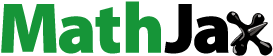
Abstract
Compounds from Lingzhi has been demonstrated the ability for inhibiting tyrosinase (a key enzyme in melanogenesis) activity. In this study, we investigated the anti-melanogenic activity from the submerged mycelial culture of Ganoderma weberianum and elucidated the skin lightening mechanism by B16-F10 murine melanoma cells. From the cellular context, several fractionated mycelium samples exhibited anti-melanogenic activity by reducing more than 40% extracellular melanin content of B16-F10 melanoma cells. In particular, the fractionated chloroform extract (CF-F3) inhibited both secreted and intracellular melanin with the lowest dosage (25 ppm). Further analysis demonstrated that CF-F3 inhibited cellular tyrosinase activity without altering its protein expression. Taken together, our study has demonstrated that the chemical extracts from submerged mycelial culture of G. weberianum have the potential to serve as an alternative anti-melanogenic agent.
1. Introduction
Melanogenesis is a complicated biosynthesis process that involves many cellular factors [Citation1]. The melanocytes are dendritic-like cells that are central to the generation and transport of melanosomes, which are organelles that contain the key enzymes of melanogenesis [Citation2]. The melanosomes are transported from the melanocytes to the neighboring keratinocytes in the epidermis [Citation3]. The natural color of our skin, hair, and eyes are attributed to melanin [Citation4]. However, abnormal pigmentation is observable in several skin disorders, including melanoma, freckles, age spots, and other over pigmentation syndromes [Citation5].
Tyrosinase (EC 1.14.18.1) is an enzyme that catalyzes two rate-limiting reactions in melanogenesis including hydroxylation of L-tyrosine into L-3,4-dihydroxyphenylalanine (L-DOPA) and further oxidation of L-DOPA into dopaquinone, while highly reactive dopaquinone can spontaneously polymerize to form melanin [Citation6]. Since tyrosinase is significant in melanogenesis, tyrosinase inhibition is a common strategy to avoid melanin biosynthesis. Numerous tyrosinase inhibitors from natural or synthetic source have been discovered, and classified into polyphenols, benzaldehyde and benzoate derivatives, long-chain lipids and steroids, other natural or synthetic inhibitors, and irreversible inhibitors [Citation7]. However, few tyrosinase inhibitors are applied in cosmetic and medicinal fields for skin-lightening due to their toxicity or potential hazards [Citation8]. Owing to the safety concerns, it is necessary to find an alternative and natural anti-melanogenic agent without significant side effects [Citation9].
Ganoderma also known as “Lingzhi”, is one of the most important traditional Chinese medicinal mushroom [Citation10]. It has been traditionally used in the prevention and treatment of different human diseases in Asia [Citation11]. However, the potential of using Lingzhi as an alternative form of skin lightening agent has only been recently explored [Citation12]. Extracts of Ganoderma lucidum had exhibited the highest tyrosinase inhibitory activity among Basidiomycetes including Antrodia camphorata, Agaricus brasiliensis and Cordyceps militaris [Citation13]. In addition, several tyrosinase inhibitors including ergosterol peroxide, methyl lucidenate F and ganodermanodiol had been purified and isolated from extract of G. lucidum [Citation6,Citation14,Citation15]. In our previous study, ethyl acetate fraction of G. formosanum mycelium ethanol extract (GFE-EA) demonstrated tyrosinase inhibitory activity in both in vitro cell-free and cellular tyrosinase system, and in vivo zebrafish model [Citation12]. These studies showed the anti-melanogenic activity of Ganoderma and more tyrosinase inhibitors might be found from Ganoderma.
In this study, we explored the possibility of using Lingzhi as a potential skin lightening agent by using mycelial extracts of G. weberianum. The possible inhibitory mechanism of mycelium extract and its cytotoxicity were also evaluated.
2. Materials and methods
2.1. Fungal strain and biomass production of G. weberianum
The strain of G. weberianum (Wu 0711-2, FB) was a gift from the National Museum of Nature Science (Taichung, Taiwan). Eight centimeter square G. weberianum mycelia from agar plate were inoculated into a 500 mL flask containing 200 mL culture medium consisting of glucose (35 g/L), peptone (2.5 g/L), yeast extract (2.5 g/L), KH2PO4·H2O (1 g/L), and MgSO4·7H2O (0.5 g/L) [Citation14], and incubated at 25 °C on a rotary shaker (100 rpm). After 5-days cultivation, 400 mL seed culture was poured into a 6 L stirred-tank bioreactor (Major Science, Wugu, Taiwan) with 4 L culture medium followed by 7-days cultivation at 25 °C under agitation at 100 rpm and aeration rate at 0.5 vvm.
