ABSTRACT
This study explores the potential of nature-based solutions and indigenous knowledge in climate change adaptation. It focuses on the Rokohouia Delta located in Aotearoa, New Zealand where Ngāi Tūāhuriri, the Indigenous People of the region, employ strategies deeply rooted in their understanding of the interdependencies between humans and ecosystems, to improve environmental quality. The paper underscores the potential of nature-based solutions (NbS), closely aligned with indigenous ways of thinking, in mitigating hydro-meteorological risks associated with the impacts of climate change. While the study was initially focused on the role of indigenous-informed approaches to NbS on lessening climate change risks, it became apparent that NbS has important potential for cultural revitalisation and decolonisation through the regeneration of mahinga kai (wild food gathering areas), addressing long-held Ngāi Tūāhuriri grievances associated with the destruction of deltaic ecosystems on which it’s culture depends. The findings underscore the potential of NbS in complementing conventional infrastructure (responses/decisions in mitigating hydro-meteorological risks), enhancing social cohesion, and promoting ecological consciousness. The study also concludes that indigenous knowledge can potentially play a crucial role in informing infrastructure choices that integrate nature-based solutions, and thereby facilitate both cultural revitalisation and climate change adaptation.
Introduction
This paper explores the deep-seated cultural and historical ties between the Ngāi Tūāhuriri, a hapū (sub-tribe) of the Ngāi Tahu iwi (tribe), and the Rokohouia Delta located in the Canterbury region. It investigates how these ties shape the community's response to hydro-meteorological risks associated with climate change related extreme events. The Ngāi Tūāhuriri worldview, informed by their rich understanding of the interdependencies between humans and ecosystems, forms the foundation of their strategies for climate adaptation and addressing land use change and environmental degradation. The concept of mauri, central to this worldview, is seen as the vitality or life-generating capacity of various ‘bodies’, including ecosystems and communities, while the concept of whakapapa informs the relationships and interdependencies between them. Furthermore, the philosophy of ‘ki uta ki tai’ (the connection from the mountain to the sea) encourages whole-of-catchment adaptation responses to hydro-meteorological risks based on ecosystem interconnectivity.
The paper underscores the potential of nature-based solutions (NbS) to complement conventional ‘grey’ or ‘hard’ infrastructure. Emerging from the field of applied ecology, NbS involves the protection, sustainable management, and restoration of natural or modified ecosystems to deliver a range of services. An example of how NbS can complement grey infrastructure is where the reconstruction of wetlands and forests can reduce flooding during extreme rainfall events, which may in turn reduce the costs associated with the construction and maintenance of flood protection infrastructure such as levees, flood walls, and detention basins. These NbS, which closely align with indigenous ways of thinking and working with nature, are presented as opportunities for communities to engage with their environment in a sustainable and resilient manner, while also acknowledging and respecting their historical and cultural ties to the local environment. Considering the need for enhanced climate change resilience in the Rokohouia Delta, the paper argues that NbS hold potential for cultural revitalisation and decolonisation through the regeneration of mahinga kai (wild food gathering areas), addressing long-held grievances associated with the destruction of the ecosystems that defined the Ngāi Tūāhuriri culture. This work provides a platform for a nuanced understanding of how cultural, historical, and ecological perspectives can shape infrastructure choices in the face of environmental challenges.
Background
In Aotearoa New Zealand, Ngāi Tūāhuriri are Indigenous People with recognised territory on the east side of the South Island between the Hakatere and Hurunui rivers (Te Rūnanga O Ngāi Tahu Act Citation1996). Historically hunter-gatherers, they were primarily sustained by an extensive and interconnected braid-delta system of abundant wetlands, rivers, streams, estuaries, and spring-fed coastal lagoons. It was named Ka Whata Kai a Te Rakihouia, or The Food Storehouse of Rakihouia in reference to the abundant wild foods present. However, colonisation alienated Ngāi Tūāhuriri from their land and resources (Evison Citation1997), and subsequent land clearance and drainage dramatically modified the delta system. For example, less than ten percent of the original wetland ecosystem extent remains (StatsNZ Citation2018). shows the extent of wetlands in the area prior to European settlement as compared to the present day. The map shows prior existence of large wetlands between Kaiapoi and Rangiora, and networks of wetlands along the coast connecting the mouths of the Rakahuri (or Ashley) and Waimakariri rivers. shows vegetation cover according to the original survey maps (mid-1800 s) of the coastal delta region, which stretches from the Waimakariri river south through present-day Ōtautahi (Christchurch) to the coastal lagoon Te Waihora, an area approximately 80 km long by 20 km wide. These maps, referred to as the black maps were digitalised by Ngāi Tūāhuriri and are considered by them to be accurate reflections of the extent of wetlands prior to colonisation.
Figure 1. Predicted historic and current extent of coastal wetlands in the study area.
Source: Drawn in ArcGIS using data from the Environment Canterbury Open Data Portal: http://data.test.ecan.govt.nz/.
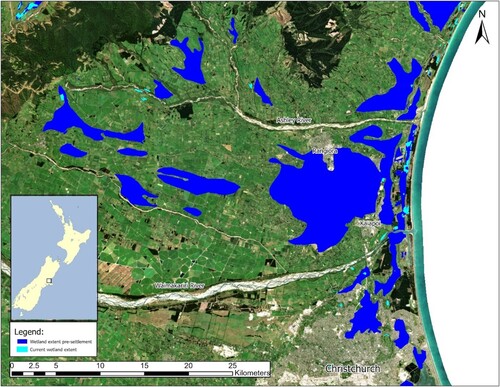
Figure 2. Vegetation cover according to original survey of coastal delta region (Canterbury Maps Citationn.d.).
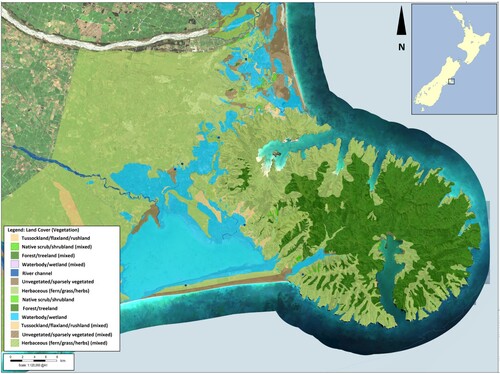
For over 150 years Ngāi Tūāhuriri has continually sought redress for alienation from their lands and resources. The primary mechanism of this alienation was Kemp’s Deed, a land purchase deal with the Crown (the New Zealand Government), where in exchange for providing land for settlers, Ngāi Tūāhuriri was guaranteed ongoing access to their mahinga kai (wild food gathering areas) and extensive reserve lands for their villages (Waitangi Tribunal Citation1991). In essence, Kemp’s Deed required that the Crown safeguard the braid-delta system as an important mahinga kai for Ngāi Tūāhuriri. Having failed to do so, in 1997 the Crown engaged in a negotiated settlement process with the broader tribal group Ngāi Tahu, which includes Ngāi Tūāhuriri. The Ngāi Tahu Deed of Settlement (Citation1997, s. 2, p. 3) included an apology which acknowledged that the Crown had acted ‘unconscionably’ and had ‘failed to allow reasonable access to traditional sources of food’.
