Abstract
The goal of this study was to tackle two questions related to the systematics of small-flowering Euphrasia species from the Eastern Alps. Our first aim was to test the hypothesis of a wider distribution of diploid E. inopinata, considered endemic to the uppermost Ötztal valley in Austria based on ploidy screening of ca. 70 populations along a latitudinal transect. We failed to find new populations of E. inopinata but during the sampling campaign, a diploid population was detected in the eastern Ortler/Ortles mountain range (Italy), which could neither be assigned to E. inopinata nor to E. minima. Thus, our second aim was to clarify, based on a comprehensive sampling of individuals and the use of Amplified Fragment Length Polymorphisms (AFLPs) and/or morphometric data, if this diploid population is morphologically and genetically consistently divergent from both, tetraploid widespread E. minima and E. inopinata. As this was confirmed, we established the relationships of the new entity based on Internal Transcribed Spacer sequences, which uncovered a close relationship with E. minima and describe it here as a new diploid species, E. ultima, which, based on current knowledge, is locally endemic even if a bigger distribution area appears likely.
Keywords:
Introduction
The genus Euphrasia L. comprises 100 to 350 hemiparasitic annual or perennial species (Fischer et al., Citation2008; Svobodova et al., Citation2016) distributed in temperate zones of both hemispheres as well as in tropical mountain areas. Whereas in the Southern Hemisphere the genus exhibits much variation in growth form, life cycle and flower colour, many Northern Hemisphere species are morphologically similar and often taxonomically intricate (Yeo, Citation1978; Vitek, Citation1998; Gussarova et al., Citation2008; Svobodova et al., Citation2016). All European species except one species from the Azores are annual. Based on x = 11 chromosomes, they exhibit two ploidy levels, diploid (2n = 2x = 22) and tetraploid (2n = 4x = 44), tetraploid species being the main source of taxonomic uncertainties (Vitek, Citation1986; Svobodova et al., Citation2016). Morphological variability is affected by the impact of host plants and environmental factors (Brown et al., Citation2019), interspecific hybridization, which is especially prevalent in tetraploids, seasonal dimorphism and phenotypic plasticity (Vitek Citation1998). Divergent morphotypes may stabilize because of frequent self-pollination (Yeo, Citation1978; Liebst and Schneller, Citation2005; French et al., Citation2008; Svobodova et al., Citation2016).
The widely distributed E. minima Jacq. ex DC. is a small-flowered tetraploid distributed in temperate and boreal alpine grasslands across the central and southern European mountain ranges (Hultén and Fries Citation1986). In Europe, a major portion of the distribution range is found in the Alps, where this species exhibits pronounced morphological variation (Vitek, Citation1986). Two diploid species endemic to the Austrian Alps were assumed to be closely related to E. minima. These are E. inopinata Ehrend. & Vitek, only known from the uppermost Ötztal valley (Obergurgl and Vent, Tyrol) and a probably introduced population in the Hohe Tauern of Salzburg (Vitek, Citation2000), and E. sinuata Ehrend. & Vitek occurring in the Rofan and Kitzbühler Alpen ranges in Tyrol, Austria (Greilhuber et al., Citation1984; Vitek, Citation1986). Genetic data (nuclear ribosomal Internal Transcribed Spacer, ITS, sequences and Amplified Fragment Length Polymorphism, AFLP), however, indicate that these two species are more closely related to E. alpina Lam. from E. ser. Alpinae than to E. minima, which belongs to ser. Parviflorae (Pan et al., Citation2019).
The restriction of E. inopinata to a small part of Ötztaler Alpen close to the main chain of the Alps is surprising from a biogeographic point of view. This mountain range was among the most strongly glaciated ones in the Eastern Alps at the Last Glacial Maximum (van Husen, Citation1987) and is thus far outside the previously recognised centres of endemism (Tribsch, Citation2004). As the morphological differences between E. inopinata and E. minima are intricate, we expected that E. inopinata has been overlooked and in fact has a wider distribution extending to the glacial refugia at the southern periphery of the Alps (Schönswetter et al., Citation2005). Thus, our first aim was to test the hypothesis of a wider distribution based on ploidy screening and morphometric analysis of a comprehensive sampling of small-flowered Euphrasia populations inhabiting silicicolous alpine vegetation along a latitudinal transect spanning from the occurrences of E. inopinata in the Ötztaler Alpen southwards towards the margin of the Alps.
During the sampling campaign, a diploid population was detected in the uppermost Ultental/Val d’Ultimo valley in the eastern Ortler/Ortles mountain range (Südtirol/Alto Adige, Italy), which could neither be assigned to E. inopinata nor to E. minima. Thus, our second aim was to clarify, based on a comprehensive sampling of individuals and the use of Amplified Fragment Length Polymorphisms (AFLPs) and/or morphometric data, if this diploid population (termed E. “Ultental” in the following) is morphologically and genetically consistently distinct from both, E. minima and E. inopinata. As such a distinction was confirmed, we established the relationships of the new entity by adding it to the phylogenetic framework based on Internal Transcribed Spacer (ITS) sequences established by Pan et al. (Citation2019) and describe it here as a new diploid species, which, based on current knowledge, is locally endemic even if a bigger distribution area appears likely.