2.2. Isolation of anti-melanogenic extract from G. weberianum
The dried mycelia were obtained by centrifugation at 6500 rpm for 10 min, followed by lyophilization (T10, HCS, New Taipei City, Taiwan). The lyophilized mycelia (15 g) were grounded into powder using a mortar and pestle, and extracted with 95% ethanol. The dry crude extract was obtained by concentrating at 40 °C under reduced pressure using a rotary evaporator (N-1200A; EYELA, Tokyo, Japan). Liquid-liquid partition was carried by dissolving crude extract in deionized water and partitioning with chloroform and ethyl acetate, respectively.
For further purification, gel permeation chromatography was set up by filling Sephadex LH-20 (GE Healthcare, Uppsala, Sweden) into a Pyrex glass column (3 cm diameter and 35 cm long) with degreasing cotton at the bottom. Both chloroform and ethyl acetate partitioned extracts were fractionated by Sephadex LH-20 column eluting with (7:4, v/v) and (1:5, v/v) chloroform and methanol solvent system, respectively.
2.3. In vitro tyrosinase inhibitory assay
Partitioned extract (180 μL) was reacted with 20 μL of cell-free tyrosinase from mushroom (480 units/mL; Sigma, St. Louis, MO) while all reagents were dissolved in 20 mM phosphate buffer. Tyrosinase inhibitory rate was calculated as
while A and B represent the absorbance of vehicle control with and without tyrosinase, respectively; C and D represent the absorbance of the experimental group with and without tyrosinase, respectively [Citation16].
2.4. Cell cultivation and viability assay
The B16-F10 murine melanoma cells (BCRC 60031) were purchased from Bioresource Collection and Research Center (Hsinchu, Taiwan). The human HaCaT keratinocyte was a gift from Dr. Been-Huang Chiang in the Institution of Food Science and Technology National Taiwan University (Taipei, Taiwan). Both cell lines were cultured in Dulbecco’s modified Eagle’s medium with 10% fetal bovine serum (Hyclone, Logan, UT), 50 µg/mL streptomycin, and 50 µg/mL penicillin in a 37 °C incubator with 5% CO2.
The B16-F10 cells were seeded in the 96-well plates at a density of 1 × 104 cells/well. The HaCaT cells were seeded at a density of 3 × 104 cells/well. After 24 h incubation, the culture medium was replaced with fresh culture medium containing different fractionated extracts at various concentrations, followed by further incubation for 48 h. The viability of the treated B16-F10 cells and human HaCaT keratinocytes were determined by using the WST-1 reagent (Roche, Basel, Switzerland). The culture medium in each well was replaced with WST-1 reagent, and then incubated at 37 °C for 30 min. The cell viability was determined at an absorbance of 450 nm using Thermo Multiskan GO (Thermo Scientific, Waltham, MA).
2.5. Measurement of melanin content
The B16-F10 cells were seeded at a density of 1 × 105 cells/well in the 24-well culture plates and incubated at 37 °C with 5% CO2 for 24 h. The B16-F10 cells were treated with different fractionated extracts at various concentrations for 48 h with replacement of culture medium containing drugs at 24 hpt. For secreted melanin content, 100 μL of culture medium was collected and determined at an absorbance of 405 nm against a melanin standard curve. The intracellular melanin content was determined as previous study [Citation17]. After removing the medium, the harvested cells were centrifuged at 3000 × g for 5 min at 4 °C and washed with phosphate-buffered saline. Then, 500 μL of 1 N NaOH with 10% DMSO was added to the cell pellet and incubated at 60 °C for 1 hour to dissolve the melanin. After incubation, the melanin content was determined at an absorbance of 405 nm against a melanin standard curve. The melanin standard curve was prepared by the serial dilution of 20 μg/mL commercial melanin (Sigma).
