Abstract
Improper disposal of herb residues in China has caused severe problems to the surrounding environment and human safety. Three herb residues, i.e., compound Kushen injection residues (CKI) and part one and part two of Qizhitongluo Capsule residues (QC1 and QC2, respectively), were used for the cultivation of Pleurotus ostreatus. The effect of the supplementation of corncobs (CC) with different herb residues on yield, nutritional composition, and antioxidant activity of P. ostreatus was investigated. Compared to the control, the higher mycelial growth rate was observed on substrates CC +30% CKI and CC +30% QC1, while the higher yield was obtained from substrates CC +30% QC2 and CC +30% CKI. Moreover, chemical analysis of fruit bodies revealed that the addition of herb residues to CC significantly increased proteins, amino acids, ashes, minerals (Na and Ca), and total phenolic contents but significantly reduced carbohydrates and IC50 values of DPPH radicals. In addition, no heavy metals (Pb, Cd, and As) were detected in the fruiting bodies harvested from different substrate combinations. These results demonstrated that mixtures of CC with herb residues might be utilized as a novel, practical, and easily available substrate for the cultivation of P. ostreatus, which is beneficial for the effective management of herb residues.
1. Introduction
In recent years, edible mushrooms cultivation has increased worldwide due to their cooking value and therapeutic benefits [Citation1]. In the world, Pleurotus spp. mushrooms are ranked second in commercially cultivated mushrooms, with China alone producing 8 million tons annually [Citation2,Citation3]. Such widespread cultivation is not only because its well-developed enzyme system can degrade the complex components of biological waste but also because it requires few infrastructures for commercial production [Citation2,Citation4]. The production of Pleurotus spp. has been reported to exploit a wide range of agro-industrial by-products as substrates [Citation5,Citation6]. Adaptation of the genus Pleurotus to new wastes can provide a major method of converting lignocellulosic wastes into edible products with high nutritional value [Citation7]. The substrate used for mushroom production in a region is dependent on locally available agricultural waste and production efficiency [Citation8].
China is most likely the largest consumer and producer of traditional Chinese medicine (TCM) worldwide. Consequently, approximately over 30 million tons of herb residues were discharged each year after the active ingredients were extracted from raw materials [Citation9]. Most of the spent materials were disposed of by stacking in an open, sanitary burial, or burning, thereby causing serious problems to the surrounding environment [Citation9–11]. However, herb residues are a type of large and easily collected biomass resource, and approximately 30–50% of the medicinal active substances still remain in them [Citation9]. Therefore, it is an important issue to make full use of the herb residues in an environmentally friendly manner. For this reason, several attempts, such as pyrolysis, composting, vermicomposting, feedstuff, and biosorbent, have been proposed in previous reports [Citation11]. However, there is still no effective method to exploit herb residues on an industrial scale to achieve the clean production of TCM [Citation12]. Edible mushroom cultivation is an economically viable, environmentally friendly method for the disposal of agro-industrial wastes, along with biotransformation into protein-rich foods [Citation13,Citation14]. Some Chinese scholars have attempted to use herb residues as substrate ingredients for the cultivation of various edible mushrooms or medicinal fungi. However, there are still numerous varieties of herb residues that have not been involved. Moreover, herb residues contain various bioactive components, which will be likely to be effectively absorbed by the Pleurotus fruiting bodies [Citation6]. However, to the best of our knowledge, there is little information in the literatures about the nutritional value and therapeutic value of the mushrooms cultivation on herb residues.
Although corncob is a major substrate for the commercial cultivation of Pleurotus mushrooms in Northern China, the status of inexpensive and readily available herb residues indicates that there is a need to develop new bioconversion schemes that are less costly and more productive. Therefore, three different herb residues were collected from a local pharmaceutical factory and used to evaluate the suitability of herb residues to partially replace corncobs (CC) for the production of P. ostreatus. Moreover, determining the nutritive content and physiologically bioactive compounds in oyster mushrooms could enhance their therapeutic value [Citation2]. Accordingly, we assessed the nutritional composition and antioxidant activity of P. ostreatus grown on corncob substrate supplemented with different herb residues. In addition, in view of the accumulation of heavy metals in edible mushrooms, which may pose a health risk to consumers [Citation15], the contents of heavy metals in P. ostreatus mushrooms were also determined.
2. Materials and methods
2.1. Microorganism and spawn preparation
Pleurotus ostreatus strain was obtained from the Mushroom Engineering Center of Shanxi Agricultural University (Jinzhong City, Shanxi, China). Grain spawn was prepared in autoclavable polypropylene bags filled with boiled wheat grains, 0.5% calcium carbonate, and 1% rice bran (w/w, in terms of dry weight). The bags were then sterilized at 121 °C for 80 min before inoculation with mycelium agar plugs (1 cm in diam.). The grain spawn was incubated at 25 °C and 70% relative humidity until the substrate was fully colonized.