The wholesale modification of the braid-delta system and ninety percent reduction in the extent of wetlands was not only detrimental to Ngāi Tūāhuriri’s ability to access traditional foods and resources, but it also impacted Ngāi Tūāhuriri culture. Ngāi Tūāhuriri cosmology is intertwined with the delta, with streams, rivers, estuaries, and wetlands constituting wai tīpuna (ancestor-relations) and sites such as waipuna (springs) representing wahi tapu (sacred areas). The mātauranga, or knowledge of how to live in the delta-braid system is contained in the mythology, stories, rituals, practices, knowledge, and art of Ngāi Tūāhuriri. The maintenance of mahinga kai and Ngāi Tahu culture are interdependent (O’Regan Citation1989), and the braid-delta ecosystem lifestyle is embedded in the Ngāi Tūāhuriri cultural consciousness. The remnants of the braid-delta system enable Ngāi Tūāhuriri to continue their foraging, fishing, and hunting practices, albeit to a much more limited degree, and the stories, mythology, and rituals that evolved in the delta continue to be passed on. However, in a very real sense Ngāi Tūāhuriri is a deltaic remnant culture that has become marooned in a landscape modified in accordance with the cultural frame of British settlers. These settlers set about ‘taming’ the delta through converting what they viewed as a wild and inhospitable environment to agricultural and urban land uses. Such action was fuelled by colonial narratives of bringing order and progress to terra nullius – ‘empty land’ supposedly owned by no one (Tau Citation2000).
The original contract with the Crown to protect Ngāi Tūāhuriri access to mahinga kai continues to be breached post-settlement. Since 2003, there has been significant intensification of agriculture in the Canterbury region, with both forestry and dryland sheep and beef farming being replaced with irrigated dairy farming in the plains and low-altitude areas (StatsNZ Citation2019). This, alongside ongoing encroachment by agriculture on the region’s braided rivers (Grove et al. Citation2015), has caused nutrient and sediment contamination of waterways, which in turn has further degraded remaining mahinga kai.
Climate change impacts
In 2010 and 2011, Christchurch city located in the centre of the historic Rokohouia Delta experienced a significant series of earthquakes that caused coastal ground subsidence of up to 0.5 m (Orchard et al. Citation2020), creating the equivalent of one hundred years of climate change-induced sea level rise (Hughes et al. Citation2015). The ground subsidence impacted shallow groundwater dynamics, causing the soil to lose its strength and become saturated, resulting in a higher liquefaction potential (Monk et al. Citation2015). A considerable area of the eastern Christchurch suburbs was zoned uninhabitable due to future flood risk and liquefaction-prone soils, impacting 7,500 households (LINZ Citation2021). Much of this ‘red zone’ comprised low-lying areas adjacent to streams and rivers that, prior to drainage by settlers, were wetlands within the delta system. On top of the earthquake-induced subsidence, future climate change-induced sea level rise is expected to further inundate coastal areas. Plausible scenarios suggest that by 2100 following a potential one metre rise in sea levels, up to 25,000 properties in Christchurch will be impacted by coastal flooding during extreme rainfall events (Tonkin + Taylor Citation2021). Due to this combination of earthquake induced subsidence and sea level rise, the coastal wetland areas of the delta system are reemerging and will continue to expand over the coming decades. Local and regional authorities have determined managed retreat to be the only viable option to deal with this challenge in the long-term, as the continued development of grey infrastructure to protect property and the built environment is financially unfeasible (Hurunui District Council Citation2023). The impact of climate change related flooding on lives, property, and the built environment will likely be extensive and traumatic.
In addition to driving sea level rise, it is anticipated that climate change will also increase the intensity and frequency of storms (Ministry for the Environment Citation2017; NIWA Citation2017). These two factors combined render coastal and low-lying communities vulnerable to flooding from storm surges or heavy rainfall in their catchments. Research suggests that climate change has made flood events in Canterbury both more likely and more severe (Tonkin + Taylor Citation2021). It was also found that the extreme rainfall that occurred in Canterbury in May 2021 was ten to fifteen percent more intense because of human influence on the climate system, and that such events are at least twenty percent more likely to occur today compared to preindustrial times, when the atmosphere was about one degree colder (Metservice Citation2021). Additionally, modelling has indicated that these heavy rainfall events are likely to increase in intensity as the atmosphere continues to warm, with much of Canterbury expected to see a 100% increase in flood events by the end of the century (Macara et al. Citation2020). Growing flood risk will compound with increases in the value and volume of assets and built infrastructure on flood plains. The economy and culture of coastal communities are at significant risk. Furthermore, intensifying storms will affect hydrology, ecological distributions, and patterns of erosion and sediment deposition in coastal areas (Michener et al. Citation1997; Bell et al. Citation2000) and beyond.
As weather extremes become more pronounced, the likelihood of extended dry periods and drought also increases (Dai Citation2013; Ault Citation2020). The Canterbury Region is projected to see less rainfall and become more arid overall, especially in winter (Ministry for the Environment Citation2018). Lower winter rainfall means lower flows in hill-fed rivers like the Rakahuri and reduced land surface recharge to groundwater, translating to lower flows in spring-fed waterways and wetlands. Warmer and wetter winters on the South Island’s West Coast may also result in reduced snowfall, leading to rivers with higher winter but lower summer flows in alpine catchments like the Waimakariri (Jenkins Citation2018). Reduced surface and groundwater flows also increase the risk of saltwater intrusion into coastal wetlands (Moomaw et al. Citation2018) and aquifers (Ingham et al. Citation2006), a risk compounded by sea level rise. Dry conditions and high temperatures will also increase evapotranspiration and drive demand for irrigation water, potentially placing additional stress on groundwater and surface water bodies. The interaction of climatic, biophysical, and social factors can all compound the impacts of drought.
Human modification of river corridors also plays a significant part in increasing climate-related risk. River morphology and flow regimes are affected by the alteration of river channels and braid plains, such as through flood control schemes or river channelisation (Hohensinner et al. Citation2018). Without constant riverbed management and gravel extraction, bed level aggradation will heighten flood risk in modified river corridors. In addition to large-scale river floods, significant damage is also caused by the flooding of smaller watercourses, and by surface flooding in built-up areas. Efforts to mitigate this risk by modifying river channels and implementing flood control schemes can inadvertently exacerbate negative impacts on remaining ecosystems. For instance, anthropogenic distortion of natural flow regimes is one of the greatest pressures on freshwater communities of fish and other biota (Bragg et al. Citation2005; Tonkin et al. Citation2018). There are movements internationally, which are gaining traction in New Zealand, to provide rivers with more space to move across landscapes as a strategy for sustainable flood risk management (Asselman and Klijn Citation2016). Such approaches align with core Māori values, and in particular allowing rivers their natural expression as wai tipuna – a core component in restoring their mauri (Brierley et al. Citation2019; Brierley et al. Citation2022). Concurrently, the escalating frequency and intensity of drought, coupled with growing water extraction to support intensive dairy farming, results in adverse consequences for aquifers, river and stream flows, and wetlands. These issues are further compounded by the influx into the system of nutrients and sediment from agricultural activities.
A Ngāi Tūāhuriri strategy for adapting to climate change and addressing environmental degradation
Climate change presents significant adaptation challenges and pressures. Rising sea levels and increased heavy rainfall combine to pose considerable risk to property, the built environment, and community well-being through flooding. This is particularly concerning for Ngāi Tūāhuriri communities, as their homes are situated in vulnerable areas. Ngāi Tūāhuriri also depend on sustainable ecosystems for their mahinga kai, and without effective mitigation and adaptation strategies, the projected impacts of climate change, flood control measures, and intensive agriculture will have cumulative detrimental effects.
In 2022, Te Kāhui Kahukura (TKK), a collaboration between Ngāi Tūāhuriri and territorially adjacent hapū (clans), developed a forward-thinking and pioneering wellbeing index (Reid Citation2022) to inform and support urban and environmental planning and development in Christchurch and the surrounding Canterbury region. TKK members have constitutionally established governing relationships with local Crown authorities – the index establishes several strategic targets to be attained by the year 2070 via these partnerships. Among these are several focussed on the reconstruction and restoration of key ecosystems including dunelands, wetlands, coastal lagoons, and forests to at least twenty-five percent of their original extent across catchments.
