Abstract
The fruiting body pattern is an important agronomic trait of the edible fungus Auricularia auricula-judae, and an important breeding target. There are two types of fruiting body pattern: the cluster type and the chrysanthemum type. We identified the fruiting body pattern of 26 test strains, and then constructed two different near-isogenic pools. Then, we developed sequence characterized amplified region (SCAR) molecular markers associated with the fruiting body pattern based on sequence-related amplified polymorphism (SRAP) markers. Ten different bands (189–522 bp) were amplified using 153 pairs of SRAP primers. The SCAR marker “SCL-18” consisted of a single 522-bp band amplified from the cluster-type strains, but not the chrysanthemum strains. This SCAR marker was closely associated with the cluster-type fruiting body trait of A. auricula-judae. These results lay the foundation for further research to locate and clone genes controlling the fruiting body pattern of A. auricula-judae.
1. Introduction
Auricularia auricula-judae is a wood rot fungus that is commonly known in China as black wood ear, based on its morphology [Citation1]. This fungus has been cultivated for at least 2100 years. With the continuing improvements in cultivation systems and technologies, A. auricula-judae has become the second most popular edible fungus in China. More than 98% of the global output of A. auricula-judae is produced in China [Citation2].
The fruiting body pattern of A. auricula-judae is an important agronomic trait. It is also an important breeding target and visual index in the breeding of A. auricula-judae. Our research team at the A. auricula-judae Breeding Station of the China Agriculture Research System has explored and summarized the agronomic traits of A. auricula-judae through studying a large number of strains over many years. These studies clarified that there are different types and times of fruiting. The two fruiting body patterns are the cluster type (CL) and the chrysanthemum type (CH), and the fruiting body character is important in the quantitative taxonomy this species. The CL strains produce a fruiting body with a veined segmented appearance on the upper surface and a wrinkled appearance on the reverse side, while the CH strains produce a fruiting body with undifferentiated organization at the base [Citation3]. Two groups separated at the largest genetic distance in an esterase isozyme analysis; one group consisted of CL strains, the other group consisted of CH strains [Citation4]. The clustering results of A. auricula-judae germplasm resources have been used to match simple sequence repeat (SSR) markers with the results of the esterase isozyme analysis [Citation5].
At present, the CL strains are easily induced to produce fruiting bodies by punching a small hole in the surface of existing fruiting bodies. This method allows producers to meet the consumer market and requires minimal labor input. To propagate the CH strains, however, the ear must be torn and the ear base discarded. This results in a low production rate, produces waste products, and requires substantial labor input. Therefore, the CL varieties have been bred to improve the single-chip ear rate in cultivation. These varieties can meet the market demand and are suitable for industrial-scale development. Chen et al. conducted an isozyme analysis, and found that the CL strains had seven stable esterase isoenzyme bands. This information was used to develop an innovative breeding model for the CL varieties [Citation6]. A directional breeding model was used to select several new CL varieties [Citation7,Citation8]. However, for accurate esterase isoenzyme analyses, the samples must be cultivated under same growth period and environmental conditions.
Sequence characterized amplified region (SCAR) markers are produced from polymorphic regions that differ in size among samples. Essentially, a SCAR marker is a more stable co-dominant molecular marker than other molecular markers (random amplified polymorphic DNA, RAPD; sequence-related amplified polymorphism, SRAP; and amplified fragment-length polymorphism, AFLP). Generally, SCAR markers are detected as bands on a gel after a rapid and simple amplification procedure [Citation9]. Researchers have used SCAR markers to identify and select resistant or susceptible plants, and for molecular marker-assisted selection breeding [Citation10,Citation11]. Some recent studies have reported the use of SCAR markers for edible fungi. For example, they have been used in studies on the color of the fruiting bodies of Agaricus bisporus [Citation12], Flammulina velutipes [Citation13] and Hypsizygus marmoreus [Citation14], the degeneration of Volvariella volvacea strains [Citation15] and F. velutipes [Citation16], mushroom disease in Auricularia polytricha [Citation17], and the mating types of edible fungi [Citation18,Citation19]. These markers have also been used to screen for various traits during breeding. However, molecular markers related to the agronomic traits of A. auricula-judae have not yet been reported.