2.6. Determination of cellular tyrosinase activity
The inhibitory activity of chloroform extracts on in vivo tyrosinase was measured as previously stated [Citation17]. The B16-F10 cells were seeded in 24-well plates and treated with different fractionated chloroform extracts at various concentrations for 48 h. The cells were then harvested using 100 μL of lysis buffer (0.1 M sodium phosphate buffer, pH 6.8, containing 1% Triton X-100 and protease inhibitor). The cells were disrupted by freezing and thawing. The cell lysates were clarified by centrifugation at 14,000 × g for 10 min. The total protein concentration of each cell lysates sample was determined by using Bradford assay (Bio-Rad, Richmond, CA) with bovine serum albumin (BSA) as standard. After normalizing the protein content of each sample, 80 μL of each lysate samples were transferred into a 96-well plate reacted with 20 μL of 5 mM L-DOPA at 37 °C for 1 hour, then tyrosinase activity was determined by measurement of the absorbance at 475 nm.
2.7. Western blot analysis
Treated B16-F10 cells were lysed in ice-cold modified RIPA buffer (50 mM Tris-HCl, pH 8.0, 1% Triton X-100, 0.5% sodium deoxycholate, 0.1% SDS, 150 mM NaCl, complete EDTA-free protease and phosphatase inhibitor). The total proteins were separated by SDS-PAGE and transferred to PVDF membranes. The membranes were incubated with primary antibody, anti-tyrosinase monoclonal antibody (1:1000; Millipore, Billerica, MA), at 4 °C overnight, then washed with Tris-buffered saline containing 0.1% Tween-20 (TBST), followed by incubation in HRP-conjugated anti-mouse IgG secondary antibodies for 1 hour at room temperature, washed with TBST again, and then visualized using an Enhanced Chemiluminescence (ECL).
2.8. Statistical analysis
All experiments were performed in triplicate. All values were expressed as mean ± standard error of the mean. Statistical significance was determined by Student’s t-tests. The minimal level of significance was p < .05 (*), p < .01 (**), or p < .001 (***).
3. Results
3.1. Extracts from submerged culture mycelium of G. weberianum inhibited in vitro mushroom tyrosinase
Tyrosinase inhibitory activity of two partitioned extracts (chloroform and ethyl acetate) was analyzed by the in vitro tyrosinase assay. Chloroform extracts (CF) exhibited tyrosinase inhibitory activity of 41.46 ± 7.0% while ethyl acetate extracts (EA) was 57.52 ± 1.2% ().
Figure 1. The in vitro tyrosinase inhibitory rate on two partitioned fractions from mycelial extract of G. weberianum. Chloroform (CF) and ethyl acetate extracts (EA) obtained from 7-day mycelium cultures (x-axis) were tested for potential inhibitory effects in an in vitro tyrosinase assay. The inhibition rate was plotted as a percentage (y-axis).
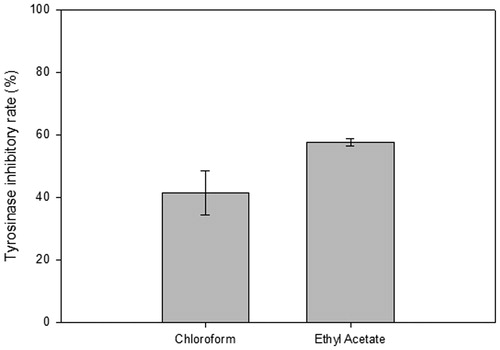
To further purify tyrosinase inhibitor, each extract was subjected to gel permeation chromatography using the Sephadex LH-20 matrix. Both chloroform and the ethyl acetate extracts were further separated into six fractions, named F1–F6. The yield of each fraction was expressed as a percentage by dividing the net weight of each fraction against the weight of the original extract ().
Table 1. The yield of each fractions from the chloroform and ethyl acetate partitioned extracts.
3.2. Fractions from both the chloroform and ethyl acetate extracts reduced the secreted melanin content within B16–F10 cells
To validate the potential anti-melanogenic agent from fractionated extracts, the secreted melanin content from treated B16-F10 cells was evaluated using different concentrations of extracts. At 48 h post-treatment (hpt), the secreted melanin content was determined by measuring the absorbance at 405 nm. After normalization with cell numbers, F3 at 25 ppm and F4 at 100 ppm from chloroform extract reduced the secreted melanin content by 40.90 ± 3.0% and 30.19 ± 0.9%, respectively, whereas all other fractions exhibited insignificant effects on the secreted melanin content (). Fractions from the ethyl acetate extract did not exhibit reduction effect on secreted melanin content, except for F1 and F2 (). As 25 ppm of CF-F3 exhibited similar anti-melanogenic effect with lower dosage compared with 100 ppm of CF-F4, the following in vivo experiments were treated with CF-F3 below 25 ppm.