This strategy is informed by an underpinning ontology that frames human and ecological relationships in terms of interdependencies. Mauri, as described above, is a Māori concept used to gauge the quality of such interdependencies, and refers to the vitality, or life generating and supporting capacity, of various ‘bodies’, including ecosystems and communities (Mead Citation2016; Reid and Rout Citation2019). Changes in the mauri of one body will have corresponding impacts on the mauri of other bodies, reflecting the interdependency between them (Mead Citation2016; Reid and Rout Citation2019). For example, restoring a wetland may result in the mauri, or quality, of the water flowing from that wetland to increase, which will in turn increase the mauri of the river or stream into which it flows, and also increase the mauri of the community that forages in or takes water from the waterbodies.
While mauri gauges the quality of relationships between bodies, the bodies themselves are categorised according to the concept of whakapapa. Whakapapa describes the genealogy of creation, where bodies including the land, waters, and sky are understood as the forebears of living bodies and their ecological communities, and which are evolving from each generation to the next as an interdependent family (Mead Citation2016; Rout et al. Citation2021). People are not ‘separate’ from the land but exist within the same chains of relationship and descent (Tau Citation2000; Mead Citation2016). Humans are seen as having an ethical obligation not to diminish the mauri of ecological communities that they exist within and act upon. This ethic and associated practices is known as kaitiakitanga. It is from this that the desire to restore ecosystems emerges: to facilitate the enhancement of mauri between the family of ecological bodies.
The convergence between indigenous thinking and nature-based solutions
The TKK and Ngāi Tūāhuriri strategies constitute nature-based solutions for climate change adaptation. This is not to suggest that these indigenous groups oppose hard engineering solutions or the development of grey infrastructure to protect property and the built environment, particularly given that their own communities are threatened by extreme climate events. It is simply that such solutions must be judged in terms of their effectiveness in synergistically increasing the mauri of human and ecological communities. The approach of Ngāi Tūāhuriri, although emerging from a different ontology, fits well with ecosystem reconstruction solutions that are gaining influence within the planning, engineering, and in particular, the science community. Such approaches may be termed more specifically blue–green infrastructure development. Blue–green infrastructure development emphasises an optimal balance between decentralised (natural ecosystems) and centralised (hard or grey infrastructure) in water management systems to optimise ecological, cultural, economic, and social outcomes (Dreiseitl et al. Citation2001).
Wetlands
The environmental and social benefits of Ngāi Tūāhuriri’s emphasis on wetland restoration and reconstruction at appropriate sites in the braid-delta system are demonstrated by the science literature. It outlines that wetlands are effective in intercepting run-off and mitigating flooding, while creating benefits in downstream water quality, biodiversity, habitat provision, and carbon sequestration (Zedler Citation2003). Historically, river margins contained a mix of swamps, wetlands, and lowland vegetation that aided flood abatement, particularly in lower reaches of the braid-delta system. Wetlands mitigate flooding by detaining flood flows as surface water, and by acting like a sponge by holding significant amounts of water in their specially adapted vegetation and deep soils (Ming et al. Citation2007), which is then released gradually to downstream areas. However, effective flood mitigation via wetland restoration requires coordinated catchment-scale planning (Zedler Citation2003). Networks of small wetlands in the upper reaches of a catchment can significantly reduce and delay flood peaks, but extensive downstream storage systems may still be required to mitigate flood damage. These downstream systems may incorporate natural wetlands but are also likely to require some degree of engineering design (Kapetas and Fenner Citation2020).
Constructed wetlands and stormwater infiltration basins help mitigate flooding by intercepting first flush and peak flows during major rain events and bring water quality benefits by treating stormwater. These are typically engineered systems that incorporate natural elements such as vegetation and microbes to treat water and can thus deliver a range of co-benefits in biodiversity, recreation and amenity value, and micro-climatic/temperature regulation. While constructed wetlands and basins are not a full substitute for natural ecosystems, they can complement ‘hard infrastructure’ such as conventional wastewater and stormwater treatment facilities. Blue–green stormwater infrastructure can have significant local benefits in and around residential and commercial areas, and with sufficient uptake it can also have benefits at the catchment scale, through regulating flows and nutrient fluxes (Pennino et al. Citation2016), and protecting receiving environments such as rivers, streams, and estuaries.
Coastal wetlands also play a very important role in mitigating the impacts of storms. Researchers have estimated that the value of coastal wetlands for storm protection globally is around US$447 billion, or US$11,000 per hectare per year (Costanza et al. Citation2021). The climate risk mitigation function of coastal wetlands depends on coastal vegetation and its interaction with coastal geomorphology. Wetland vegetation decreases storm surges and waves while maintaining shallow water depths, making coastal wetlands such as estuaries, mangroves, and salt marshes a buffer against storms (Mulder et al. Citation2020; Costanza et al. Citation2021). In addition to storm surges and wave action, coastal wetlands have even been shown to mitigate damage from tsunamis (Van Coppenolle et al. Citation2018; Ruangpan et al. Citation2020). A large volume of research in this field has focused on the role of coral reefs and mangrove swamps in lessening the impact of tropical storms and cyclones (e.g. Alongi Citation2008; van Zanten et al. Citation2014; Krauss and Osland Citation2019). Of more relevance in New Zealand is the role of estuaries, salt marshes, and coastal swamps.
Forests
Ngāi Tūāhuriri also place emphasis on forest restoration and reconstruction. Forests are one of the most significant biomes in the provision of hydrological ecosystem services (Brauman et al. Citation2007), but the effects of forests on catchment hydrology are complex and highly site dependent. The protection of forests and afforestation or revegetation in certain catchment areas may help to mitigate impacts of flooding. Forests can help absorb surface runoff, reduce flood peaks, and mitigate flooding during small to medium rainfall events (Carvalho-Santos et al. Citation2014). However, research has also suggested that forests may not play a flood mitigation role during major rainfall events, and in the case of commercial plantation forests or poorly planned forest restoration may even exacerbate flooding (Calder and Aylward Citation2006). Even if the effect of forests on flood flows is limited, there is often a case for afforestation and restoration for the wide range of other ecosystem services provided – including erosion and sediment control, carbon sequestration, habitat and biodiversity creation, and local micro climatic regulation (Lavorel et al. Citation2015). The use of forested riparian buffers on large braided rivers would require special attention to the natural state of these river systems, which do not always have an easily identifiable channel or bank, and are naturally fringed by large swathes of wetlands and dynamic mixed habitat (Piégay et al. Citation2006; Gray and Harding Citation2011;). Nevertheless, research in New Zealand has found that the costs of riparian afforestation in agricultural areas are often outweighed by significant environmental benefits (Daigneault et al. Citation2017).
Dunelands
Alongside wetlands, coastal dune systems are an integral part of the braid-delta landscape and resource base in the Ngāi Tūāhuriri territory. These dynamic systems play an important role in protecting and sheltering coastal ecosystems and communities. It is increasingly recognised that conventional approaches to coastal protection (e.g. seawalls, breakwaters) are expensive, non-adaptive, and require ongoing maintenance (Narayan et al. Citation2016). Demand is growing for the development of sustainable nature-based solutions to coastal climate hazards (Janssen et al. Citation2015; Morris et al. Citation2019), with investment in nature-based coastal defences increasing worldwide (Narayan et al. Citation2016). Such solutions can potentially address both mounting pressures from climate change and the environmental impact of coastal zone development (the ‘coastal squeeze’), which are combining to increase exposure and vulnerability (Chávez et al. Citation2021). A growing body of research examines how healthy coastal ecosystems can reduce the vulnerability of people and property to these pressures (Langridge et al. Citation2014).
Taylor et al. (Citation2015) have documented how sandy beaches and dune systems dampen wave energy and protect infrastructure against erosion and storm surge flooding. Natural and restored dune systems can be superior to hard engineered coastal defence structures in buffering capacity and resilience (Wamsley et al. Citation2011), much cheaper to create and maintain (Narayan et al. Citation2016) and can provide beneficial habitat and connected ecosystem services (Everard et al. Citation2010; Barbier et al. Citation2011; Morris et al. Citation2018). However, storms can disturb duneland ecosystems in the short-term, and climate change processes can gradually shift dune-based plant communities and affect ecological succession, so the protection of dune ecosystems is a priority for the social-ecological resilience of coasts.