2.2. Substrate preparation
Three different herb residues tested in this study were obtained from the production of two types of Chinese patent drugs in a local pharmaceutical factory. Compound Kushen injection residues (CKI) contained Kushen (Radix Sophorae flavescentis) and Tufuling (Rhizoma smilacis glabrae). Qizhitongluo Capsule residues were divided into two parts. Part one (QC1) consisted of Huangqi (Radix astragali) and Renshen (Radix ginseng), while part two (QC2) consisted of Maidong (Radix Ophiopogonis), Wuweizi (Fructus Schisandrae chinensis), Danggui (Radix angelicae sinensis), Chuanxiong (Rhizoma chuanxiong), Chishao (Radix Paeoniae Rubra), Maodongqing (Ilex pubescens), Jixueteng (Caulis spatholobi), Danshen (Radix Salviae Miltiorrhizae), Zhiheshouwu (Radix Polygoni Multiflori Preparata), Honghua (Flos Carthami), Zelan (Herba Lycopi), DiLong (Pheretima), Yujin (Radix Curcumae), Jianghuang (Rhizoma Curcumae Longae), Tianma (Rhizoma Gastrodiae), Qianghuo (Rhizoma et Radix Notopterygii), and Zhuyazao (Fructus Gleditsiae Abnormalis).
The individual herb residues were dried under the sun to about 15% of the water content and then mixed with the CC at a ratio of 3:5 (w/w). The obtained mixtures were ground into pieces (1.0–2.0 cm) and soaked separately in water overnight, and the surplus water was then drained before blending with 16% (w/w) millet bran and 4% (w/w) calcium carbonate. The control substrate formulation consisted of 80% (w/w) corncob, 16% (w/w) millet bran, and 4% (w/w) calcium carbonate. These ingredients were based on dry weight. Finally, the moisture content of the obtained substrate combination was adjusted to 60% (w/w).
2.3. Mushroom cultivation process
Mushroom cultivation was carried out as previously reported with minor modifications [Citation4]. Briefly, 2.5 kg of the wet substrate (equivalent to 1 kg dry substrate) was filled into autoclavable polypropylene bags (24 × 48 cm size), sterilized at 121 °C for 2 h, and inoculated with 5% (w/w) grain spawn. The mycelial colonization of the substrate was performed at 25 ± 2 °C under dark conditions in a spawn running room. When the bags became white and visible from the transparent polythene sheets, the bags were then cut open. These bags were then transferred to a cropping room, where the temperature and relative humidity was controlled at 20 ± 2 °C and 80–90%, respectively. When the in-rolled margin of the mushroom cap began to flatten, fruiting bodies were harvested in three flushes [Citation13].
2.4. Assessment of cultivation parameters
To evaluate the suitability of the substrate mixtures for P. ostreatus cultivation, the following parameters were assessed as described by Sardar et al. [Citation2]: (a) Mycelial colonization time, defined as the day from inoculation to complete colonization of the substrate by the mycelium; (b) yield, calculated as the sum of fresh weight of the mushrooms harvested in three flushes and expressed in grams per bag; and (c) biological efficiency, calculated as the percentage ratio of fresh fruiting bodies weight over the dry substrate weight.
2.5. Determination of chemical composition
For substrate analysis, samples were dried at 60 °C to a constant weight and then ground into powder using a mill. The carbon (C) and nitrogen (N) contents were determined following the method described by Koutrotsios et al. [Citation4]. The carbon/nitrogen ratio (C/N) of each substrate was then calculated.
Mushroom samples were analyzed for the chemical composition in terms of moisture, crude protein, crude fat, and ash, using the procedures recommended by the Association of Official Analytical Chemists [Citation16]. Moisture content was determined by drying fresh samples until constant weight at 105 °C in a hot air oven. Ash content was determined by incineration at 550 ± 5 °C for 24 h in a muffle furnace. Crude protein content was determined by the Kjeldahl method and was calculated using a conversion factor of 4.38 [Citation13]. Crude fat content was determined by extraction with petroleum ether using a Soxhlet apparatus. Total carbohydrate content was calculated by subtracting the sum of the percentages of crude protein, crude fat, and ash from 100 [Citation17]. Total energy was calculated according to the following equation: Total Energy (kJ) = 17 × (g crude protein + g total carbohydrate) + 37 × (g crude fat) [Citation15]. Reported values were an average of three determinations.
2.6. Determination of amino acid composition
Amino acid analysis of the mushroom samples was performed at the PONY Testing International Group (Beijing, China) according to the Chinese national standard, GB 5009.124-2016 [Citation18]. Briefly, 100 mg of dried fruiting bodies samples was hydrolyzed in screw-capped glass test tubes for 22 h at 110 ± 1 °C using 10 mL of 6 mol/L HCl. The acid hydrolyzate was filtered through filter paper and then evaporated using a tube concentrator at 50 °C under reduced pressure. Subsequently, 1.0 mL sodium citrate buffer solution (pH 2.2) was added to the tube to re-dissolve the dried hydrolyzate. The obtained solution was passed through 0.22 μm filter membrane and used for the determination of amino acids. The sample solution and amino acid standard working solution were separately injected into the amino acid analyzer, and the concentration of amino acids in the sample solution was calculated through the peak area using the external standard method.