In this study, specific polymorphic fragments were amplified using many SRAP marker primers, and then transformed into a single, stable SCAR marker, which differentiated between CL and CH strains at the DNA level. This provides an effective method for molecular marker-assisted breeding for the fruiting body pattern in A. auricula-judae.
2. Materials and methods
2.1. Strains and culture conditions
All strains were obtained from the A. auricula-judae Breeding Station of the China Agriculture Research System. Information for all strains used is summarized in . B1–B20 are nationally accredited varieties commonly cultivated in China. These verified strains were used to test the SCAR markers developed in this study. The fruiting body patterns of all strains were confirmed by a fruiting test [Citation4]. The strains were cultivated on a substrate consisting of 78% hardwood sawdust, 20% wheat bran, 1% gypsum, and 1% lime; with 65% relative humidity. The conditions for mycelium incubation and fruiting followed conventional cultivation practices for A. auricula-judae [Citation1].
Table 1. Parent strains of isogenic lines and verified strains.
2.2. Development of isogenic lines
The schematic representation of experiment method is shown in . The F1 strain was generated by crossing A14-5 and A18-119. The monokaryotic strains, which were isolated from mature fruiting bodies of the F1 strain, were identified by a lack of clamp connections as observed under an optical microscope [Citation20] and named of 119 × 5-X. The monokaryotic strains were crossed with A184-57. The hybrid combinations were identified by the presence of clamp connections and two cell nuclei between two nearby dissepiments, as observed under an optical microscope [Citation20] and named of C X. X was the same number that the monokaryotic strains. All hybrid strains were subjected to a fruiting test. The F1 monokaryotic strains with obvious fruiting body pattern genotypes were designated as isogenic lines.
2.3. Extraction of genomic DNA
Total genomic DNA was extracted from F1 monokaryotic strains by a modified CTAB method [Citation21,Citation22]. The concentration of DNA from each strain was diluted to 50 µg/μL. The CL gene pool was constructed by mixing the DNA of the CL-type monokaryotic strains. The CH gene pool was constructed by mixing the DNA of the CH-type monokaryotic strains.
2.4. SRAP analyses
Nine forward primers and 17 reverse primers for SRAPs were combined two by two for the diversity analysis of the set of fruiting body pattern genotypes () [Citation23,Citation24]. Each amplification was carried out in a 20-μL reaction mixture under the following PCR conditions according to Li et al. [Citation23].
Table 2. List of SRAP primer sequences.
Specific bands associated with the fruiting body pattern were screened, excised, and purified with an AXYGEN DNA Gel Extraction Kit (Axygen, Union City, CA). The extracted DNA was ligated into the pEASY®-T5 zero cloning vector (TransGen Biotech Co., Beijing, China), and the positive clones were screened for DNA sequencing. The DNA was sequenced by a commercial DNA sequencing service (Jinweizhi Biotechnology Co., Ltd., Suzhou, China). Data analysis and sequence alignments were conducted using DNAMAN® version 5.2.9 (Lynnon Bio Soft, San Ramon, CA) and the GenBank database (https://www.ncbi.nlm.nih.gov/).
2.5. Development of SCAR markers
The sequencing results were used to design fruiting body pattern-specific SCAR primers to identify fruiting body patterns in the verified strains shown in . The primers were designed with Primer 3 software [Citation25]. The sequences of primers and annealing temperature are listed in . The PCR amplification conditions were the same as those used for the SRAP analyses.
Table 3. List of SCAR primer sequences.
3. Results
3.1. Construction of isogenic lines of fruiting body pattern
The F1 strain 119 × 5 was generated by crossing A14-5 and A18-119 (). Twenty-six single spore isolates (SSIs) were obtained from mature fruiting bodies of the F1 strain 119 × 5 (). The 26 monokaryons were separately crossed with A184-57. The hybrids were subjected to a fruiting test to determine the genotype of 26 monokaryotic strains, which distinguished 12 as the CL type and 14 as the CH type (, ). The CL-type isogenic line was generated by mixing the DNA of 12 monokaryotic strains carrying the CL-type genotype. The CH-type isogenic line was generated by mixing the DNA of fourteen monokaryotic strains carrying the CH-type genotype. Then, SRAP-PCR was performed using DNA from the isogenic lines as the template.