Coastal dune systems also serve to protect areas further inland by sheltering them from waves and wind during storm events. Dune systems are highly dynamic environments, constantly being re-shaped through the interactions of geology, climate, and vegetation (Miller et al. Citation2009). This dynamism is fundamental to the resilience of dunelands, and the modification of dune vegetation or morphology can throw dune systems out of dynamic equilibrium (Stallins Citation2005), affecting their capacity to re-stabilise following storm disturbance. Ecological restoration of dune systems, including the removal of inappropriate exotic species, can help to restore the storm buffering capacity of dunes (Bergin Citation2011).
In general, coastal habitats – including coastal wetlands, dune systems, and others – directly intercept and attenuate wind and wave energy, and also physically shape coastal landscapes to better handle storms and sea level rise (Arkema et al. Citation2013; Möller et al. Citation2014; Leonardi et al. Citation2018). Recognition of the potential for naturally adaptive vegetation and ecosystems to mitigate coastal hazards has stimulated widespread uptake of nature-based coastal adaptation solutions. For example, varieties of ‘living shoreline’ are being implemented alongside conventional coastal protection structures in a range of settings worldwide (Davis et al. Citation2015; Moosavi Citation2017; Smith et al. Citation2018). These can entail varying degrees of protection, restoration, or enhancement of existing natural coastal systems, as well as elements of constructed coastal wetland and shoreline (Chambers et al. Citation2021).
Ki uta ki tai – landscape connectivity and resilience
While the reconstruction and restoration of discrete ecosystems is one element of adapting to climate change, creating maximum impact requires considering the connectivity between multiple ecosystems. As argued above, careful catchment-level landscape planning is required to identify optimal areas for ecosystem restoration and reconstruction in combination with hard infrastructure such as flood controls and water treatment facilities. In the Māori worldview, such planning should be guided by an ontological position that encourages the collective enhancement of mauri among connected ecological and hard infrastructure bodies. Ngāi Tūāhuriri, and many iwi (Māori tribes), call this holistic approach to landscape management ‘ki utu ki tai’, meaning ‘mountains to the sea’ (Crow et al. Citation2020). Connected by the flow of water across the landscape, healthy ecosystem bodies work together to enhance the mauri of each, as well as the human communities sustained by them. Systems like wetlands and swamps often play a central role in connecting terrestrial, freshwater, and coastal/marine systems (Alexander et al. Citation2018), as well as maintaining critical hydrologic, biogeochemical, and biological processes within catchments. These synergistic relationships help mitigate the negative impacts of extreme weather events, industrial agriculture, and coastal inundation – all of which are harms exacerbated by climate change. Science demonstrates that patchwork landscapes of multiple ecosystem types can more effectively buffer against droughts, flood events, and storms through the provision of a host of complementary ecosystem services (Alexander et al. Citation2018).
Drought
Landscapes with high connectivity between diverse ecosystems are more resilient to drought (Wang et al. Citation2019; Fanok et al. Citation2021; Wunder et al. Citation2021). Just as wetlands help mitigate floods by holding back flood water, they also play an important part in mitigating drought by storing and slowly releasing water back into the catchment. Wetland soils are often able to support vegetation through dry spells and thus play an important role in sustaining ecological communities and systems. Depending on their composition and character, riparian buffers can work in a similar way to mitigate drought risk by retaining water, providing shade, and regulating temperature and microclimates (Ellison et al. Citation2017). Healthy wetlands and riparian buffers can therefore provide refugia for indigenous fish, amphibians, and birds that could otherwise be threatened by drought conditions (Chapman et al. Citation1996).
Vegetation plays an important role in modifying some of the key processes that propagate drought conditions, such as evaporation (e.g. from surface water and soil) and evapotranspiration (Van Loon Citation2015). Thus, the maintenance of appropriate vegetation cover across a variety of connected ecosystems can also help allay the onset of drought. Also helpful in delaying or preventing drought is slowing water flow through the catchment through wetland restoration or other nature-based approaches, as well as increasing catchment storage in soil, vegetation, and water bodies. In areas where land drainage predominates, a viable strategy may be to decommission drains and drainage infrastructure to retain water on the land surface and in the soil (Zedler and Kercher Citation2005; Erwin Citation2008).
Storms and floods
Reestablishing connectivity among fragmented ecosystems at a ‘mountains to sea’ scale also helps to reduce impacts of storms and floods. Although the coast itself often bears the brunt of major storm events, storm damage affects other parts of the landscape such as rivers, riparian margins, hillslopes, and built-up areas – particularly where there has been a high degree of modification of the natural environment. Harms are mainly attributed to flooding (both riverine and surface), winds, and resultant erosion. As noted above, waterways that have been morphologically altered are often more flood prone. The clearing of vegetation can make catchment areas prone to erosion and slips, especially steeper hillslopes (Marzen et al. Citation2017). It also allows the delivery of considerable overland flows to rivers and streams, and resultant sediment mobilisation, transport and downstream deposition, exacerbating flooding and sedimentation. Built-up areas generate significant surface runoff from roofs and other impermeable surfaces, which contributes to unnaturally volatile flows in urban streams and drains (Walsh et al. Citation2005). Blue–green infrastructure is an increasingly central pillar in water-sensitive urban design, and facilities such as constructed wetlands, stormwater basins, swales, and rain gardens all contribute to storm resilience at scales ranging from individual buildings to entire catchments (Mottaghi et al. Citation2016; Rosenzweig et al. Citation2019).
Rivers, streams, and their associated corridors and riparian areas can provide a wide range of ecosystem services alongside flood mitigation. Much international research has examined the merits of providing more room for rivers as a strategy for sustainable flood risk management (de Groot and de Groot Citation2009; Klijn et al. Citation2013; Janssen et al. Citation2015). In addition to mitigating flood risk, retiring and restoring riparian areas can generate a range of improvements in water quality, habitat, and biodiversity, as well as recreational and community benefits (Kousky and Walls Citation2014). With a focus on flooding among a range of hydro-meteorological risks, Ruangpan et al. (Citation2020) find evidence for the effectiveness of nature-based solutions at both small (e.g. building, street) and large (e.g. catchment, region) scales, provided that solutions are tailored to local conditions.
The importance of creating new delta-river systems in mitigating the impacts of climate change – a grey-blue–green infrastructure
The above analysis demonstrates the strong connection between the indigenous ontology of Ngāi Tūāhuriri and the science underpinning nature-based solutions to extreme weather events. Although both land on the same solution, the metaphysical underpinning of each has different origins and many lead to differences in NbS strategies. As outlined previously, for Ngāi Tūāhuriri whakapapa is the ontological grounding for understanding the relationship between different atua, or environmental domains, with ki utu ki tai encouraging mauri and mana enhancing relationships as domains intersect from mountains to the sea. This is a relational way of perceiving the environment where personhood is a fundamental attribute of both people and the environment (Reid and Rout Citation2016, Citation2018). Conversely, from a Western science perspective, the environment is perceived through a mechanistic and physicalist lens, whereby environmental systems (e.g. hydrological, geological, and biological) are understood in terms of their functionality and instrumentality – for instance the functions required to deliver ecosystem services (Reid and Rout Citation2016, Citation2018). In short, for Ngāi Tūāhuriri NbS concerns enhancing positive relationality, whereas from the Western science position NbS involves improving environmental functionality. While the two positions may to some extent be complementary, they may also come into conflict. Western science is likely to focus on NbS that restore ecosystems to the minimum viable function required to deliver sought after ecosystem services, whereas Ngāi Tūāhuriri is more likely to focus on NbS that reestablish positive relationality – a standard that may exceed minimum viable function and be informed by mātauranga Māori.