Material and methods
Sampling
As the morphological discrimination between E. minima and E. inopinata is intricate, our strategy was as follows. During the first field season in 2017 usually twenty individuals were sampled (ten individuals in both, alcohol and silica gel for morphologic and genetic analysis, ten individuals as herbarium material) in each of 69 uniformly white- and small-flowered alpine populations (; ). We only sampled white-flowering populations as yellow-flowered individuals, alone or together with white-flowered ones, are among the focal species only known from E. minima. Populations were situated between 1697 m and 2877 m a.s.l. along a transect spanning from Obergurgl (Ötztaler Alps, Austria; i.e. the type locality of E. inopinata) in the north to Passo del Maniva (Prealpi Bresciane or Adamello-Presanella, Italy) in the south (“large dataset”; ). Samples of E. inopinata (used in Pan et al, Citation2019) were contributed by D. Pan (University of Vienna).
Figure 1. Sampled populations of Euphrasia minima (numbered), E. alpina, E. inopinata and E. “Ultental” (details are given in ). The broken line indicates the maximal extent of the ice shield during the Last Glacial Maximum (taken from van Husen Citation1987).
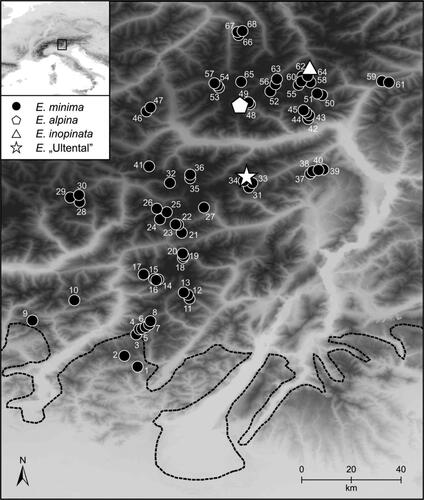
Table 1. Studied Euphrasia populations. NFCM and NMORPH, number of individuals investigated with flow cytometry and morphometry, respectively.
During the second field season in 2019 we focussed on the vicinity of the occurrence of E. “Ultental” (“small dataset”). There, in total 70 individuals of E. minima and E. “Ultental” were collected. In addition, 20 individuals of E. alpina were sampled ca. 30 km further north (). From each individual one leaf each of two to four leaf pairs and, if available, flowers were immediately stored in silica gel for flow cytometry and genetic analysis, while the rest of the plant was stored in 70% ethanol for morphometric analysis. Herbarium vouchers are stored in the herbarium of the University of Innsbruck (IB). Finally, in August 2020 young flowering buds of E. “Ultental” were fixed in a freshly prepared 3:1 mix of EtOH and glacial acetic acid for chromosome counting.
Flow cytometry
For ploidy level determination relative genome size (RGS) was measured using flow cytometry (FCM) of 4′,6-diamidino-2-phenylindole (DAPI) stained nuclei. Bellis perennis L. (2 C = 3.38 pg; Schönswetter et al., Citation2007) was used as reference standard to measure the relative fluorescence intensity of 3000 particles using a CyFlow Space flow cytometer (Partec, Münster, Germany). Measurements were done using one silica gel dried flower and the appropriate amount of fresh leaf material of reference standard, and processed as described in Suda and Trávníček (Citation2006). Histograms were evaluated using FloMax software (Partec) and the reliability of the measurements was assessed by calculating coefficients of variation (CVs) for the G1 peaks of both the analysed sample and the reference standard. The CV was below 5% for all samples. For the large dataset five individuals per population were measured; if a diploid individual was detected, all ten collected individuals of the population were measured, totalling 390 measured individuals. For the small data set all individuals were measured.
Chromosome number analysis
For chromosome number analysis flower buds of several individuals were fixed in ethanol: glacial acetic acid (3:1) overnight and stored at −20 °C until use. The material was hydrolyzed in 5 N HCl for 20 min, washed with water, and stained with Schiff’s reagent (Merck, Darmstadt, Germany) for 1 hr at room temperature in darkness. The tissue was squashed in a drop of 60% acetic acid under a cover slip and the preparations were analysed using an AxioPlan fluorescence microscope (Carl Zeiss, Vienna, Austria) and 43 HE filter (excitation BP 550/25). Images were captured using a CCD black-white camera and Axiovision 4.8 software (Carl Zeiss, Vienna, Austria).
DNA isolation, ITS sequencing and AFLP fingerprinting
Total genomic DNA was extracted from all individuals of the small dataset. DNA was extracted from silica dried leaves following a CTAB protocol (Doyle and Doyle, Citation1987) with modifications detailed in Schönswetter et al. (Citation2002). Prior to extraction with a high-salt CTAB buffer the ground tissue was washed twice with a wash buffer containing sorbitol to remove polysaccharides (Tel-Zur et al., Citation1999).