Figure 2. The secreted melanin content from B16–F10 mouse melanoma cells after treated with chloroform (A) and ethylacetate (B) extracts. Melanin inhibition effect which normalized by cells number was determined at 48 h post-treatment and represented as percentage of control (y-axis) when compared against untreated cells (control).
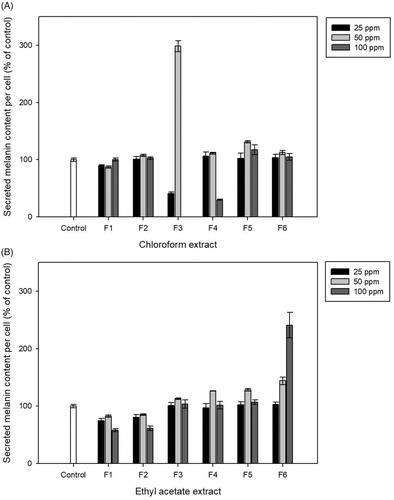
3.3. The CF-F3 exhibited inhibitory effect on the production of both secreted and intracellular melanin
To verify the anti-melanogenic activity of CF-F3, both the secreted melanin and intracellular melanin content of B16-F10 cells were determined. The secreted and intracellular melanin content from 25 ppm of CF-F3 treatments were decreased to 63.38 ± 1.0% and 54.90 ± 7.4%, respectively ().
Figure 3. Anti-melanogenic effect of CF-F3 on B16-F10 melanoma cells at 48 h post-treatment with the replacement of medium at 24 h post-treatment. (A) The secreted melanin content of B16-F10 melanoma cells which were treated with CF-F3 at various concentration (x-axis). Secreted melanin inhibition effect which normalized by cells number was represented as percentage of control (y-axis) when compared against untreated cells (control). (B) The intracellular melanin content of B16–F10 melanoma cells which were treated with CF-F3 at various concentration (x-axis). Intracellular melanin inhibition effect which normalized by cells number was represented as percentage of control (y-axis) when compared against untreated cells (control).
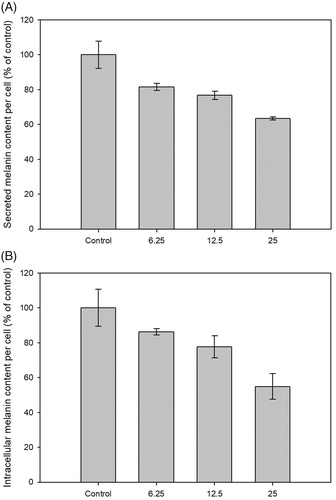
3.4. Evaluation of CF-F3 for cytotoxicity
Cytotoxicity effect of CF-F3 on human keratinocyte HaCaT cells was evaluated. Cells with 48 hpt of CF-F3 at various concentrations were evaluated for cell viability based on the WST-1 cell cytotoxicity assay. As shown in , CF-F3 did not exhibit a cytotoxic effect on HaCaT cells within the concentration of 12.5–50 ppm.
Figure 4. The cytotoxicity analysis of the human HaCaT keratinocyte cells under the treatment of CF-F3. HaCaT human keratinocyte cells were treated with the various concentration of CF-F3, (x-axis). The cytotoxicity effect was determined at 48 h post-treatment and represented as percentage of control (y-axis) when compared against untreated cells (control).
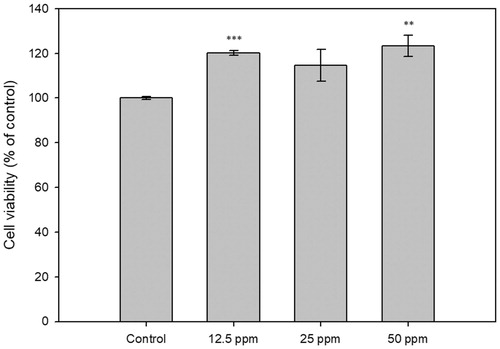
3.5. Mechanism of melanogenesis inhibition of CF-F3
To elucidate the anti-melanogenic mechanism of CF-F3, cellular tyrosinase activity was determined. Tyrosinase activity from 25 ppm CF-F3 treated B16-F10 cells were inhibited up to 70.80 ± 1.0% (). To further investigate whether the tyrosinase inhibitory activity of CF-F3 was accomplished by suppressing the protein level in B16-F10 cells, protein expression of cellular tyrosinase was determined by western blot assay. As shown in , there was no observable difference in the expression of tyrosinase between the untreated and CF-F3 treated B16–F10 cells.