2.7. Determination of minerals
Mineral elements analysis was performed as described by Heleno et al. [Citation19] with minor modifications. Briefly, 500 mg of dried mushroom sample underwent a dry-ash mineralization in a muffle furnace at 450 °C. The residue of incineration was extracted with HNO3 (50% v/v) and HCl (50% v/v) and adjusted with distilled water up to 25 mL. The residue sample was then passed through filter paper. A blank digest was prepared in the same manner. Mineral elements analysis was carried out in an atomic absorption spectrophotometer (ZA3000, Hitachi, Japan). The macroelements (K, Na, Ca, and Mg) and microelements (Fe, Zn, Cu, and Mn) were analyzed using flame atomic absorption spectrometry. The heavy metals (Pb, Cd, and As) were analyzed using graphite furnace atomic absorption spectrometry. These results were expressed as mg per kg of dry weight.
2.8. Total phenolic contents assay
Aqueous extracts were prepared following the report described by Liu et al. [Citation20]. Here, 250 g of mushroom powder samples was decocted in 2500 mL water with stirring for 2 h. The aqueous extract was filtered through filter paper and then evaporated under reduced pressure with a rotary evaporator at 50 °C, after which the aqueous extract was lyophilized.
Total phenolic content was determined using the method described by Sardar et al. [Citation2]. Lyophilized samples were dissolved in water to obtain a concentration of 20 mg/mL. Next, 0.5 mL of the extracted sample was added to 1 mL of Folin–Ciocalteu reagent and vortexed thoroughly. Subsequently, saturated sodium carbonate solution (1 mL) was added to the mixture, which was then made up to 10 mL with distilled water. The solution was further incubated under dark conditions for 60 min and the absorbance at 760 nm was then measured. Total phenolic content was determined by a standard curve prepared for gallic acid and expressed as mg of gallic acid equivalents (GAE) per g of dry mushroom.
2.9. Antioxidant activity assay
Antioxidant activity was determined by the method of DPPH radical scavenging ability of the mushroom extracts as described by Liu et al. [Citation20]. Here, 0.1 mL of aqueous extract at different concentrations was mixed with 2.9 mL of 6 × 10−5 mol/L DPPH solution in methanol. The mixture was incubated for 60 min in the dark. The absorbance of the mixture was then measured with a spectrophotometer at 517 nm. DPPH radical scavenging activity was calculated using the following equation: radical scavenging activity (%) = (Acontrol – Asample/Acontrol) × 100, where A is the absorbance. The concentration of extracts required to scavenge 50% of the total DPPH radicals (IC50) was calculated from the graph plotting radical scavenging activity percentage against extracts concentration.
2.10. Statistical analysis
Results were expressed as the mean ± standard deviation. Significant differences among the mean of the individual treatments were assessed using one-way ANOVA with Duncan’s multiple range tests.
3. Results and discussion
3.1. Effects of herb residuals on the cultivation parameters of P. ostreatus
Mycelial colonization time was considerably different among P. ostreatus groups cultivated on different substrate mixtures (). It was noted that the mycelial colonization time ranged between 31 and 42 d and was longer than the values reported by Li et al. in P. ostreatus at 26–29 d [Citation8]. The highest mycelial growth rate was observed on substrate CC +30% CKI, followed by CC +30% QC1 and CC, while substrate CC +30% QC2 showed the longest time for completion of mycelial growth. These results suggested that the addition of QC1 or CKI to substrate could promote mycelial growth and shorten the cultivation cycle. Narain et al. [Citation21] reported the nature of lignocellulosic materials, particularly the C/N ratio, which play a crucial role in mycelial growth of mushroom. The slow rate of spawn running on the CC substrate may be due to its high C/N ratio, whereas slower growth on substrate CC +30% QC2 may be caused by less air permeability as a result of smaller volume for the same dry weight [Citation22].
Table 1. Mycelial colonization time, total yield and biological efficiency of P. ostreatus fruit bodies harvested from corncob substrate supplemented with different herb residuals.