ITS was sequenced for four individuals of E. “Ultental” using the primers ITS4 and ITS5 from White et al. (Citation1990) for amplification and a protocol modified from Pan et al. (Citation2019). The PCR reaction mix (10 μL in total) contained 5 μL ReddyMix PCR Master Mix (Thermo Fisher Scientific, Vienna, Austria), 2 μL trehalose dihydrate (1 M; Sigma, Vienna, Austria), 2 μL bovine serum albumin (BSA) (1 mg mL−1, Thermo Fisher Scientific), 0.3 μL dimethyl sulfoxide (DMSO) (100%, Thermo Fisher Scientific), 1 μL of each primer (5 μM μL−1) and 1 μL of genomic DNA with unknown concentration. The PCR condition was: denaturation for 2 min at 95 °C; 35 cycles each of 25 s at 95 °C, 35 s at 50 °C, 1 min at 72 °C; 5 min at 72 °C. PCR products were checked on a 1.5% agarose gel. PCR products were purified using the mixture EXO/FastAP (Exonuclease I and Thermosensitive Alkaline Phosphatase, Thermo Fisher Scientific) following the manufacturer’s instructions. Sequencing was carried out at Eurofins Genomics (Ebersberg, Germany).
AFLP profiles were generated following established protocols (Vos et al. Citation1995) with the modifications either described in Schönswetter et al. (Citation2009) or detailed below. One blank (DNA replaced by water) was included to test for systematic contamination, and reproducibility was tested by replicating 25 individuals (Bonin et al., Citation2004). We used the same selective primer combination as Pan et al. (Citation2019), i.e. EcoRI (6-FAM)ATG/MseI-CTT, EcoRI (VIC)ACG/MseI-CAA and EcoRI (NED)-AGC/MseI-CTG (6-FAM-labelled primer: Sigma-Aldrich, St. Louis, Missouri, U.S.A.; NED- and VIC-labelled primers: Applied Biosystems, Foster City, California, U.S.A.). Subsequently, 3.3 μL of each selective PCR product was purified using a Sephadex G-50 Fine (GE Healthcare Bio-Sciences, Uppsala, Sweden) suspension applied to a MultiScreen-HV plate (Millipore, Molsheim, France) in three steps of 200 μL each and packed at 750 g for 1, 1 and 5 min, respectively, and 10 μL water for prewetting the Sephadex columns. Then 0.6 μL of the elution product was mixed with 10 μL formamide (Applied Biosystems) and 0.1 μL GeneScan 500 ROX (Applied Biosystems) and run on an Applied Biosystems 3130xl Genetic Analyzer at the Department of Botany and Biodiversity Research of the University of Vienna (Austria).
Analysis of ITS sequences and AFLP data
Contigs were assembled and edited using Geneious Pro v.5.5.9 (Kearse et al., Citation2012). The sequences were added and manually aligned to the data set of Pan et al. (Citation2019). A maximum likelihood (ML) analysis was done using RAxML 8.2.3 (Stamatakis Citation2014) employing the same settings as Pan et al. (Citation2019).
Raw AFLP data were aligned with the internal size standard using ABI Prism GeneScan 3.7.1 (Applied Biosystems) and imported into Genographer 1.6.0 (version no longer available) for scoring. The error rate (Bonin et al., Citation2004) was calculated based on 23 replicated individuals as the ratio of mismatches (scoring of 0 vs. 1) over matches (1 vs. 1) in AFLP profiles of replicated individuals. Fragments with single presences or absences were excluded. A neighbour-joining (NJ) analysis based on Nei-Li genetic distances (Nei and Li, Citation1979) was conducted and bootstrapped (2000 pseudo-replicates) with TREECON v.1.3b (Van de Peer and De Wachter, Citation1997). The tree was rooted with E. alpina. Due to the clear phylogenetic structure no further analyses were conducted.
Morphometry
Plants were photographed with an Olympus UC30 camera mounted on an Olympus SZX9 stereomicroscope using the Olympus image analysis software analySIS pro. For the large dataset, the single available individual of E. inopinata, three individuals of E. “Ultental” and 69 individuals of E. minima from 25 populations were measured (). Five characters were used directly, including three leaf characters measured on a lowermost bract, i.e. “number of teeth per side of the leaf” (NT), “mean angle of the tips of both subterminal teeth” [°] (AT), and “angle of the leaf basis” [°] (AB), plus two floral characters, which are “flower length” [mm] (FL) and “depth of the incision in the middle part of the lower lip” [mm] (ID). Further, three ratios, i.e. “flower length”/”leaf length” (FL/L), “length of the lower lip”/”width of the broadest part of the lower lip” (LL/WB), and “leaf length”/”number of teeth per side of the leaf” (L/NT) were included, resulting in eight characters ().
Figure 2. Morphological characters scored for Euphrasia “Ultental” and E. minima. Abbreviations are as in .
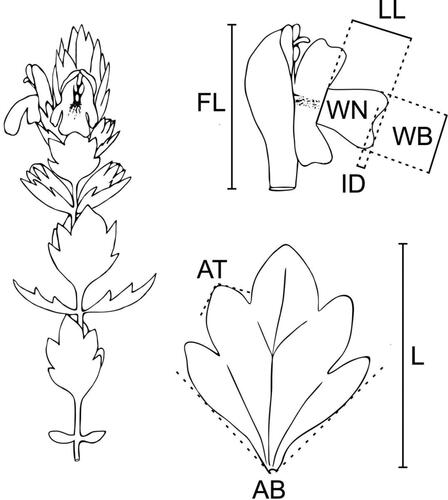
In the small dataset the morphologically clearly divergent, large-flowered E. alpina was excluded and the character “width of the narrowest part of the lower lip” [mm] (WN) was additionally measured to better capture the differentiation between co-occurring E. minima (24 individuals) and E. “Ultental” (55 individuals) and used in the ratio “length of the lower lip”/”width of the narrowest part of the lower lip” (LL/WN) resulting in a total of nine characters (, ). The samples were complemented with three and six individuals of E. “Ultental” and E. minima, respectively, which were also used in the large dataset.