Figure 5. Cellular tyrosinase activity and western blot analysis of the tyrosinase protein expression in CF-F3 treated B16–F10 melanoma cells. (A) The tyrosinase inhibition effects from the various concentrations of CF-F3 (x-axis) were represented as percentage of control (y-axis) when compared against untreated cells (control). (B) B16–F10 melanoma cells at 18, 24, 48 h post-treatment (untreated, 100 nM alpha-melanocyte stimulating hormone (α-MSH), kojic acid (KA), and the CF-F3 fraction) were harvested to extract the total cellular protein for the western blot analysis. As a load control, western blot detection of β-actin was performed.
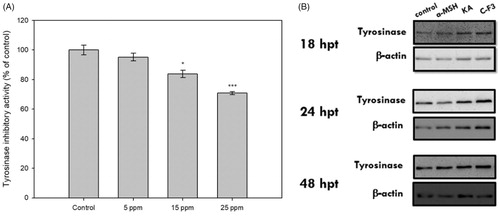
4. Discussion
In 2008, the inhibitory activity of G. lucidum extract on tyrosinase was first evaluated [Citation13]. Ethanolic and aqueous extracts of G. lucidum mycelium exhibited the highest tyrosinase inhibitory rate (IC50 = 320 ppm) among the tested Basidiomycetes (C. militaris, A. brasiliensis, and Antrodia camphorate). In this study, chloroform (CF) and ethyl acetate (EA) extracts of G. weberianum exhibited tyrosinase inhibitory activity of 41.46 ± 7.0% and 57.52 ± 1.2%, respectively. While Inonotus mikadoi (a species of fungi in the family Hymenochaetaceae) among fifty-five mushroom mycelial extracts (10 mg/mL) showed similar tyrosinase inhibitory activity (40%) on in vitro tyrosinase assay [Citation18], CF and EA from G. weberianum mycelia exhibited better inhibitory effect with lower dosage (200 ppm). This result indicated that submerged mycelial extract of G. weberianum may be a potent source to isolate novel compounds as tyrosinase inhibitor.
After further purification of tyrosinase inhibitor by gel permeation chromatography, both CF-F3 at 25 ppm, CF-F4 at 100 ppm, EA-F1 at 100 ppm, and EA-F2 100 ppm extracts significantly reduced the secreted melanin content of treated B16-F10 cells while CF-F3 was selected for the following experiment. In addition, both secreted melanin and intracellular melanin content per cells were dramatically reduced under CF-F3 treatment in a dose-dependent manner. It was obvious that CF-F3 not only reduced the production of melanin, but also inhibited the melanin secretion. Various studies screened tyrosinase inhibitors by cell-free tyrosinase assay without any in vivo experiments, resulting cellular anti-melanogenic effect remains unknown [Citation19,Citation20]. While CF-F3 showed suppression on both extracellular and intracellular melanin production of B16-F10 cells, a well-established cell model, validating its cellular anti-melanogenic activity. Hsu et al. [Citation12], found that 200 ppm of the ethyl acetate fraction of G. formosanum mycelia ethanolic extract (GFE-EA) reduced ∼50% of melanin formation of zebrafish embryos, which provides a possible way for rapid screening of potential skin-lightening compounds using an animal model.
HaCaT cells have been widely used as a cell model for cytotoxicity test because the cytotoxicity test of HaCaT cell gave acute information within a short period of treatment [Citation21]. CF-F3 with concentration lower than 50 ppm did not exhibited cytotoxic effect on HaCaT cells. Besides, the previous study showed nontoxic effect of Ganoderma mycelial chloroform extract on RAW264.7 cells below 300 ppm [Citation22]. Therefore, 50 ppm of CF-F3 was considered as safe on the following in vivo cell models.
Cellular tyrosinase activity was inhibited by 25 ppm CF-F3 without alteration of tyrosinase protein expression. Adlay (Coix lachryma-jobi) extract, a traditional Chinese medicinal plant, also showed about 70% cellular tyrosinase inhibitory activity on B16-F10 cells by 25 ppm dosage while tyrosinase expression was affected by adlay extract [Citation23]. In addition, ergosterol peroxide and ganodermanodiol, tyrosinase inhibitors isolated from G. lucidum, attenuated tyrosinase activity by suppression of tyrosinase-related protein expression [Citation14,Citation15]. All these compounds accomplished anti-melanogenic activity by protein suppression, while potential hazard on suppressing tyrosinase protein expression remains unknown.