Regardless the restoration of interconnected braid-delta ecosystems has the potential to restore past functionality and positive relationality to the landscape of the Rakahuri and Waimakariri catchments. Not only would such restoration assist with community adaptation to climate change risk, it also creates an opportunity for the reinvigoration of the deltaic culture of Ngāi Tūāhuriri through the regeneration of mahinga kai – addressing long-held grievances associated with the destruction of the ecosystems that defined a culture. The inevitable return of coastal ecosystems through sea level rise, while having deleterious effects on property, built environment, and the communities dwelling therein, perhaps also offers opportunity for the restoration of Ngāi Tūāhuriri mahinga kai. Researchers have advocated for the idea of ‘coastal ecosystem mosaics’ to describe ecosystem complexes comprising ‘tightly interlinked coastal, estuarine, wetland and freshwater habitats at the interface of land and sea’ (Sheaves Citation2009, p. 107). In the context of Ka Whata Kai a Te Rakihouia, this mosaic also incorporates Ngāi Tūāhuriri as indigenous people and their mātauranga (knowledge). The restoration and reconstruction of coastal ecosystem mosaics in this case not only provides the range of previously outlined ecosystem services, but it regenerates mahinga kai and can rejuvenate associated indigenous knowledge and cultural practice.
Drawing together indigenous thinking and science through a framing of relationships and connectivity is helpful for Ngāi Tūāhuriri in pressing local and national authorities for whole-of-landscape planning approaches in the delta-braid system. Focused government and private investment into blue–green infrastructure in the study area, in addition to conventional grey infrastructure, is likely to enhance environmental resilience, and in turn, the wellbeing and resilience of local communities (Meurk and Swaffield Citation2000). At the same time, an avenue for cultural revitalisation and decolonisation among Ngāi Tūāhuriri is provided. Such investment offers a mutually reinforcing means by which to (re)build social-ecological resilience in the face of climate-related pressures (Adger et al. Citation2005; Folke et al. Citation2016).
Such nature-based solutions could be targeted at different scales of time and space within wider catchments or landscapes. Complementary interventions might be made in different parts of a catchment (Liu et al. Citation2016), or at different points in the local water cycle, or over different time periods. Long-term and large-scale strategies may involve returning space to large, braided rivers, which have been deprived of significant areas of available floodplain and confined to much narrower beds than what they historically occupied (Brierley et al. Citation2019). Restoring room for these rivers to move would entail modification of flood protection schemes, and retiring land that has been claimed from the riverbed for development over the years (Klijn et al. Citation2013). Similarly, many smaller waterways that have been straightened or diverted might be re-naturalised to bring back their meandering form – especially in farming areas. This can help slow flood waters and has numerous co-benefits for water quality and stream health (Bechtol and Laurian Citation2005; Heritage and Entwistle Citation2020).
Large-scale and long-term systemic restoration of ecosystems clearly requires significant resourcing, careful planning and consultation, and collaborative implementation. Such restoration is certainly more feasible in some areas than others. Research has indeed found that nature-based solutions are in some cases not economically viable (Shultz and Leitch Citation2003). There is now a considerable level of development on some floodplains, particularly the Waimakariri and the lower reaches of the Rakahuri. In the case of the Waimakariri, this includes residential and industrial areas in northern Christchurch and Kaiapoi, Christchurch International Airport, and other infrastructure such as major roads. Along certain reaches of both rivers, however, there may be scope to implement specific NbS or retire agricultural and other land for appropriate restoration to grassland, flax, wetland or other lowland vegetation. Also, where establishment of riparian buffers is difficult on the main rivers, there may be scope on key tributaries for nature-based flood mitigation and the conservation of riparian margins and flood plains.
The nature and extent of such investments may be decided according to the values and priorities of Ngāi Tūāhuriri and local communities, based on considerations such as flood risk management, the protection and restoration of remnant riparian ecosystems, or the mitigation of land use impacts such as sediment run-off (Stutter et al. Citation2021). Smaller incremental restoration projects can bring multiple benefits at the local scale, but long-term, large-scale interventions will be needed to address the impacts of climate change, land use change, and floodplain development, as the space required to attenuate flood flows and mitigate other climate-related hazards via nature-based solutions is often considerable (Thorslund et al. Citation2017). Furthermore, new investment in NbS and blue–green infrastructure will inevitably occur alongside ongoing investment in conventional ‘grey’ infrastructure. The balance of blue–green and grey infrastructure will vary based on what is needed and possible given spatial, technical, and financial constraints. However, where blue–green, nature-based solutions are achievable, they have the potential to complement conventional infrastructure while delivering social and ecological co-benefits (Moore and Hunt Citation2012).
Conclusion
Nature-based solutions offer important mechanisms to mitigate hydro-meteorological risks and the impacts of climate change. These solutions, emerging from applied ecology and climate adaptation policy and practice, and closely aligned with indigenous ways of thinking, are presented as opportunities for communities to engage with their environment in a sustainable and resilient manner. The particular mix of blue–green and grey infrastructure that is appropriate in a given setting will depend on spatial, technical, and financial constraints. However, where achievable, nature-based solutions complement conventional infrastructure while delivering multiple social and ecological co-benefits. Ngāi Tūāhuriri's strategies for climate adaptation and addressing environmental degradation, informed by their rich understanding of the interdependencies between humans and ecosystems, serve as a testament to the potential of indigenous knowledge in informing infrastructure choices. Opportunities for nature-based solutions to mounting climate change risks in the Ngāi Tūāhuriri territory also offer the potential for cultural revitalisation and decolonisation through the regeneration of mahinga kai (wild food gathering areas), addressing long-held grievances associated with the destruction of the ecosystems that define Ngāi Tūāhuriri culture. In conclusion, this work provides a nuanced understanding of how cultural, historical, and ecological perspectives can shape infrastructure choices in the face of environmental challenges. It underscores the potential of nature-based solutions in complementing conventional infrastructure, enhancing social cohesion, and promoting ecological consciousness. It also highlights the importance of indigenous knowledge in informing these choices and the potential for cultural revitalisation through the regeneration of traditional food gathering areas.
Disclosure statement
No potential conflict of interest was reported by the author(s).
Additional information
Funding
References
- Adger WN, Hughes TP, Folke C, Carpenter SR, Rockström J. 2005. Social-ecological resilience to coastal disasters. Science. 309:1036–1039. doi:10.1126/science.1112122.
- Alexander LC, Fritz KM, Schofield KA, Autrey BC, DeMeester JE, Golden HE, Goodrich DC, Kepner WG, Kiperwas HR, Lane CR, et al. 2018. Featured collection introduction: connectivity of streams and wetlands to downstream waters. JAWRA Journal of the American Water Resources Association. 54:287–297. doi:10.1111/1752-1688.12630.
- Alongi DM. 2008. Mangrove forests: resilience, protection from tsunamis, and responses to global climate change. Estuarine, Coastal and Shelf Science. 76:1–13. doi:10.1016/j.ecss.2007.08.024.
- Arkema KK, Guannel G, Verutes G, Wood SA, Guerry A, Ruckelshaus M, Kareiva P, Lacayo M, Silver JM. 2013. Coastal habitats shield people and property from sea-level rise and storms. Nature Climate Change. 3:913–918. doi:10.1038/nclimate1944.
- Asselman N, Klijn F. 2016. Making room for rivers: quantification of benefits from a flood risk perspective. E3S Web of Conferences. 7:12001. doi:10.1051/e3sconf/20160712001.
- Ault TR. 2020. On the essentials of drought in a changing climate. Science. 368:256–260. doi:10.1126/science.aaz5492.
- Barbier EB, Hacker SD, Kennedy C, Koch EW, Stier AC, Silliman BR. 2011. The value of estuarine and coastal ecosystem services. Ecological Monographs. 81:169–193. doi:10.1890/10-1510.1.
- Bechtol V, Laurian L. 2005. Restoring straightened rivers for sustainable flood mitigation. Disaster Prevention and Management: An International Journal. 14:6–19. doi:10.1108/09653560510583806.