Table 2. Morphological differentiation between Euphrasia “Ultental” and E. minima from Ultental/Val d’Ultimo (Südtirol/Alto Adige, Italy) based on nine morphometric characters (units are in squared parentheses, abbreviations of characters are in parentheses, the most strongly differentiating characters are in bold).
Non-parametric Spearman rank coefficients were computed to reveal the correlation structure among the characters and to ensure that no strong correlations (> 0.95) between characters were present. After standardization to zero mean and unit variance, a principal component analysis (zero-centred PCA based on a covariance matrix) was applied to display the overall variation pattern along the first two components for both datasets. To express the extent of morphological differentiation between the two ploidy-defined groups, a linear discriminant analysis (LDA) and a classificatory discriminant analysis (CDA) were performed for the small dataset. Analyses were conducted using MorphoTools (Koutecký, Citation2015) in R 3.6.3 (R Core Team, Citation2020).
Results
In the large dataset, the mean ratio between sample and standard was 0.64 ± 0.02 for tetraploid E. minima (). The three individuals of E. “Ultental” had an RGS of 0.31 ± 0.01. In the small dataset, the 20 individuals of E. alpina were diploid (0.36 ± 0.01). Among the 70 individuals from Ultental, 53 were diploid (0.31 ± 0.01) and thus represent E. “Ultental,” and 17 individuals were tetraploid (0.62 ± 0.01) and, consequently, are E. minima.
Analyses of 12 flower buds contained already fully developed anthers with pollen grains, thus the chromosome number was determined in somatic cells of the flower buds. All analyzed cells possessed 2n = 22 small chromosomes ().
One polymorphism was detected in the ITS sequences (Genbank accession numbers MW561628–MW561631) retrieved from four individuals of E. “Ultental”; specifically, the sequence of one individual (MW561630) differed in having a C instead of a clear C/G polymorphism with two equally high peaks in both forward and reverse sequences at position 467. The other three sequences of E. “Ultental” were identical to those of E. minima presented by Pan et al. (Citation2019), with which they formed a maximally supported clade (bootstrap, BS, 100; ). Unsurprisingly, the other relationships were qualitatively identical to those in Pan et al. (Citation2019).
Figure 4. Phylogenetic placement of Euphrasia “Ultental” based on nuclear ITS sequences analysed using maximum likelihood (numbers at nodes are bootstrap support values of at least 50). Newly obtained sequences are in bold.
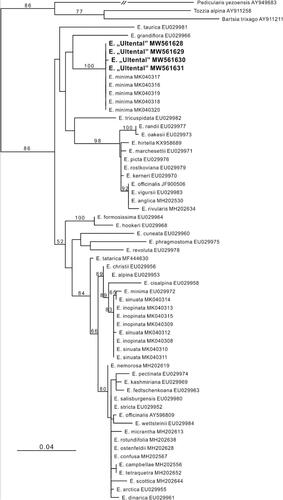
A few individuals failed to produce high-quality AFLP fingerprints and were excluded. The error rate based on 23 sample-replicate comparisons was 0.4%. The length of the fragments varied between 84 and 494 bp. The final matrix comprised 64 individuals (20 of E. alpina, 17 of E. minima and 27 of E. “Ultental”) and 118 AFLP fragments (five fragments present in only a single individual were excluded). The NJ tree rooted with E. alpina resolved all three species as monophyletic groups with maximal BS support ().
Figure 5. Neighbour joining tree based on Nei-Li distances among AFLP fingerprints of E. “Ultental” and co-occurring E. minima. The tree was rooted with E. alpina. Numbers are bootstrap values, which are given only for major branches.
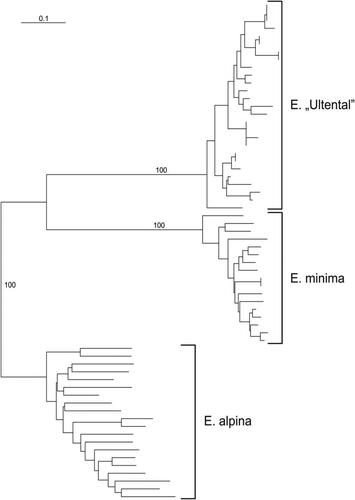
In both morphometric datasets all characters were retained as no correlations > 0.95 were observed. For the large dataset, the PCA () showed a clear differentiation between the diploid entities, but pronounced variation within E. minima. For the small dataset, we found pronounced differentiation between E. “Ultental” and E. minima in several characters (). The PCA ordination diagram () showed a clear separation of E. “Ultental” and E. minima along the first axis. “Depth of the incision in the middle part of the lower lip” contributed most strongly to the observed differentiation, followed by the ratio “length of the lower lip/width of the narrowest part of the lower lip” (). The LDA supported the results obtained by the PCA, separating the two entities without overlap (). Likewise, “depth of the incision in the middle part of the lower lip” contributed most strongly. A CDA classified 100% of E. “Ultental” and 87.5% of E. minima correctly, i.e. from the 79 individuals included in the analysis only three individuals of E. minima were incorrectly classified.