Table 4. Fruiting body pattern of hybrids.
3.2. Analysis of SRAP markers
In total, 153 pairs of SRAP primers were used to amplify fragments from the CL-type and CH-type isogenic lines. Ten pairs of primers could distinguish the fruiting body pattern of all the monokaryotic strains. The primers combination and specific fragments were list in . Eight PCR-amplified fragments were specific to the CL type and two were specific to the CH type. The ten specific fragments were excised, purified, and ligated into the cloning vector.
Table 5. Sequencing information of target fragments.
After cloning, nine target fragments were transformed successfully and produced a single band, while one target fragment (6 × 6-CL-250) produced more than one band, so it was not sequenced (). The sequences of the target fragments were used to conduct BLAST searches of the GenBank database. No homology was found between the sequenced target fragments and known sequences in the NCBI database (data not shown). Sequence information for the target fragments is shown in .
Figure 2. Test results of positive clones of SRAP fragments. Lanes 1 and 2, plasmid target fragment of 7 × 5-CL-300; lanes 3 and 4, plasmid target fragment of 4 × 12-CL-240; lanes 5 and 6, plasmid target fragment of 1 × 8-CL-510; lanes 7 and 8, plasmid target fragment of 3 × 9-CL-300; lanes 9 and 10, plasmid target fragment of 4 × 9-CL-300; lanes 11 and 12, plasmid target fragment of 3 × 4-CH-250; lanes 13 and 14, plasmid target fragment of 5 × 14-CL-240; lanes 15 and 16, plasmid target fragment of 6 × 4-CL-200; lanes 17 and 18, plasmid target fragment of 6 × 6-CL-250; lanes 19 and 20, plasmid target fragment of 1 × 6-CH-450; M: 2,000-bp ladder marker.
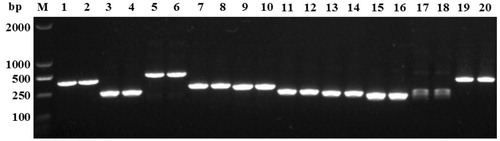
3.3. Analysis of SCAR markers
Based on the sequences of fragments obtained using SRAP primers, SCAR primers were designed using Primer 3 (). These primers were used to amplify fragments from the 20 verified strains shown in . Only one marker was amplified as a single 522-bp band from eight verified strains (): B1, B3, B6, B9, B13, B16, B18, and B20, which were CL-type strains. Therefore, this marker was specific to CL-type strains. The SCAR marker was named SCL-18. The other primers did not produce fruiting body-specific markers that effectively distinguished the fruiting body patterns. The SCAR marker SCL-18 was specific to the CL strains and could accurately distinguish the fruiting body patterns in A. auricula-judae.
Figure 3. Verification of SCAR marker SCL-18. Lanes 1–20 indicate verified strains (B1–B20) of nationally accredited varieties in China (). Single 522-bp band was amplified by SCL-18 primer in lane 1, 3, 6, 9, 13, 16, 18, and 20, corresponding to strains with cluster-type fruiting body pattern (). M: 1500-bp ladder marker.
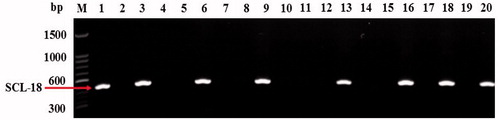
4. Discussion
Whereas higher plants have a complex structure, edible fungi are lower organisms with a simple pattern of differentiation. The agronomic traits of A. auricula-judae (Auriculariales) differ from those of other common edible fungi [Citation2]. Its agronomic traits are not as stable or easily defined as those of other edible fungi; for example, the color of the fruiting body of A. bisporus [Citation12] and the sporeless fruiting body of Pleurotus ostreatus [Citation26]. This has resulted in slow progress of A. auricula-judae breeding. Our research group has explored the fruiting body pattern of A. auricula-judae in many strains cultivated over a long period [Citation20,Citation27]. The fruiting body pattern can be classified as the CL type or the CH type [Citation3]. The CL strains are more easily cultivated than the CH strains, and so they have been the main target of breeding [Citation28].