The total yield of P. ostreatus harvested from different substrate mixtures is shown in . Significant differences in the yield of fruiting bodies were obtained by the addition of herb residues to CC substrates. The highest yield (978.00 g) was obtained from substrate CC +30% QC2, followed by CC +30% CKI (931.60 g), and CC (855.80 g), while substrate CC +30% QC1 showed least yield (844.40 g). The obtained values were similar to the reported values of 763–962 g in P. ostreatus described by Li et al. [Citation8], who proposed that the physical properties and C/N ratio of substrates stimulated substrate bioconversion and improved cultivation of oyster mushrooms. In this study, the substrates with lower C/N ratios resulted in higher yield except for QC1-containing substrate, indicating that high nitrogen content in substrates could promote the yield of P. ostreatus mushroom, which was consistent with previous reports [Citation22]. Furthermore, it was interesting to note that CC +30% QC2 substrate with lowest mycelial growth rate produced the maximal yield of fruiting bodies. Similar observations were reported by other studies demonstrating that mycelial growth in the cultivation of P. ostreatus was not consistent with the yield [Citation17]. The maximal yield obtained from substrate CC +30% QC2 might be because the supplement of QC2 increased nutrient available to the fungus and promoted water-holding capacity of the substrate, thereby enhancing the yield of mushroom [Citation14].
Biological efficiency of the substrates reflects the ability of a specific strain to grow on that substrate. In our study, the biological efficiency of P. ostreatus varied between 84.44 and 97.80% (). It showed that the biological efficiency of the substrates supplemented with CKI or QC2 were superior to the control, and the substrates containing QC2 was the best of the three substrate mixtures. These results are consistent with previous reports, which demonstrated that lignocellulosic materials are a good substrate for the cultivation of Pleurotus species [Citation8,Citation14,Citation23]. Moreover, when different lignocellulosic materials were used to cultivate P. ostreatus mushroom, variable biological efficiency has been reported. Li et al. [Citation8] reported that biological efficiency of P. ostreatus cultivated on perilla stalks ranged from 76.33 to 96.17%, whereas Koutrotsios et al. [Citation4] reported that biological efficiency differed considerably by ranging from 18.8 to 137.2% in P. ostreatus cultivated on different lignocellulosic residues. These findings indicated that substrate composition and mushroom genotype might affect the biological efficiency of P. ostreatus. In this study, different herb residues contain different types and amounts of nutrients, among which cellulose, hemicellulose, and nitrogen are the key factors related to optimum growth of P. ostreatus [Citation24]. In addition, in view of the fact that different substrates have a significant effect on the yield of mushrooms, further research on the optimization of cultivation formula is needed to improve the yield of P. ostreatus.
3.2. Effects of herb residuals on the proximate compositions of fruiting bodies
The proximate compositions of P. ostreatus fruit bodies, harvested from mixtures of CC with herb residuals, are shown in . The moisture contents varied between 86.45 and 90.58%, which were consistent with previously reported values [Citation7,Citation13]. The moisture content is dependent on the mushroom species, as well as other parameters relevant to growth, harvest, and storage conditions [Citation5]. The dry matter content is the most important factor when evaluating the nutritional value of mushroom and fresh mushrooms usually containing 5–15% dry matter [Citation25]. In this study, the dry matter content of P. ostreatus fruit bodies ranged from 9.42 to 13.55%. Regarding fat contents, the values varied in the range of 2.34–2.58%, and there were no significant differences between mushrooms from different substrate combinations. The values were greater than that reported of 1.18 and 1.68 g/100 g crude fat for P. ostreatus grown on printed and blank paper substrates, respectively [Citation7], but they did not exceed the limit of 6% indicated by the FDA to comply as a low-fat food [Citation6].
Table 2. Proximate composition of P. ostreatus fruit bodies harvested from corncob substrate supplemented with different herb residuals.
Crude protein content of P. ostreatus fruit bodies varied between 18.35 and 25.68%. The addition of herb residuals to CC significantly increased the contents of crude protein, with a maximum for fruit bodies harvested from substrate CC +30% QC2. The values obtained in this study are similar to the reported values of 20.5–26.1% protein from P. ostreatus cultivated on cotton seed hull mixed with perilla stalk [Citation8] but less than 27.4–34.8% protein reported from P. sajor caju fruit bodies grown on wheat straw supplemented with raw/detoxified mahua cake [Citation13]. According to Koutrotsios et al. [Citation4], the crude protein content was highly variable: 14.64–31.36% for P. ostreatus, which was produced on nine cultivation substrates. It is well known that the protein content of mushrooms varies with the type of substrate, as a result of the differences in nutrient supply [Citation13].
The ash content of P. ostreatus fruit bodies ranged from 7.82 to 9.65%. The values obtained here were similar to the reported values of 6.21–9.86% in P. ostreatus cultivated on nine types of lignocellulosic residues [Citation4] and 6.89–9.70 g/100 g dw in P. ostreatus grown on wheat straw supplemented with sugar beet [Citation26]. The fruiting bodies harvested from CC supplemented with different herb residuals, particularly CKI residues, revealed higher ash content than the control, which might be due to the nature of the cultivation substrate. Mintesnot et al. [Citation27]) reported that the protein and ash contents of mushrooms were closely related to the growth substrates, which were consistent with our results.