Figure 6. Principal component analysis of Euphrasia minima (white dots), E. “Ultental” (stars) and E. inopinata (black dot) illustrating the morphological differentiation between the two last-mentioned diploid, albeit distantly related (), entities (large dataset). Arrows in the inset represent the contribution of the characters to the overall explained variation. The arrows start at 0/0 and have been shifted to the upper right corner to ease legibility of the figure. Abbreviations of morphometric characters correspond to .
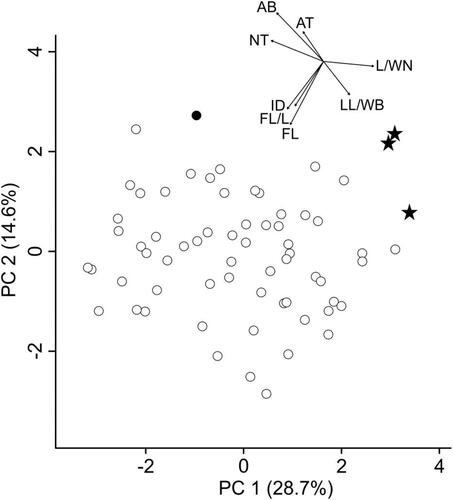
Figure 7. Morphological differentiation between Euphrasia “Ultental” and E. minima from Ultental/Val d’Ultimo (Südtirol/Alto Adige, Italy; small dataset). Boxes show median, upper and lower quartiles, whiskers show 1.5x interquartile range, all values outside this range are shown as dots (outliers).
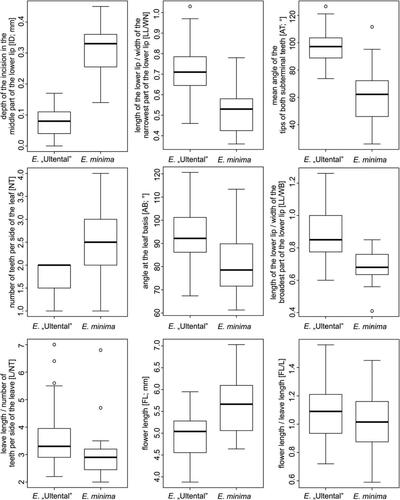
Figure 8. Morphological differentiation between Euphrasia “Ultental” (black) and E. minima (white) from Ultental/Val d’Ultimo (Südtirol/Alto Adige, Italy; small dataset). (A) Principal component analysis; arrows in the insets represent the contribution of the characters to the overall explained variation. For details, see . (B) Histogram of a linear discriminant analysis, the contribution of the characters to the linear discriminant function is given in .
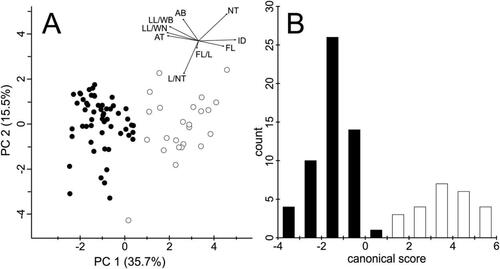
Discussion
Our first aim was to test the hypothesis that E. inopinata, considered to be locally endemic to the surroundings of the villages Obergurgl and Vent in the central Ötztaler Alpen (Tyrol, Austria), is in fact more widely distributed and as such may also occur in well-known peripheral refugial areas on the southern margin of the Alps (Tribsch and Schönswetter Citation2003; Tribsch Citation2004). We, therefore, sampled and cytotyped, using flow cytometry, 68 populations of small-flowered alpine Euphrasia arranged in a latitudinal transect spanning from the distribution area of E. inopinata north of the main chain of the Alps to their southern periphery (). Based on the available dataset, which obviously does not include all occurrences of small-flowered alpine Euphrasia in the sampling area, we failed to find populations of E. inopinata outside of its known distribution area (). As a wider distribution of E. inopinata overlapping also with glacial refugia at the southern margin of the Alps (Tribsch Citation2004) was rejected, one of the previously suggested scenarios appears to be more plausible; specifically, E. inopinata may have survived in situ as suggested in the species’ description (Ehrendorfer and Vitek Citation1984), or it may be of recent (postglacial) origin as suggested for Euphrasia species endemic to the British Isles (French et al. Citation2008; Pan et al. Citation2019).