- Bell R, Goring D, de Lange W. 2000. Sea-level change and storm surges in the context of climate change. Transactions of the Institution of Professional Engineers New Zealand: General Engineering. 27:1–10.
- Bergin D. 2011. Weed control options for coastal sand dunes: a review. Rotorua: Scion.
- Bragg OM, Black AR, Duck RW, Rowan JS. 2005. Approaching the physical-biological interface in rivers: a review of methods for ecological evaluation of flow regimes. Progress in Physical Geography: Earth and Environment. 29:506–531. doi:10.1191/0309133305pp460ra.
- Brauman KA, Daily GC, Duarte TK, Mooney HA. 2007. The nature and value of ecosystem services: an overview highlighting hydrologic services. Annual Review of Environment and Resources. 32:67–98. doi:10.1146/annurev.energy.32.031306.102758.
- Brierley G, Fuller I, Williams G, Hikuroa D, Tilley A. 2022. Re-imagining wild rivers in Aotearoa New Zealand. Land. 11:1272. doi:10.3390/land11081272.
- Brierley G, Tadaki M, Hikuroa D, Blue B, Šunde C, Tunnicliffe J, Salmond A. 2019. A geomorphic perspective on the rights of the river in Aotearoa New Zealand. River Research and Applications. 35:1640–1651. doi:10.1002/rra.3343.
- Calder IR, Aylward B. 2006. Forest and floods. Water International. 31:87–99. doi:10.1080/02508060608691918.
- Canterbury Maps. n.d. Interactive map of Canterbury, New Zealand Government. https://mapviewer.canterburymaps.govt.nz/?webmap=0db87348adef4595a91994a3dc85cefe.
- Carvalho-Santos C, Honrado JP, Hein L. 2014. Hydrological services and the role of forests: conceptualization and indicator-based analysis with an illustration at a regional scale. Ecological Complexity. 20:69–80. doi:10.1016/j.ecocom.2014.09.001.
- Chambers RM, Gorsky AL, Isdell RE, Mitchell MM, Bilkovic DM. 2021. Comparison of nutrient accrual in constructed living shoreline and natural fringing marshes. Ocean & Coastal Management. 199:105401. doi:10.1016/j.ocecoaman.2020.105401.
- Chapman LJ, Chapman CA, Chandler M. 1996. Wetland ecotones as refugia for endangered fishes. Biological Conservation. 78:263–270. doi:10.1016/S0006-3207(96)00030-4.
- Chávez V, Lithgow D, Losada M, Silva-Casarin R. 2021. Coastal green infrastructure to mitigate coastal squeeze. Journal of Infrastructure Preservation and Resilience. 2:7. doi:10.1186/s43065-021-00026-1.
- Costanza R, Anderson SJ, Sutton P, Mulder K, Mulder O, Kubiszewski I, Wang X, Liu X, Pérez-Maqueo O, Luisa Martinez M, et al. 2021. The global value of coastal wetlands for storm protection. Global Environmental Change. 70:102328. doi:10.1016/j.gloenvcha.2021.102328.
- Crow SK, Tipa GT, Nelson KD, Whitehead AL. 2020. Incorporating Māori values into land management decision tools. New Zealand Journal of Marine and Freshwater Research. 54(3):431–448. doi:10.1080/00288330.2020.1772322.
- Dai A. 2013. Increasing drought under global warming in observations and models. Nature Climate Change. 3:52–58. doi:10.1038/nclimate1633.
- Daigneault AJ, Eppink FV, Lee WG. 2017. A national riparian restoration programme in New Zealand: is it value for money? Journal of Environmental Management. 187:166–177. doi:10.1016/j.jenvman.2016.11.013.
- Davis JL, Currin CA, O’Brien C, Raffenburg C, Davis A. 2015. Living shorelines: coastal resilience with a blue carbon benefit. PLoS One. 10:e0142595.
- de Groot M, de Groot WT. 2009. “Room for river” measures and public visions in the Netherlands: a survey on river perceptions among riverside residents. Water Resources Research. 45. doi:10.1029/2008WR007339.
- Dreiseitl H, Grau D, Karl L. 2001. Waterscapes: planning, building and designing with water. Basel: Birkhauser.
- Ellison D, Morris CE, Locatelli B, Sheil D, Cohen J, Murdiyarso D, Gutierrez V, Noordwijk Mv, Creed IF, Pokorny J, et al. 2017. Trees, forests and water: cool insights for a hot world. Global Environmental Change. 43:51–61. doi:10.1016/j.gloenvcha.2017.01.002.
- Erwin KL. 2008. Wetlands and global climate change: the role of wetland restoration in a changing world. Wetlands Ecology and Management. 17:71–84. doi:10.1007/s11273-008-9119-1.
- Everard M, Jones L, Watts B. 2010. Have we neglected the societal importance of sand dunes? An ecosystem services perspective. Aquatic Conservation: Marine and Freshwater Ecosystems. 20:476–487. doi:10.1002/aqc.1114.
- Evison H. 1997. The long dispute: Maori land rights and European colonisation in southern New Zealand. Christchurch (NZ): Canterbury University Press.
- Fanok L, Beltrán B, Burnham M, Wardropper CB. 2021. Visions for large landscape drought resilience in rangelands. Rangelands. 43:47–56. doi:10.1016/j.rala.2020.11.003.
- Folke C, Biggs R, Norström AV, Reyers B, Rockström J. 2016. Social-ecological resilience and biosphere-based sustainability science. Ecology and Society. 21(3):41.
- Gray DP, Harding JS. 2011. Multi-scaled environmental determinism of benthic invertebrate communities in braided rivers: evidence for complex hierarchical controls on local communities. Fundamental and Applied Limnology. 179:3–15. doi:10.1127/1863-9135/2011/0179-0003.
- Grove P, Parker M, Gray D, Behrens F. 2015. Land use change on the margins of lowland Canterbury braided rivers, 1990–2012. Christchurch: Environment Canterbury Regional Council.
- Heritage G, Entwistle N. 2020. Impacts of river engineering on river channel behaviour: implications for managing downstream flood risk. Water. 12:1355. doi:10.3390/w12051355.
- Hohensinner S, Hauer C, Muhar S. 2018. River morphology, channelization, and habitat restoration. In: Schmutz S, Sendzimir J, editors. Riverine ecosystem management: science for governing towards a sustainable future. Cham: Springer; p. 41–65.
- Hughes MW, Quigley MC, van Ballegooy S, Deam BL, Bradley BA, Hart DE, Measures R. 2015. The sinking city: earthquakes increase flood hazard in Christchurch, New Zealand. GSA Today. 25:3–4.
- Hurunui District Council. 2023. Amberley beach coastal adaptation plan. [accessed 2023 Jul 27]. https://www.hurunui.govt.nz/repository/libraries/id:23wyoavbi17q9ssstcjd/hierarchy/Regulatory_Services/Coastal_conversations/Amberley_Beach/Amberley%20Beach%20CAP%20-%20FINAL.pdf.
- Ingham M, McConchie J, Wilson S, Cozens N. 2006. Measuring and monitoring saltwater intrusion in shallow unconfined coastal aquifers using direct current resistivity traverses. Journal of Hydrology (New Zealand). 45:69–82.
- Janssen SKH, van Tatenhove JPM, Otter HS, Mol APJ. 2015. Greening flood protection—an interactive knowledge arrangement perspective. Journal of Environmental Policy & Planning. 17:309–331. doi:10.1080/1523908X.2014.947921.
- Jenkins BR. 2018. Water management in New Zealand's canterbury region: a sustainability framework. Dordrecht: Springer.
- Kapetas L, Fenner R. 2020. Integrating blue-green and grey infrastructure through an adaptation pathways approach to surface water flooding. Philosophical Transactions of the Royal Society A: Mathematical, Physical and Engineering Sciences. 378:20190204. doi:10.1098/rsta.2019.0204.