Total carbohydrates were observed to be the highest (71.26%) on the control substrate, while the lowest values (62.54%) were obtained from the QC2-containing substrate. The obtained values were higher than that reported of 61.9% for Croatian wild variety of P. ostreatus studied by Beluhan et al. [Citation25], but less than the reported values of 73.2–78.1% in P. ostreatus studied by Fernandes et al. [Citation7]. Koutrotsios et al. [Citation4] reported various content of total carbohydrates in P. ostreatus grown on nine cultivation substrates, ranging from 57% (for substrate combination: almond and walnut shells) to 76% (for pine needles substrate). Based on carbohydrate, protein, and fat contents, the energy values of P. ostreatus fruit bodies were also calculated (). The energy values ranged from 1585.68 to 1618.55 kJ, and the control substrate provided the maximum value for this variable.
3.3. Effects of herb residues on the amino acid composition of fruiting bodies
The amino acid composition in mushrooms grown on different substrate mixtures is shown in . A total of 18 amino acids were detected in the mushrooms in this study. The contents of total amino acids varied between 12.79 and 19.09 g 100 g−1 dw, which was greater than that reported of 67.78 mg/g dw for the Croatian wild variety of P. ostreatus studied by Beluhan et al. [Citation25]. However, this value was less than the reported values of 29.36–34.76 g 100 g−1 dw in P. sajor caju fruiting bodies studied by Gupta et al. [Citation13]. The contents of essential amino acids ranged from 5.26 to 7.81 g 100 g−1 dw, which amounted to 40.92–41.37% of the total amino acids contents. The total amino acid content in fruiting bodies obtained from substrate containing herb residuals was higher than the control substrate. The content of total amino acid was highest in mushrooms cultivated on QC2-containing substrate (19.09 g 100 g−1 dw).
Table 3. Amino acid composition of P. ostreatus fruit bodies harvested from corncob substrate supplemented with different herb residuals (g/100 g dry weight).
Numerous studies have reported that mushrooms are a potential source of essential amino acids [Citation15,Citation20]. The ratios of the essential to nonessential amino acids in this study ranged from 0.69 to 0.71, which is consistent with the reference value of 0.6 recommended by the FAO/WHO [Citation20]. It was observed that the EAA/NEAA value of mushrooms obtained from substrate CC +30% CKI was slightly higher than the other values. Flavor is closely related to consumer acceptance and amino acids are one of the main non-volatile compounds [Citation15]. Among the amino acids, aspartic, and glutamic acid belong to palatable tasting amino acids, while alanine, glycine, serine, and threonine belong to sweet tasting amino acids. Palatable and sweet components might be responsible for the delightful taste of mushrooms [Citation25]. In our study, the palatable and sweet tasting amino acids were obtained in the range of 3.32–4.52 and 2.83–4.32 g 100 g−1 dw, respectively. Beluhan et al. [Citation25] reported that wild P. ostreatus mushroom contained 41.26 mg/g dw palatable tasting amino acids and 13.81 mg/g dw sweet tasting amino acids.
In this study, P. ostreatus revealed an identical amino acid profile containing 18 amino acids for all growth substrates. However, the content of individual amino acid differed among mushrooms harvested from different substrates, which is consistent with previous reports [Citation8,Citation13,Citation22]. This difference may be due to genetic variability in commercial P. ostreatus strains and to the differences in the growth substrates [Citation8]. The content of each identified amino acid was higher in mushrooms harvested from substrate combinations than that in the control substrate, indicating that the supplementation of CC with herb residuals enhanced protein biosynthesis in oyster mushrooms. However, the underlying mechanisms remain to be further elucidated.
3.4. Effects of herb residues on the mineral compositions of fruiting bodies
Minerals are required for metabolic reactions, rigid bone formation, regulation of water and salt balance, sensory stimulation, and so on [Citation28]. Several studies have reported that mushrooms are good sources of mineral elements [Citation29]. In our study, the macroelements Na, K, Mg, and Ca, and the microelements Mn, Fe, Zn, and Cu were determined in P. ostreatus mushrooms as shown in .
Table 4. Mineral composition of P. ostreatus fruit bodies harvested from corncob substrate supplemented with different herb residuals.