Our second aim was to test, based on an array of complementary approaches including flow cytometry, AFLP fingerprinting, ITS sequencing and morphometry, if a newly detected, diploid () and morphologically aberrant Euphrasia population from Ultental/Val d’Ultimo in Südtirol/Alto Adige, Italy (E. “Ultental”) in fact represents a new species. As a first step, we established the phylogenetic relationships of E. “Ultental” against the background of a recently published ITS phylogeny (Pan et al. Citation2019); plastid data were previously shown to only weakly reflect evolutionary relationships likely due to the recency of the group’s radiation and the frequency of interspecific hybridisation (Gussarova et al. Citation2008). The ITS sequences uncovered a very tight relationship of E. “Ultental” with tetraploid E. minima (); in fact, sequences were either identical or differed only in a single, ambiguous base position. This finding is relevant as previous attempts to identify diploid progenitors of E. minima have failed (the hypothesised ancestors E. inopinata and E. sinuata are rather derived from E. alpina; Pan et al. Citation2019). If E. “Ultental” is indeed a diploid ancestor of E. minima, this might be another example where a polyploid derivative occupies a much larger range than its diploid ancestor (Kuzmanović et al. Citation2017; Winkler et al. Citation2017).
Once the close relationship of E. “Ultental” and E. minima was established, we were interested in the depth of genetic divergence between the two entities; we tackled this issue using highly resolving AFLP fingerprinting, a frequently employed approach to disentangle complexes of both allopolyploid and autopolyploid origin (e.g. Dixon et al. Citation2009, Winkler et al. Citation2017). The AFLPs uncovered a deep split between E. “Ultental” and syntopic E. minima without showing any signs of introgression (). The obvious strength of the crossing barrier is not unexpected given the prevalence of autogamy in small-flowered Euphrasia species (French et al. Citation2005) and the difference in ploidy level, although gene flow from diploids into tetraploids via unreduced gametes (Kolář et al. Citation2017) may be possible.
Finally, we focussed on the morphological differences between E. “Ultental” and closely related or superficially similar species. Specifically, we could show, using a broad sampling (25 populations, large dataset) of E. minima, that both E. inopinata and E. “Ultental” fall outside the pronounced variation seen in E. minima (). In this particular analysis it was also evident that E. “Ultental” and E. inopinata are morphologically dissimilar, reflecting their distant relationship unravelled by the ITS sequences (). When focussing on the local scale, that is, on the few hundred square meters representing the currently known distribution of E. “Ultental” (small dataset; ; and ), clear morphological differences differentiate this entity and co-occurring E. minima. The most strongly discriminating character is the depth of the incision in the middle part of the lower lip, which is absent to very shallow in E. “Ultental,” and the wide angle of the subterminal teeth on the leaf margin (), but also other characters have some discriminative power leading to a complete lack of overlap of both entities in PCA () and LDA ().
Altogether, we are convinced that – based on the depth of genetic and phenotypic divergence and the lack of intermediates – E. “Ultental” is sufficiently differentiated on the various levels of variation tackled by our analyses to render taxonomic recognition on the species level warranted ( and ). This, in line with other recent findings (Köckinger Citation2003; Flatscher et al. Citation2015), clearly illustrates that we are still far from a comprehensive knowledge of biodiversity, even in exceptionally well-investigated areas such as the European Alps.
Taxonomic treatment
Euphrasia ultima J. Hartmann & Schönsw. sp. nov.
Type: Italy, Südtirol/Alto Adige, Ortler-Gruppe/Gruppo Ortles-Cevedale, Ultental/Val d’Ultimo: close to the dam of the accumulation lake Grünsee, 2540 m, 10.08.2019, P. Schönswetter & J. Hartmann (holotype IB No. 110312!; isotypes W and BOZ).
Description: Plant (2.6–)3.0–5.5(–6.7) cm tall, upper leaves densely imbricate, stem and leaves glabrous or hairy, but always eglandular. Leaves slightly succulent, (3.3–)3.7–6.5(–7.6) mm wide and (4.4–)5.1–7(–7.9) mm long, angle at leaf basis (67–)83–109(–121)°, leaf margin strongly revolute, up to two teeth per side, their tips (73–)82–113(–126)°. Corolla (3.88–)4.16–5.51(–5.95) mm long, middle lobe of the lower lip almost rectangular with a ratio between the broadest and the narrowest part of (0.6–)0.7–0.9(–1.0), with no or only a shallow incision of (0–)0.02–0.14(–0.17) mm depth. 2n = 22 ().
Diagnosis: Diploid E. ultima differs from the closely related widespread tetraploid E. minima, with which it co-occurs, and the more distantly related, but morphologically superficially similar diploid E. inopinata, which is locally endemic to the upper-most Ötztal, Austria, in the incision in the middle part of the lower lip of their flowers, which is lacking or shallow and the low number (0–2 per each side) of teeth of the bracts. In addition, it differs from E. minima by the densely imbricate leaves at the shoot apex and from E. inopinata by having less acute tips of the teeth at the leaf margin and by the branched stem of vigorous individuals.
Ecology: The locus classicus is situated at ca. 2,500 m a.s.l. on siliceous bedrock with a high iron content, the surrounding vegetation is dominated by Silene acaulis subsp. bryoides (Jord.) Nyman, Agrostis alpina Scop. and Festuca halleri All. Further accompanying species are Comastoma tenellum (Rottb.) Toyok., Erigeron uniflorus L., Euphrasia minima, Gentiana nivalis L., Hieracium alpinum L. s.l., Leucanthemopsis alpina (L.) Heywood s.l., Minuartia recurva (All.) Schinz & Thell., Pedicularis kerneri Dalla Torre, Phyteuma hemisphaericum L., Poa alpina L., Saxifraga bryoides L., Senecio insubricus (Chenevard) R. Flatscher, Schneew. and Schönsw., Sibbaldia procumbens L., and Solidago virgaurea L. subsp. minuta (L.) Arcang. ().
