. 77(3):224–230.
- Williams PC. 1967. Nickel, iron, and manganese in the metabolism of the oat plant. Nature. 214(5088):628.
- Zemunik G, Winter K, Turner BL. 2020. Toxic effects of soil manganese on tropical trees. Plant Soil. 453(1-2):343–354.