In this study, the SCAR marker SCL-18 related to the CL type was successfully screened from hundreds of pairs of SRAP primers. Compared with protein bands or isozyme markers, DNA markers are more conservative and less susceptible to environmental effects [Citation29]. A DNA marker allows for the screening of strains during the hyphal growth period, which reduces the workload in selecting parents or hybrids and improves breeding efficiency [Citation19]. A reliable molecular marker is a powerful tool for edible fungi breeding. In the future, we intend to study the genetic characteristics of CL strains, combining transcriptome and specific esterase isoenzyme data.
Recent research on the fruiting body color of A. bisporus resulted in the development of two SCAR markers linked to white and brown fruiting bodies [Citation12]. The white fruiting body was found to be a recessive trait controlled by the PPC1 gene [Citation30], which is closely linked to resistance to brown spot disease [Citation31]. These results strongly suggested that PPC1 may be related to disease resistance. The sequences of the specific fragments obtained in this study were used as BLASTN queries to search the GenBank database, but there were no homologous sequences in the database. In future research, we will try to locate the trait loci on the SSR genetic linkage map of A. auricula-judae. This will allow us to explore genetic regulation mechanisms and genes of interest through the linkage relationships with other traits and/or molecular markers.
The first SCAR marker was developed from RAPD molecular markers to select genes involved in downy mildew resistance in lettuce [Citation32]. Other molecular markers such as SRAP and AFLP can also be transformed into SCAR markers. The RAPD markers have low requirements for primer design, high sensitivity, and low cost, but they are poorly reproducible, which affects the accuracy of the results. The AFLP markers are polymorphic and reproducible, but their disadvantages include the complex technology required, cumbersome operation steps, and relatively high labor costs. The SRAP markers are highly stable and polymorphic, but the conversion rate is low [Citation30]. In this study, SRAP markers were used to screen the polymorphisms related to fruiting body traits. From the successful SRAP markers, we generate a stable 522-bp SCAR marker designated as SCL-18. It is a highly stable and accurate SCAR marker. This provides an effective method to identify the CL strains of A. auricula-judae at an early growth stage. We describe an efficient and rapid identification method that can be used to select breeding parents and hybrids during breeding.
Acknowledgements
We thank Jennifer Smith, PhD, from Liwen Bianji, Edanz Group China (www.liwenbianji.cn/ac), for editing the English text of a draft of this manuscript.
Disclosure statement
No potential conflict of interest was reported by the authors.
Additional information
Funding
References
- Yao FJ, Bian YB. Graphic illustration on key cultivation techniques of Auricularia auricular-judae. Beijing: China Agricultural Press; 2011.
- Yao FJ, Zhang YM, Lu LX, et al. Research progress on genetics and breeding of Auricularia auricula-judae. J Fungal Res. 2015;13:125–128.
- Chen Y, Yao FJ, Zhang YM, et al. Numerical classification of cultivated germplasm of Auricularia auricular-judae. Mycosystema. 2014;33:984–996.
- Chen Y, Yao FJ, Zhang YM, Fang M. Study on esterase isozyme of cultivated germplasm on Auricularia auricula-judae (Bull.) Quél. In: The 10th National Academic Symposium on Edible Fungi; 2014 Mar 21–23; Beijing, China. Beijing: Mycological society of China; 2014. p. 75–80.
- Wang P, Yao FJ. Studies on germplasm resources evaluation by SSR on Auricularia auricular-judae. In: The 11th Academic Symposium on Medicinal Fungi; 2016 Oct 27–30; Chengdu, China. Beijing: China edible fungi association; 2016.
- Chen Y. Studies on numerical classification of germplasm, directional breeding model construction and differential gene expression analysis of degeneration strain by cDNA-AFLP on Auricularia auricular-judae [dissertation]. Changchun: Jilin Agricultural University; 2014.
- Chen Y, Yao FJ, Zhang YM, et al. A new Auricularia auricula cultivar ‘Jihei 3’. Acta Hortic Sinica. 2014;41:1751–1752.
- Fang M, Yao FJ, Wang XE, et al. A new Auricularia auricula cultivar ‘Jihei 2’. Acta Hortic Sinica. 2013;40:1215–1216.
- Bhagyawant SS. RAPD-SCAR markers: an interface tool for authentication of traits. J Biosci Med. 2016;4:1–9.