Distribution: The species is only known from the type locality but a wider distribution appears likely.
Conservation status: As the distribution is most likely insufficiently known, we suggest classifying E. ultima as data deficient (DD) according to the IUCN (2012) criteria.
Etymology: The species is named in reference to the Italian name of its only known occurrence in uppermost Ultental/Val d’Ultimo.
Acknowledgements
Much of the data presented formed a part of Julia Hartmann’s MSc thesis conducted at the Department of Botany of the University of Innsbruck. A collecting permit for the Nationalpark Stilfserjoch/Parco Nazionale dello Stelvio was issued by the Autonome Provinz Bozen – Südtirol/Provincia Autonoma Bolzano – Alto Adige on August 20th, 2019.
Disclosure statement
The authors declare that they have no conflict of interests.
Additional information
Funding
References
- Bonin A, Bellemain E, Eidesen PB, Pompanon F, Brochmann C, Taberlet P. 2004. How to track and assess genotyping errors in population genetics studies. Mol Ecol. 13(11):3261–3273.
- Brown M, Frachon N, Wong ELY, Metherell C, Twyford A. 2019. Life history evolution, species differences and phenotypic plasticity in hemiparasitic eyebrights (Euphrasia). Am J Bot. 107(3):1–10.
- Dixon CJ, Schönswetter P, Suda J, Wiedermann M, Schneeweiss GM. 2009. Reciprocal Pleistocene origin and postglacial range formation of an allopolyploid and its sympatric ancestors (Androsace adfinis group, Primulaceae). Mol Phylogenet Evol. 50(1):74–83.
- Doyle JJ, Doyle JL. 1987. A rapid DNA isolation procedure for small amounts of fresh leaf tissue. Phytochem Bull. 19(1):11–15.
- Ehrendorfer F, Vitek E. 1984. Evolution alpiner Populationen von Euphrasia (Scrophulariaceae): Entdeckung kleinblütiger diploider Sippen. Pl Syst Evol. 144(1):25–44.
- Fischer MA, Oswald K, Adler W. 2008. Exkursionsflora für Österreich, Liechtenstein und Südtirol. 2nd ed. Linz: Biologiezentrum der Oberösterreichischen Landesmuseen.
- Flatscher R, Escobar García P, Hülber K, Sonnleitner M, Winkler M, Saukel J, Schneeweiss GM, Schönswetter P. 2015. Underestimated diversity in one of the world’s best studied mountain ranges: The polyploid complex of Senecio carniolicus (Asteraceae) contains four species in the European Alps. Phytotaxa. 213(1):1–21.
- French GC, Ennos RA, Silverside AJ, Hollingsworth PM. 2005. The relationship between flower size, inbreeding coefficient and inferred selfing rate in British Euphrasia species. Heredity (Edinb). 94(1):44–51.
- French GC, Hollingsworth PM, Silverside AJ, Ennos RA. 2008. Genetics, taxonomy and the conservation of British Euphrasia. Conserv Genet. 9(6):1547–1562.
- Greilhuber J, Vitek E, Ehrendorfer F. 1984. Evolution alpiner Populationen von Euphrasia (Scrophulariaceae): Chromosomenzählungen an diploiden und polyploiden Sippen aus den Ostalpen. Pl Syst Evol. 144(1):45–51.
- Gussarova G, Popp M, Vitek E, Brochmann C. 2008. Molecular phylogeny and biogeography of the bipolar Euphrasia (Orobanchaceae): Recent radiations in an old genus. Mol Phylogenet Evol. 48(2):444–460.
- Hultén E, Fries M. 1986. Atlas of North European vascular plants: north of the Tropic of Cancer I-III. Königstein (DE): Koeltz Botanical Books.
- IUCN. 2012. Species Survival Commission. IUCN Red List Categories and Criteria, version 3.1. 2nd ed. Gland (CH): IUCN.
- Kearse M, Moir R, Wilson A, Stones-Havas S, Cheung M, Sturrock S, Buxton S, Cooper A, Markowitz S, Duran C, et al. 2012. Geneious basic: An integrated and extendable desktop software platform for the organization and analysis of sequence data. Bioinformatics. 28(12):1647–1649.
- Köckinger H. 2003. Saxifraga styriaca spec. nova (Saxifragaceae) – ein Endemit der östlichen Niederen Tauern (Steiermark, Österreich). Phyton. 43(1):79–108.
- Kolář F, Čertner M, Suda J, Schönswetter P, Husband B. 2017. Mixed-ploidy species: progress and opportunities in polyploid research. Trends Plant Sci. 22(12):1041–1055.
- Koutecký P. 2015. MorphoTools: a set of R functions for morphometric analysis. Plant Syst Evol. 301(4):1115–1121.
- Kuzmanović N, Lakušić D, Frajman B, Alegro A, Schönswetter P. 2017. Phylogenetic relationships in Seslerieae (Poaceae) including resurrection of Psilathera and Sesleriella, two monotypic genera endemic to the Alps. Taxon. 66(6):1349–1370.