- Klijn F, de Bruin D, de Hoog MC, Jansen S, Sijmons DF. 2013. Design quality of room-for-the-river measures in the Netherlands: role and assessment of the quality team (Q-team). International Journal of River Basin Management. 11:287–299. doi:10.1080/15715124.2013.811418.
- Kousky C, Walls M. 2014. Floodplain conservation as a flood mitigation strategy: examining costs and benefits. Ecological Economics. 104:119–128. doi:10.1016/j.ecolecon.2014.05.001.
- Krauss KW, Osland MJ. 2019. Tropical cyclones and the organization of mangrove forests: a review. Annals of Botany. 125:213–234.
- Langridge SM, Hartge EH, Clark R, Arkema K, Verutes GM, Prahler EE, Stoner-Duncan S, Revell DL, Caldwell MR, Guerry AD, et al. 2014. Key lessons for incorporating natural infrastructure into regional climate adaptation planning. Ocean & Coastal Management. 95:189–197. doi:10.1016/j.ocecoaman.2014.03.019.
- Lavorel S, Colloff MJ, Mcintyre S, Doherty MD, Murphy HT, Metcalfe DJ, Dunlop M, Williams RJ, Wise RM, Williams KJ. 2015. Ecological mechanisms underpinning climate adaptation services. Global Change Biology. 21:12–31. doi:10.1111/gcb.12689.
- Leonardi N, Carnacina I, Donatelli C, Ganju NK, Plater AJ, Schuerch M, Temmerman S. 2018. Dynamic interactions between coastal storms and salt marshes: A review. Geomorphology. 301:92–107. doi:10.1016/j.geomorph.2017.11.001.
- LINZ – Land Information New Zealand. 2021. Final Christchurch red zone demolition underway – published 21 September 2021. [accessed 2023 Jul 27]. https://www.linz.govt.nz/news/2021-09/final-christchurch-red-zone-demolition-underway.
- Liu Y, Theller LO, Pijanowski BC, Engel BA. 2016. Optimal selection and placement of green infrastructure to reduce impacts of land use change and climate change on hydrology and water quality: An application to the Trail Creek Watershed, Indiana. Science of the Total Environment. 553:149–163. doi:10.1016/j.scitotenv.2016.02.116.
- Macara G, Woolley J, Pearce P, Wadhwa S, Zammit C, Sood A, Stephens S. 2020. Climate change projections for the Canterbury Region. Report prepared by the National Institute of Water & Atmospheric Research (NIWA) – published February 2020.
- Marzen M, Iserloh T, de Lima JLMP, Fister W, Ries JB. 2017. Impact of severe rain storms on soil erosion: experimental evaluation of wind-driven rain and its implications for natural hazard management. Science of the Total Environment. 590-591:502–513. doi:10.1016/j.scitotenv.2017.02.190.
- Mead HM. 2016. Tikanga Maori (revised edition): living by Maori values. Wellington (NZ): Huia Publishers.
- MetService. 2021. Extreme weather event: Canterbury 28–29 May 2021. Media Release. https://about.metservice.com/assets/media-release/EWERAM-Canterbury-28-29May-2021-PR-9-Dec-21.pdf.
- Meurk CD, Swaffield SR. 2000. A landscape ecological framework for indigenous regeneration in rural New Zealand-Aotearoa. Landscape and Urban Planning. 50:129–144. doi:10.1016/S0169-2046(00)00085-2.
- Michener WK, Blood ER, Bildstein KL, Brinson MM, Gardner LR. 1997. Climate change, hurricanes and tropical storms, and rising sea level in coastal wetlands. Ecological Applications. 7:770–801. doi:10.1890/1051-0761(1997)007[0770:CCHATS]2.0.CO;2.
- Miller TE, Gornish ES, Buckley HL. 2009. Climate and coastal dune vegetation: disturbance, recovery, and succession. Plant Ecology. 206:97–104. doi:10.1007/s11258-009-9626-z.
- Ming J, Xian-guo L, Lin-shu X, Li-juan C, Shouzheng T. 2007. Flood mitigation benefit of wetland soil — a case study in Momoge National Nature Reserve in China. Ecological Economics. 61:217–223. doi:10.1016/j.ecolecon.2006.10.019.
- Ministry for the Environment. 2017. Preparing for coastal change: a summary of coastal hazards and climate change guidance for local government. Wellington: Ministry for the Environment.
- Ministry for the Environment. 2018. Climate change projections for New Zealand: atmospheric projections based on simulations undertaken for the IPCC 5th assessment, 2nd ed. Wellington: Ministry for the Environment.
- Möller I, Kudella M, Rupprecht F, Spencer T, Paul M, van Wesenbeeck BK, Wolters G, Jensen K, Bouma TJ, Miranda-Lange M, Schimmels S. 2014. Wave attenuation over coastal salt marshes under storm surge conditions. Nature Geoscience. 7:727–731. doi:10.1038/ngeo2251.
- Monk CD, van Ballegooy S, Hughes M, Villeneuve M. 2015. Liquefaction vulnerability increase at North New Brighton due to subsidence, sea level rise and reduction in thickness of the non-liquefying layer. Bulletin of the New Zealand Society for Earthquake Engineering. 49:334–340. doi:10.5459/bnzsee.49.4.334-340.
- Moomaw WR, Chmura GL, Davies GT, Finlayson CM, Middleton BA, Natali SM, Perry JE, Roulet N, Sutton-Grier AE. 2018. Wetlands In a changing climate: science, policy and management. Wetlands. 38:183–205. doi:10.1007/s13157-018-1023-8.
- Moore TLC, Hunt WF. 2012. Ecosystem service provision by stormwater wetlands and ponds – a means for evaluation? Water Research. 46:6811–6823. doi:10.1016/j.watres.2011.11.026.
- Moosavi S. 2017. Ecological coastal protection: pathways to living shorelines. Procedia Engineering. 196:930–938. doi:10.1016/j.proeng.2017.08.027.
- Morris RL, Konlechner TM, Ghisalberti M, Swearer S. 2018. From grey to green: efficacy of eco-engineering solutions for nature-based coastal defence. Global Change Biology. 24:1827–1842. doi:10.1111/gcb.14063.
- Morris RL, Strain EMA, Konlechner TM, Fest BJ, Kennedy DM, Arndt SK, Swearer SE. 2019. Developing a nature-based coastal defence strategy for Australia. Australian Journal of Civil Engineering. 17:167–176. doi:10.1080/14488353.2019.1661062.
- Mottaghi M, Aspegren H, Jönsson K. 2016. Integrated urban design and open storm drainage in our urban environments: merging drainage techniques into our city's urban spaces. Water Practice and Technology. 11:118–126. doi:10.2166/wpt.2016.016.
- Mulder OJ, Mulder KP, Kubiszewski I, Anderson SJ, Costanza R, Sutton P. 2020. The value of coastal wetlands for storm protection in Australia. Ecosystem Services. 46:101205. doi:10.1016/j.ecoser.2020.101205.
- Narayan S, Beck MW, Reguero BG, Losada IJ, van Wesenbeeck B, Pontee N, Sanchirico JN, Ingram JC, Lange G-M, Burks-Copes KA. 2016. The effectiveness, costs and coastal protection benefits of natural and nature-based defences. PLoS One. 11:e0154735.
- Ngāi Tahu Deed of Settlement. 1997. Section 2: Crown’s apology. Acknowledgements and Agreements. V1: s2.1.
- NIWA. 2017. Intensity of cyclones predicted to increase. Article published on 5 October 2017. [accessed 2023 Nov 15]. https://niwa.co.nz/news/intensity-of-cyclones-predicted-to-increase.
- Orchard S, Hughey KFD, Schiel DR. 2020. Risk factors for the conservation of saltmarsh vegetation and blue carbon revealed by earthquake-induced sea-level rise. Science of the Total Environment. 746:141241. doi:10.1016/j.scitotenv.2020.141241.