- Cunha CMS, Hinz RH, Pereira A, et al. A SCAR marker for identifying susceptibility to Fusarium oxysporum f. sp. Cunense in banana. Sci Hortic. 2015;191:108–112.
- Khan M, Pan Y, Iqbal J. Development of an RAPD-based SCAR marker for smut disease resistance in commercial sugarcane cultivars of Pakistan. Crop Prot. 2017;94:166–172.
- Loftus M, Bouchti KL, Robles C, et al. Use of SCAR marker for cap color in Agaricus bisporus breeding programs. Mush Sci. 2000;15:201–205.
- Kong WS, Kim GH, Kim KH, et al. Molecular marker related to fruitbody color of Flammulina velutipes. Mycobiology. 2004;32:6–10.
- Lee CY, Park JE, Lee J, et al. Development of new strains and related SCAR markers for an edible mushroom, Hypsizygus marmoreus. FEMS Microbiol Lett. 2012;327:54–59.
- Li DQ, Wang J. Screening of molecular markers associated with degeneration of Volvariella volvacea strains. J Northwest A&F Univ (Nat. Sci. Ed). 2015;43:195–201.
- Kim SY, Kim KH, Im CH, et al. Identification of degenerate nuclei and development of a SCAR marker for Flammulina velutipes. PLoS One. 2014;9:e107207.
- Sun J, Bian YB. Slippery scar: a new mushroom disease in Auricularia polytricha. Mycobiology. 2012;40:129–133.
- Li D, Liu Y, Wang P, et al. Development of SCAR markers to determine the mating types of Lepista nuda protoplast monokaryons. Curr Microbiol. 2014;68:536–542.
- Zhao Y, Lin F, Yan SY. Establishment and application of an efficient cross breeding method assisted by molecular markers of Volvariella volvacea. Microbiology China. 2015;42:1165–1174.
- Liu GJ. Study on incompatible factor and breeding of Auricularia auricular [dissertation]. Changchun: Jilin Agricultural University; 2011.
- Murray MG, Thompson WF. Rapid isolation of high molecular weight plant DNA. Nucleic Acids Res. 1980;8:4321–4326.
- Wang XE. Analysis of Genome and lignocellulose degradation genes and new cultivar breeding of Auricularia auricular [dissertation]. Changchun: Jilin Agricultural University; 2013.
- Li G, Quiros CF. Sequence-related amplified polymorphism (SRAP), a new marker system based on a simple PCR reaction: its application to mapping and gene tagging in Brassica. Theor Appl Genet. 2001;103:455–461.
- Lin ZX, Zhang XL, Nie YC, et al. Construction SRAP genetic linkage map in cotton. Chin Sci Bullet. 2003; 15:1676–1679.
- Rozen S, Skaletsky H. Primer3 on the WWW for general users and for biologist programmers. Methods Mol Biol. 2000;132:365–386.
- Baars JJP, Sonnenberg ASM, Mikosch TSP, et al. Development of a sporeless strain of oyster mushroom Pleurotus ostreatus. Indian J Pharm Sci. 2000;74:588–591.
- Chen Y. Study on the diversity of cultured Auricularia auricular germplasm resources and the establishment of core collection [dissertation]. Changchun: Jilin Agricultural University; 2010.
- Wan JN. Study on effect and mechanism of small hole-fruiting method on quality of Auricularia auricular [dissertation]. Changchun: Jilin Agricultural University; 2009.
- Chang ST, Lin FC. Genetics and breedings of edible mushroom. Beijing: China Agriculture Press; 1997.
- Callac P, Moquet F, Imbernon M, et al. Evidence for PPC1 a determinant of the pilei-pellis color of Agaricus bisporus fruiting bodies. Fungal Genet Biol. 1998;23:181–188.
- Moquet F, Desmerger C, Mamoun M, et al. A quantitative trait locus of Agaricus bisporus resistance to Pseudomonas tolaasii is closely linked to natural cap color. Fungal Genet Biol. 1999;28:34–42.
- Paran I, Michelmore RW. Development of reliable PCR-based markers linked to downy mildew resistance genes in lettuce. Theoret Appl Genetics. 1993;85:985–993.