- Liebst B, Schneller J. 2005. How selfing and intra- and interspecific crossing influence seed set, morphology and ploidy level in Euphrasia: An experimental study of species occurring in the Alps of Switzerland. Plant Syst Evol. 255(3-4):193–214.
- Nei M, Li WH. 1979. Mathematical model for studying genetic variation in terms of restriction endonucleases. Proc Natl Acad Sci U S A. 76(10):5269–5273.
- Pan D, Schönswetter P, Moser T, Vitek E, Schneeweiss GM. 2019. Ancestral remnants or peripheral segregates? Phylogenetic relationships of two narrowly endemic Euphrasia species (Orobanchaceae) from the eastern European Alps. AoB Plants. 11(2):plz007.
- R Core Team. 2020. R: A language and environment for statistical computing. Vienna, Austria: R Foundation for Statistical Computing.
- Schönswetter P, Stehlik I, Holderegger R, Tribsch A. 2005. Molecular evidence for glacial refugia of mountain plants in the European Alps. Mol Ecol. 14(11):3547–3555.
- Schönswetter P, Suda J, Popp M, Weiss-Schneeweiss H, Brochmann C. 2007. Circumpolar phylogeography of Juncus biglumis (Juncaceae) inferred from AFLP fingerprints, cpDNA sequences, nuclear DNA content and chromosome numbers. Mol Phylogenet Evol. 42(1):92–103.
- Schönswetter P, Tribsch A, Barfuss M, Niklfeld H. 2002. Several Pleistocene refugia detected in the high alpine plant Phyteuma globulariifolium Sternb. & Hoppe (Campanulaceae) in the European Alps. Mol Ecol. 11(12):2637–2647.
- Schönswetter P, Solstad H, Escobar Garcí P, Elven R. 2009. A combined molecular and morphological approach to the taxonomically intricate European mountain plant Papaver alpinum s.l. (Papaveraceae) — taxa or informal phylogeographical groups?Taxon. 58(4):1326–1348.
- Stamatakis A. 2014. RAxML version 8: a tool for phylogenetic analysis and post-analysis of large phylogenies. Bioinformatics. 30(9):1312–1313.
- Suda J, Trávníček P. 2006. Reliable DNA ploidy determination in dehydrated tissues of vascular plants by DAPI flow cytometry-new prospects for plant research. Cytometry A. 69(4):273–280.
- Svobodova Š, Košnar J, Koutecký P, Štech M. 2016. Microsatellite analysis of four similar Euphrasia (Orobanchaceae) species changes the traditional view of this group. Pl Ecol Evol. 149(1):45–58.
- Tel-Zur N, Abbo S, Myslabodski D, Mizrahi Y. 1999. Modified CTAB procedure for DNA isolation from epiphytic cacti of genera Hylocereus and Selenicereus (Cactaceae). Plant Mol Biol Rep. 17(3):249–254.
- Tribsch A. 2004. Areas of endemism of vascular plants in the Eastern Alps in relation to Pleistocene glaciation. J Biogeogr. 31(5):747–760.
- Tribsch A, Schönswetter P. 2003. Patterns of endemism and comparative phylogeography confirm palaeo-environmental evidence for Pleistocene refugia in the Eastern Alps. Taxon. 52(3):477–497.
- Van de Peer Y, De Wachter R. 1997. Construction of evolutionary distance trees with TREECON for Windows: accounting for variation in nucleotide substitution rate among sites. Comput Appl Biosci. 13(3):227–230.
- van Husen D. 1987. Die Ostalpen in den Eiszeiten. Vienna (A): Geologische Bundesanstalt.
- Vitek E. 1986. Evolution alpiner Populationen von Euphrasia (Scrophulariaceae): Die tetraploide E. minima. Pl Syst Evol. 151(3-4):241–269.
- Vitek E. 1998. Are the taxonomic concepts of agamospermous genera useful for autogamous groups – A critical discussion using the example of Euphrasia (Scrophulariaceae. Folia Geobot. 33(3):349–352.
- Vitek E. 2000. Euphrasia inopinata Ehrendorfer & Vitek (Scrophulariaceae) in Salzburg. Wulfenia. 7:83–86.
- Vos P, Hogers R, Bleeker M, Reijans M, van de Lee T, Hornes M, Frijters A, Pot J, Peleman J, Kuiper M. 1995. AFLP: a new technique for DNA fingerprinting. Nucleic Acids Res. 23(21):4407–4414.
- White TJ, Bruns T, Lee STaylor J., 1990. Amplification and direct sequencing of fungal ribosomal RNA Genes for phylogenetics. In: Sninsky JJ, Gelfand DH, White TJ, Innis MA, editors. PCR protocols: A guide to methods and applications. San Diego (CA): Academic Press; p. 315–322.
- Winkler M, Escobar García P, Gattringer A, Sonnleitner M, Hülber K, Schönswetter P, Schneeweiss GM. 2017. A novel method to infer the origin of polyploids from Amplified Fragment Length Polymorphism data reveals that the alpine polyploid complex of Senecio carniolicus (Asteraceae) evolved mainly via autopolyploidy. Mol Ecol Resour. 17(5):877–892.
- Yeo PF. 1978. A taxonomic revision of Euphrasia in Europe. Bot J Linn Soc. 77(4):223–334.