The macroelements contents varied in this order: K (19,496–20,388 mg/kg) > Mg (1271–1754 mg/kg) > Na (615–1093 mg/kg) > Ca (391–821 mg/kg). The values obtained here are comparable or greater than the reported values of Na (747.5–887.03 mg/kg), K (17,900–23,409 mg/kg), and Ca (121.59–354.81 mg/kg) for Pleurotus fruiting bodies studied by Sardar et al. [Citation2]. The content of potassium was highest in the samples, which is consistent with the results described by Heleno et al. [Citation29]. The ratio of potassium to sodium (K/Na) in vegetables and fruits is usually >2 [Citation15], whereas the ratios for our mushroom samples varied between 18.17 and 31.66. From a nutritional perspective, high ratios found in P. ostreatus are beneficial to consumers because diets with high K/Na ratios are ideal for patients suffering from hypertension and heart diseases [Citation30]. Regarding microelements, Fe was the microelement detected in higher levels (80.18–129.88 mg/kg), followed by Zn (42.83–52.10 mg/kg), Cu (9.67–14.60 mg/kg), and Mn (3.09–7.44 mg/kg). The values were approximate to those reported of 51–136 mg/kg Fe, 24–55 mg/kg Zn, 13–48 mg/kg Cu, and 2.08–8.50 mg/kg Mn in the P. ostreatus fruiting bodies studied by Li et al. [Citation8]. Fe, Ze, Mn, and Cu, as components of key enzyme complexes, are essential elements in the human body, and thus, our mushroom samples provided a potentially good source of microelements.
Pb, Cd, and As, as the principal toxic metals in food, can lead to progressive toxicity [Citation15]. The Chinese national standard, GB 2762-2017 [Citation31], proposed standard limits for As (5 μg/g dw), Pb (10 μg/g dw), and Cd (2 μg/g dw). In our study, no heavy metals (Pb, Cd, and As) were detected in any of the fruiting bodies harvested from different substrate combinations. The contents of Pb, Cd, and As in our mushroom samples were observed to be at very safe levels, and thus, the mushrooms examined here would not bring health risks to consumers.
The compositions of substrate have significant effects on the accumulation of minerals in mushrooms, and significant differences were found in the uptake of individual elements [Citation8]. In this study, a significant variation was shown among various substrates. It was observed that the maximum uptake and accumulation of various minerals, excluding Zn, was recorded in fruiting bodies grown on substrate CC +30% CKI, which might have been attributed to the increased nutrient supply by the addition of CKI to CC substrate. Furthermore, the contents of Ca obtained from substrates mixtures with herb residues were approximately one time higher than that obtained from the control substrate. These observations were similar to those in previous report by Sardar et al. [Citation2], who found that the differences in minerals contents of P. eryngii mushrooms were closely related to the biological nature and chemical composition of the substrates used for cultivation.
3.5. Effects of herb residues on the total phenolic content and antioxidant activity of P. ostreatus extract
The total phenolic content (TPC) was determined for P. ostreatus cultivated on CC and its mixtures with different herb residues as shown in . There was a significant difference in TPC of P. ostreatus grown on various substrates. The highest values of TPC were shown in fruiting bodies obtained from CKI-containing substrate. The TPC values in this study (1.18–2.27 mg GAE/g of dry mushroom) were higher than the values of 0.145–0.230 mg GAE/g in P. eryngii mushroom cultivated on various agro-industrial residues [Citation2]; however, they were lower than the values of 3.761–4.686 mg GAE/g obtained from P. ostreatus grown on perilla stalks [Citation8]. Srikram et al. [Citation32] reported that edible wild and cultivated mushrooms from Northeast Thailand exhibited a TPC in the range of 9.78–567.65 mg GAE/100 g dw.
Figure 1. Effect of herb residues on the TPC (a) and IC50 value of DPPH (b) of P. ostreatus fruit bodies. Values are expressed as the mean ± standard deviation. Bars with different letters indicate significant differences (p < .05).
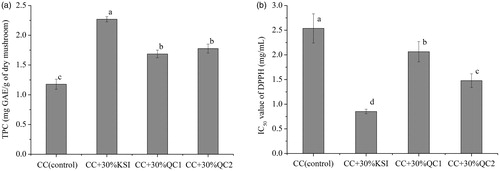
Free radical scavenging is one of the mechanisms involved in inhibiting lipid oxidation and is commonly used to estimate antioxidant activity. The antioxidant activities in the P. ostreatus extracts were evaluated by determining the IC50 values of DPPH radicals (); lower IC50 values represent higher antioxidant activity. The highest IC50 value of these studied mushroom was obtained from the control substrate (2.54 mg/mL), followed by substrates CC +30% QC1 (2.06 mg/mL) and CC +30% QC2 (1.48 mg/mL), while the lowest value was obtained from substrate CC +30% KSI (0.85 mg/mL). This finding suggested that the antioxidant activities were higher for mushrooms grown on substrates containing herb residues than for the control. The IC50 values of the DPPH radical obtained in this study were similar to the values (1.80, 2.65, and 7.30 mg/mL, respectively) reported from three edible mushrooms extracts [Citation33]. Moreover, the differences in the antioxidant properties of mushrooms in our study appeared to be related to the differences in TPC, as phenolics could remove free radicals, inhibit oxidases, reduce α-tocopherol radicals, activate antioxidant enzymes, and chelate metal catalysts [Citation34]. In addition, the mushrooms may contain flavonoids, tocopherols, ascorbic acid, and other bioactive substances, which could also promote the antioxidant activity of mushrooms to a specific extent [Citation15].