- O’Regan T. 1989. The Ngāi Tahu claim. In: IH Kawharu, editor. Waitangi: Maori & Pakeha perspectives of the treaty of Waitangi. Auckland: Oxford University Press; p. 234–262.
- Pennino MJ, McDonald RI, Jaffe PR. 2016. Watershed-scale impacts of stormwater green infrastructure on hydrology, nutrient fluxes, and combined sewer overflows in the mid-Atlantic region. Science of the Total Environment. 565:1044–1053. doi:10.1016/j.scitotenv.2016.05.101.
- Piégay H, Grant G, Nakamura F, Trustrum N, Petts GE. 2006. Braided river management: from assessment of river behaviour to improved sustainable development. In: Sambrook Smith GH, Best JL, Bristow CS, editors. Braided rivers: process, deposits, ecology and management. Malden: Blackwell; p. 257–276.
- Reid J. 2022. Te Kāhui Kahukura: Urban Wellbeing Index. Ngāi Tahu Research Centre. https://www.canterbury.ac.nz/ntrc/research/ntrc-contemporary-research-division/contemporary-research-division-publications/Huritanga-Paper-07-07-JR—MR-(003)-(002).pdf.
- Reid J, Rout M. 2016. Getting to know your food: the insights of indigenous thinking in food provenance. Agriculture and Human Values. 33:427–438. doi:10.1007/s10460-015-9617-8.
- Reid J, Rout M. 2018. Can sustainability auditing be indigenized? Agriculture and Human Values. 35(2):283–294. doi:10.1007/s10460-017-9821-9.
- Reid J, Rout M. 2019. Developing sustainability indicators – the need for radical transparency. Ecological Indicators. doi:10.1016/j.ecolind.2019.105941.
- Rosenzweig B, Ruddell BL, McPhillips L, Hobbins R, McPhearson T, Cheng Z, Chang H, Kim Y. 2019. Developing knowledge systems for urban resilience to cloudburst rain events. Environmental Science & Policy. 99:150–159. doi:10.1016/j.envsci.2019.05.020.
- Rout M, Awatere S, Mika J, Reid J, Roskruge M. 2021. Te Ao Tūroa, Te Ao Hurihuri, Te Ao Mārama—the old world, a changing world, a world of light: a Māori approach to environmental economics. Oxford: Oxford Encyclopaedia of Environmental Economics.
- Ruangpan L, Vojinovic Z, Di Sabatino S, Leo LS, Capobianco V, Oen AMP, McClain ME, Lopez-Gunn E. 2020. Nature-based solutions for hydro-meteorological risk reduction: a state-of-the-art review of the research area. Natural Hazards and Earth System Sciences. 20:243–270. doi:10.5194/nhess-20-243-2020.
- Sheaves M. 2009. Consequences of ecological connectivity: the coastal ecosystem mosaic. Marine Ecology Progress Series. 391:107–115. doi:10.3354/meps08121.
- Shultz SD, Leitch JA. 2003. The feasibility of restoring previously drained wetlands to reduce flood damage. Journal of Soil and Water Conservation. 58:21–29.
- Smith CS, Puckett B, Gittman RK, Peterson CH. 2018. Living shorelines enhanced the resilience of saltmarshes to Hurricane Matthew (2016). Ecological Applications. 28:871–877. doi:10.1002/eap.1722.
- Stallins JA. 2005. Stability domains in barrier island dune systems. Ecological Complexity. 2:410–430. doi:10.1016/j.ecocom.2005.04.011.
- StatsNZ. 2018. Wetland extent – published April 2018. https://www.stats.govt.nz/indicators/wetland-extent/.
- StatsNZ. 2019. Canterbury lamb gives way to dairy. https://www.stats.govt.nz/news/canterbury-lamb-gives-way-to-dairy.
- Stutter M, Baggaley N, Ó hUallacháin D, Wang C. 2021. The utility of spatial data to delineate river riparian functions and management zones: a review. Science of the Total Environment. 757:143982. doi:10.1016/j.scitotenv.2020.143982.
- Tau TM. 2000. Ngāi Tahu and the Canterbury landscape – a broad context. In: J Cookson, G Dunstall, editors. Southern capital, Christchurch: towards a cirty biography, 1850–2000. Christchurch: Canterbury University Press; p. 41–59.
- Taylor EB, Gibeaut JC, Yoskowitz DW, Starek MJ. 2015. Assessment and monetary valuation of the storm protection function of beaches and foredunes on the Texas coast. Journal of Coastal Research. 315:1205–1216, 1212. doi:10.2112/JCOASTRES-D-14-00133.1.
- Te Rūnanga O Ngāi Tahu Act 1996. Schedule 1.
- Thorslund J, Jarsjo J, Jaramillo F, Jawitz JW, Manzoni S, Basu NB, Chalov SR, Cohen MJ, Creed IF, Goldenberg R, et al. 2017. Wetlands as large-scale nature-based solutions: status and challenges for research, engineering and management. Ecological Engineering. 108:489–497. doi:10.1016/j.ecoleng.2017.07.012.
- Tonkin JD, Merritt DM, Olden JD, Reynolds LV, Lytle DA. 2018. Flow regime alteration degrades ecological networks in riparian ecosystems. Nature Ecology & Evolution. 2:86–93. doi:10.1038/s41559-017-0379-0.
- Tonkin + Taylor. 2021. Coastal hazard assessment for Christchurch district. Technical report prepared for Christchurch City Council – published September 2021. https://resources.ccc.govt.nz/assets/environment/land/coastalhazards/2021-09-29_CHA_Tech_Report.pdf.
- Van Coppenolle R, Schwarz C, Temmerman S. 2018. Contribution of mangroves and salt marshes to nature-based mitigation of coastal flood risks in major deltas of the world. Estuaries and Coasts. 41:1699–1711. doi:10.1007/s12237-018-0394-7.
- Van Loon AF. 2015. Hydrological drought explained. WIREs Water. 2:359–392. doi:10.1002/wat2.1085.
- van Zanten BT, van Beukering PJH, Wagtendonk AJ. 2014. Coastal protection by coral reefs: a framework for spatial assessment and economic valuation. Ocean & Coastal Management. 96:94–103. doi:10.1016/j.ocecoaman.2014.05.001.
- Waitangi Tribunal. 1991. The Ngai Tahu report (Report No. Wai 27). Wellington: GP Publications.
- Walsh CJ, Roy AH, Feminella JW, Cottingham PD, Groffman PM, Morgan RP. 2005. The urban stream syndrome: current knowledge and the search for a cure. Journal of the North American Benthological Society. 24:706–723. doi:10.1899/04-028.1.
- Wamsley TV, Waters JP, King DB. 2011. Performance of experimental low volume beach fill and clay core dune shore protection project. Journal of Coastal Research. 59:202–210, 209. doi:10.2112/SI59-021.1.
- Wang Y, Huang J, Chen X. 2019. Do forests relieve crop thirst in the face of drought? Empirical evidence from South China. Global Environmental Change. 55:105–114. doi:10.1016/j.gloenvcha.2019.01.008.
- Wunder S, Calkin DE, Charlton V, Feder S, Martínez de Arano I, Moore P, Rodríguez y Silva F, Tacconi L, Vega-García C. 2021. Resilient landscapes to prevent catastrophic forest fires: socioeconomic insights towards a new paradigm. Forest Policy and Economics. 128:102458. doi:10.1016/j.forpol.2021.102458.
- Zedler JB. 2003. Wetlands at your service: reducing impacts of agriculture at the watershed scale. Frontiers in Ecology and the Environment. 1:65–72. doi:10.1890/1540-9295(2003)001[0065:WAYSRI]2.0.CO;2.
- Zedler JB, Kercher S. 2005. Wetland resources: status, trends, ecosystem services, and restorability. Annual Review of Environment and Resources. 30:39–74. doi:10.1146/annurev.energy.30.050504.144248.