We infer that the anti-melanogenic effect of CF-F3 was due to the inhibition of the tyrosinase activity rather than suppression of protein expression, which is similar to the standard skin lightening agents, kojic acid and arbutin [Citation7]. Kojic acid, a tyrosinase inhibitor, is considered as a safe ingredient in cosmetic product with concentration below 2%, while a depigmentation study found that kojic acid exhibited significant skin lightening activity on guinea pig experiment at a concentration of 4% [Citation24]. Nevertheless, previous study had suggested that kojic acid may have tumor promotion and weak carcinogenicity on animal tests [Citation25]. In our previous research, kojic acid had exhibited a negative effect on heart-beating rate in zebrafish model [Citation12]. Even though Taiwan Food and Drug Administration (TFDA) listed kojic acid as a legal ingredient in skin lightening products, it remains a safety concern about using kojic acid for anti-melanogenesis. In this study, CF-F3 exhibited similar anti-melanogenic mechanism to kojic acid by inhibiting tyrosinase activity without suppressing its protein expression. In addition, CF-F3 did not contribute any significant cytotoxicity towards an immortalized human keratinocyte cell line, HaCaT cells, below 50 ppm which was higher than the effective anti-melanogenic concentration 25 ppm.
For development as a skin lightening agent, further researches are needed to compare the anti-melanogenic efficacy in different animal models, for example, phenotype-based zebrafish, and skin penetration evaluation. In summary, this study demonstrated potentiality of CF-F3 as an alternative skin lightening agent.
In conclusion, various tyrosinase inhibitors (i.e., ergosterol peroxide and methyl lucidenate F) have been isolated from Ganoderma spp., showing the potential anti-melanogenic activity of Gandoerma [Citation12,Citation13]. Our study evaluated extracts obtained from the mycelium of submerged culture of G. weberianum, a Ganoderma sp. widely distributed in Taiwan, and further investigated the mechanism of anti-melanogenesis. Results demonstrated that CF-F3 exhibited the most inhibitory effect for melanogenesis at the lowest dosage (25 ppm). Moreover, we validated that the anti-melanogenesis effect of CF-F3 was via the inhibition of the enzymatic activity of tyrosinase, in vivo, without affecting its protein level. Finally, and most importantly, it was determined that CF-F3 did not contribute any significant cytotoxicity towards human keratinocyte HaCaT cells. These findings suggested that G. weberianum and its constituents might be potential candidates for novel skin-lightening agents. Further study aims to identify the major compound(s) for tyrosinase inhibitory activity using a high throughput system is necessary [Citation26]. An animal model study, for example, phenotype-based zebrafish and skin penetration evaluation are suggested [Citation12,Citation27].
Acknowledgments
We would like to thank Dr. Sheng-Hua Wu from National Museum of Nature Science in Taichung for kindly gifting us the G. weberianum strain.
Disclosure statement
No potential conflict of interest was reported by the authors.
Additional information
Funding
References
- D’Mello S, Finlay G, Baguley B, et al. Signaling pathways in melanogenesis. Int J Mol Sci. 2016;17:1144.
- Lee CS, Park M, Han J, et al. Liver X receptor activation inhibits melanogenesis through the acceleration of ERK-mediated MITF degradation. J Invest Dermatol. 2013;133:1063–1071.
- Kim K. Effect of ginseng and ginsenosides on melanogenesis and their mechanism of action. J Ginseng Res. 2015;39:1–6.
- Weiner L, Fu W, Chirico WJ, et al. Skin as a living coloring book: how epithelial cells create patterns of pigmentation. Pigment Cell Melanoma Res. 2014;27:1014–1031.
- Chung KW, Jeong HO, Lee EK, et al. Evaluation of antimelanogenic activity and mechanism of galangin in silico and in vivo. Biol Pharm Bull. 2018;41:73–79.
- Zhang L, Ding ZY, Xu P, et al. Methyl lucidenate F isolated from the ethanol-soluble-acidic components of Ganoderma lucidum is a novel tyrosinase inhibitor. Biotechnol Bioproc E. 2011;16:457–461.