The fruiting bodies of Pleurotus respond dramatically to the chemical composition of the substrate where they grow and develop. The bioactive compounds, including PC, flavonoids, alkaloids, tannins, as well as other chemicals with high antioxidant activities, can be effectively absorbed by the fruiting bodies of Pleurotus [Citation6,Citation35]. It was observed that the addition of herb residues to the corncob substrate significantly increased the TPC and antioxidant activity against DPPH radicals. Likewise, it has been reported that the utilization of Agave sisalana wastes for the cultivation of Pleurotus sp. increased the contents of PC, β-carotenes, essential minerals [Citation36], whereas perilla (an edible medical plant) stalks produced P. ostreatus fruiting bodies with high PC and high antioxidant activities [Citation8]. The herb residues contain various functional components, such as polysaccharide, flavonoids, and saponins in Huangqi (Radix Astragali), alkaloids and flavonoids in Kushen (Radix Sophorae Flavescentis), and ginsenosides in Renshen (Radix Ginseng) [Citation37–39]. The accumulation or transformation of these functional components might have contributed to the increase in antioxidant activity of oyster mushrooms. Mushrooms are considered to be a good source of antioxidants, which can protect our body from free radicals [Citation2]. The P. ostreatus extracts obtained from the fruiting body on various substrate combinations revealed a favorable DPPH radical scavenging ability, thereby expanding its healthy value.
Acknowledgments
The authors would like to thank Dr. Hui Zhang from Shanxi Zhendong Group for kindly supplying herb residues.
Disclosure statement
No potential conflict of interest was reported by the authors.
Additional information
Funding
References
- Patel Y, Naraian R, Singh VK. Medicinal properties of Pleurotus species (Oyster mushroom): a review. World J Fungal Plant Biol. 2012;3:1–12.
- Sardar H, Ali MA, Anjum MA, et al. Agro-industrial residues influence mineral elements accumulation and nutritional composition of king oyster mushroom (Pleurotus eryngii). Sci Hortic. 2017;225:327–334.
- Chanakya HN, Malayil S, Vijayalakshmi C. Cultivation of Pleurotus spp. on a combination of anaerobically digested plant material and various agro-residues. Energy Sustain Dev. 2015;27:84–92.
- Koutrotsios G, Mountzouris KC, Chatzipavlidis I, et al. Bioconversion of lignocellulosic residues by Agrocybe cylindracea and Pleurotus ostreatus mushroom fungi – assessment of their effect on the final product and spent substrate properties. Food Chem. 2014;161:127–135.
- Corrêa RCG, Brugnari T, Bracht A, et al. Biotechnological, nutritional and therapeutic uses of Pleurotus spp. (Oyster mushroom) related with its chemical composition: a review on the past decade findings. Trends Food Sci Tech. 2016;50:103–117.
- Carrasco-González JA, Serna-Saldívar SO, Gutiérrez-Uribe JA. Nutritional composition and nutraceutical properties of the Pleurotus fruiting bodies: potencial use as food ingredient. J Food Compos Anal. 2017;58:69–81.
- Fernandes Â, Barros L, Martins A, et al. Nutritional characterisation of Pleurotus ostreatus (Jacq. ex Fr.) P. Kumm. produced using paper scraps as substrate. Food Chem. 2015;169:396–400.
- Li H, Zhang Z, Li M, et al. Yield, size, nutritional value, and antioxidant activity of oyster mushrooms grown on perilla stalks. Saudi J Biol Sci. 2017;24:347–354.
- Meng F, Yang S, Wang X, et al. Reclamation of Chinese herb residues using probiotics and evaluation of their beneficial effect on pathogen infection. J Infect Public Health. 2017;10:749–754.
- Yang J, Qiu K. Development of high surface area mesoporous activated carbons from herb residues. Chem Eng J. 2011;167:148–154.
- Zhao S, Zhou T. Biosorption of methylene blue from wastewater by an extraction residue of Salvia miltiorrhiza Bge. Bioresour Technol. 2016;219:330–337.
- Guo F, Dong Y, Dong L, et al. An innovative example of herb residues recycling by gasification in a fluidized bed. Waste Manage. 2013;33:825–832.
- Gupta A, Sharma S, Saha S, et al. Yield and nutritional content of Pleurotus sajor caju on wheat straw supplemented with raw and detoxified mahua cake. Food Chem. 2013;141:4231–4239.
- Yang W, Guo F, Wan Z. Yield and size of oyster mushroom grown on rice/wheat straw basal substrate supplemented with cotton seed hull. Saudi J Biol Sci. 2013;20:333–338.
- Liu Y, Chen D, You Y, et al. Nutritional composition of boletus mushrooms from Southwest China and their antihyperglycemic and antioxidant activities. Food Chem. 2016;211:83–91.