- Chang TS. An updated review of tyrosinase inhibitors. Int J Mol Sci. 2009;10:2440–2475.
- Lee SY, Baek N, Nam T-g. Natural, semisynthetic and synthetic tyrosinase inhibitors. J Enzyme Inhib Med Chem. 2016;31:1–13.
- Campos PM, Horinouchi CDD, Prudente AD, et al. Effect of a Garcinia gardneriana (Planchon and Triana) Zappi hydroalcoholic extract on melanogenesis in B16F10 melanoma cells. J Ethnopharmacol. 2013;148:199–204.
- Pengjiao Z, Zhihua G, Xuan Z, et al. Chemical, biochemical, preclinical and clinical studies of Ganoderma lucidum polysaccharide as an approved drug for treating myopathy and other diseases in China. J Cell Mol Med. 2018;0:3278–3297.
- Pi CC, Chu CL, Lu CY, et al. Polysaccharides from Ganoderma formosanum function as a Th1 adjuvant and stimulate cytotoxic T cell response in vivo. Vaccine. 2014;32:401–408.
- Hsu KD, Chen HJ, Wang CS, et al. Extract of Ganoderma formosanum mycelium as a highly potent tyrosinase inhibitor. Sci Rep. 2016;6:32854.
- Chien CC, Tsai ML, Chen CC, et al. Effects on tyrosinase activity by the extracts of Ganoderma lucidum and related mushrooms. Mycopathologia. 2008;166:117–120.
- Mukaiyama T, Tsujimura N, Otaka S, et al. Anti-melanogenic activity of ergosterol peroxide from Ganoderma lucidum on a mouse melanoma cell line. Animal Cell Technology: Basic & Applied Aspects. Berlin, Germany: Springer; 2008. p. 273–277.
- Kim JW, Kim HI, Kim JH, et al. Effects of ganodermanondiol, a new melanogenesis inhibitor from the medicinal mushroom Ganoderma lucidum. Int J Mol Sci. 2016;17:E1798.
- Likhitwitayawuid K, Sritularak B. A new dimeric stilbene with tyrosinase inhibitiory activity from Artocarpus gomezianus. J Nat Prod. 2001;64:1457–1459.
- Arung ET, Furuta S, Ishikawa H, et al. Anti-melanogenesis properties of quercetin- and its derivative-rich extract from Allium cepa. Food Chem. 2011;124:1024–1028.
- Park KM, Kwon KM, Lee SH. Evaluation of the antioxidant activities and tyrosinase inhibitory property from mycelium culture extracts. Evid-Based Compl Alt. 2015;2015:1.
- Chen XX, Shi Y, Chai WM, et al. Condensed tannins from Ficus virens as tyrosinase inhibitors: structure, inhibitory activity and molecular mechanism. Plos One. 2014;9:e91809.
- Chai WM, Lin MZ, Song FJ, et al. Rifampicin as a novel tyrosinase inhibitor: inhibitory activity and mechanism. Int J Biol Macromol. 2017;102:425–430.
- Boonkaew B, Kempf M, Kimble R, et al. Cytotoxicity testing of silver-containing burn treatments using primary and immortal skin cells. Burns. 2014;40:1562–1569.
- Geng Y, Zhu S, Lu Z, et al. Anti-inflammatory activity of mycelial extracts from medicinal mushrooms. Int J Med Mushrooms 2014;16:319–325.
- Huang HC, Hsieh WY, Niu YL, et al. Inhibitory effects of adlay extract on melanin production and cellular oxygen stress in B16F10 melanoma cells. Int J Mol Sci. 2014;15:16665.
- Burnett CL, Bergfeld WF, Belsito DV, et al. Final report of the safety assessment of kojic acid as used in cosmetics. Int J Toxicol. 2010;29:244s–273s.
- Takizawa T, Imai T, Onose J-i, et al. Enhancement of hepatocarcinogenesis by kojic acid in rat two-stage models after initiation with N-bis (2-hydroxypropyl) nitrosamine or N-diethylnitrosamine. Toxicol Sci. 2004;81:43–49.
- Hsu KD, Chan YH, Chen HJ, et al. Tyrosinase-based TLC autography for anti-melanogenic drug screening. Sci Rep. 2018;8:401.
- Praca FSG, Medina WSG, Eloy JO, et al. Evaluation of critical parameters for in vitro skin permeation and penetration studies using animal skin models. Eur J Pharm Sci. 2018;111:121–132.