- Association of Official Analytical Chemists. Official methods of analysis. 16th ed. Arlington (VA): Association of Official Analytical Chemists; 1995.
- Liang C, Wu C, Lu P, et al. Biological efficiency and nutritional value of the culinary-medicinal mushroom Auricularia cultivated on a sawdust basal substrate supplement with different proportions of grass plants. Saudi J Biol Sci. 2016. DOI:10.1016/j.sjbs.2016.10.017
- GB 5009.124 -2016. National food safety standards in food amino acids. Beijing: Standardization Administration of the People’s Republic of China; 2016.
- Heleno SA, Barros L, Martins A, et al. Nutritional value, bioactive compounds, antimicrobial activity and bioaccessibility studies with wild edible mushrooms. LWT Food Sci Technol. 2015c;63:799–806.
- Liu Y, Sun J, Luo Z, et al. Chemical composition of five wild edible mushrooms collected from Southwest China and their antihyperglycemic and antioxidant activity. Food Chem Toxicol. 2012;50:1238–1244.
- Narain R, Sahu RK, Kumar S, et al. Influence of different nitrogen rich supplements during cultivation of Pleurotus florida on maize cobs substrate. Environmentalist. 2009;29:1–7.
- Xu F, Li Z, Liu Y, et al. Evaluation of edible mushroom Oudemansiella canarii cultivation on different lignocellulosic substrates. Saudi J Biol Sci. 2016;23:607–613.
- Liang Z, Wu C, Shieh Z, et al. Utilization of grass plants for cultivation of Pleurotus citrinopileatus. Int Biodeter Biodegr. 2009;63:509–514.
- Chukwurah NF, Eze SC, Chiejina NV, et al. Correlation of stipe length, pileus width and stipe girth of oyster mushroom (Pleurotus ostreatus) grown in different farm substrates. J Agric Biotech Sustain Dev. 2013;5:54–60.
- Beluhan S, Ranogajec A. Chemical composition and non-volatile components of Croatian wild edible mushrooms. Food Chem. 2011;124:1076–1082.
- Manzi P, Gambelli L, Marconi S, et al. Nutrients in edible mushrooms: an inter-species comparative study. Food Chem. 1999;65:477–482.
- Mintesnot B, Ayalew A, Kebede A. Evaluation of biomass of some invasive weed species as substrate for oyster mushroom (Pleurotus spp.) cultivation. Pak J Biol Sci. 2014;17:213–219.
- Inyod T, Sassanarakit S, Payapanon A, et al. Selection of Macrocybe crassa mushroom for commercial production. Agric Nat Resour. 2016;50:186–191.
- Heleno SA, Barros L, Martins A, et al. Chemical composition, antioxidant activity and bioaccessibility studies in phenolic extracts of two Hericium wild edible species. LWT Food Sci Technol. 2015b;63:475–481.
- Alananbeh KM, Bouqellah NA, Al Kaff NS. Cultivation of oyster mushroom Pleurotus ostreatus on date-palm leaves mixed with other agro-wastes in Saudi Arabia. Saudi J Biol Sci. 2014;21:616–625.
- GB 2762-2017. National food safety standards in food contaminants. Beijing: Standardization Administration of the People’s Republic of China; 2017.
- Srikram A, Supapvanich S. Proximate compositions and bioactive compounds of edible wild and cultivated mushrooms from Northeast Thailand. Agric Nat Resour. 2016;50:432–436.
- Heleno SA, Ferreira RC, Antonio AL, et al. Nutritional value, bioactive compounds and antioxidant properties of three edible mushrooms from Poland. Food Biosci. 2015a;11:48–55.
- Amic D, Davidović-Amić D, Bešlo D, et al. Structure-radical scavenging activity relationships of flavonoids. Croat Chem Acta. 2003;76:55–61.
- da Paz MF, Breyer CA, Longhi RF, et al. Determining the basic composition and total phenolic compounds of Pleurotus sajor-caju cultivated in three different substrates by solid state bioprocess. J Biotec Biodivers. 2012;3:11–14.
- Muthangya M, Mshandete AM, Amana MJ, et al. Nutritional and antioxidant analysis of Pleurotus HK 37 grown on Agave sisalana saline solid waste. Int J Res Biochem Biophys. 2014;4:5–12.
- Yang H, Zhou Z, He L, et al. Hepatoprotective and inhibiting HBV effects of polysaccharides from roots of Sophora flavescens. Int J Biol Macromol. 2017;108:744–752.
- Lee JH, Ko MJ, Chung MS. Subcritical water extraction of bioactive components from red ginseng (Panax ginseng C.A. Meyer). J Supercrit Fluid. 2018;133:177–183.
- Wang QH, Han N, Dai N, et al. The structural elucidation and antimicrobial activities of two isoflavane glycosides from Astragalus membranaceus (Fisch) Bge. var. mongholicus (Bge) Hsiao. J Mol Struct. 2014;1076:535–538.