Abstract
Three hepatic failure poisoning incidents caused by Lepiota brunneoincarnata and Lepiota venenata mushrooms have been occurred in China in 2017, L. venenata has been described as a new species. However, the cyclopeptide toxins of these lethal mushrooms remain poorly understood. In this study, the composition and content of amatoxins in L. brunneoincarnata and L. venenata are analyzed and compared, the analysis of composition and content of amatoxins in L. venenata are reported for the first time. The results showed that β-amanitin (β-AMA), α-amanitin (α-AMA), amanin, and amaninamide were identified in L. brunneoincarnata, and α-AMA, amanin II (an analog of amanin), and an unknown compound were identified in L. venenata. The differences between L. brunneoincarnata and L. venenata in the identified compounds provide chemical evidence for L. venenata as a new species. Quantitative analysis shows that α-AMA concentrations in L. brunneoincarnata and L. venenata were 0.72–1.97 mg/g dry weight, β-AMA concentrations in L. brunneoincarnata were 0.57–0.94 mg/g dry weight, and β-AMA was absent in L. venenata.
1. Introduction
The genus Lepiota contains approximately 400 species of mushrooms distributed worldwide [Citation1]. It is well-known that several species have caused fatal poisonings after ingestion from Europe, America, and Asia, such as Lepiota brunneoincarnata [Citation2–6], Lepiota helveola [Citation7,Citation8], and Lepiota subincarnata [Citation9]. These poisonous Lepiota species contain amatoxins, which are responsible for the hepatotoxicity by irreversibly binding to RNA polymerase II [Citation1,Citation10]. However, in contrast to Amanita, there have been relatively few analyses of amatoxins composition and content in Lepiota species. In the early years of the last century, the amatoxins detection of Lepiota species have been restricted to the Meixner test, thin layer chromatography, and radioimmunoassay; these methods have poor resolution and low sensitivity [Citation11,Citation12]. Until the last few years, the amatoxins analysis in Lepiota species has been established using modern high-resolution methods in two related literature. Sgambelluri et al. [Citation12] analyzed the composition of amatoxins present in six Italian species of Lepiota using liquid chromatography coupled to UV absorbance and mass spectrometry, and Yilmaz et al. [Citation13] investigated the amatoxins concentration in different parts and in different growth phases of L. brunneoincarnata mushroom collected in Turkey using the reversed phase high-performance liquid chromatography (HPLC) method.
In China, mushroom poisoning caused by Lepiota species has never been reported; however, three mushroom poisoning incidents caused by Lepiota mushrooms occurred in 2017 and we obtained the Lepiota species specimens from the poisoning sites. Among these three poisoning cases, two cases were caused by L. brunneoincarnata, and one cases was caused by Lepiota venenata, which has been described as a new species [Citation14]. The objective of this study was to detect the amatoxins composition and content in L. brunneoincarnata and L. venenata collected from China using HPLC coupled with high-resolution mass spectrometry (HPLC-HRMS).
2. Materials and methods
2.1. Mushroom collection and sample conditions
Three Lepiota samples (L. brunneoincarnata MHHNU 31026, MHHNU 31032, and L. venenata MHHNU31031) were collected from the place where the patients had collected the mushrooms under the leadership of the patient’s relatives and the mushroom was confirmed by the patients that it was the mushroom they ate. L. brunneoincarnata MHHNU 31030 sample was collected from the Labor Park in Daliang, Liaoning. All fresh mushroom carpophores were dried at 50 °C with airflow food dryer (Jintao Electrical Co., LTD, Ningbo, China) for 24 h until completely dry. All samples were deposited in desiccator in the Mycological Herbarium of Hunan Normal University (MHHNU). Information on the collections and their ITS accession numbers in GenBank are given in and .
Figure 1. Basidiomata of Lepiota species. (A) Lepiota brunneoincarnata (MHHNU 31026); (B) L. brunneoincarnata (MHHNU 31030); (C) L. brunneoincarnata (MHHNU 31032); (D) L. venenata (MHHNU 31031; photos A, B, and D by Zuohong Chen; photo C by Xiaolan He).
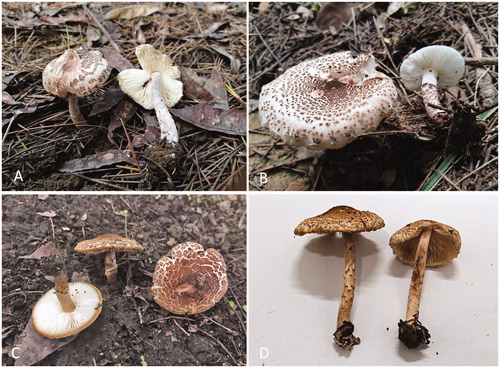
Table 1. List of samples analyzed in this study.
2.2. Mushroom identification and phylogenetic analysis based on ITS sequences
All Lepiota species were identified by both morphological and molecular phylogenetic analyses. The morphological identification was performed according to the macroscopic and microscopic characteristics using our previous methods [Citation14]. DNA extraction, polymerase chain reaction amplification, sequencing, and alignment of the ITS sequences were performed as described by Zhang et al. [Citation15] and Cai et al. [Citation16]. The phylogenetic tree was generated with both maximum likelihood analyses and Bayesian inference was performed using RAxML 7.2.6 [Citation17] and MrBayes 3.2.4 [Citation18], respectively, with the parameters employed by Cai et al. [Citation16], except that the Bayesian Inference runs were performed for 10 million generations.
2.3. Extraction of the amatoxins
The extraction of amatoxins was performed by our previous described method [Citation19,Citation20]. Pieces of the pileus from the dried carpophores of the samples were cut and dried at 45 °C with airflow food dryer to a constant weight, then ground using a KC-04 pulverizer. For each sample, 0.05 g ground material was weighed and dispensed accurately into individual test tubes, and the peptide toxins were extracted with 2 mL formic acid/methanol/water (0.5:50:49.5, v/v/v). The test tubes were then shaken at 200 rpm for 12 h. Following centrifugation at 14,000 rpm for 10 min, the supernatant was decanted and retained while the residue was extracted again as described above. The two supernatants were combined and evaporated, and the residue was dissolved in 1 mL of water. This solution was degreased in petroleum ether, evaporated again, and the residue was redissolved in 100 μL of 10% methanol in water for HPLC analysis. The extraction was performed in triplicate for each sample, and the mean value was determined.
2.4. HPLC-HRMS analysis
HPLC conditions: Waters Acquity UPLC (Waters, Milford, MA, USA) coupled with a variable wavelength detector, CAPCELL PAK C18 (2.1 mm × 100 mm, 2.7 µm), F3643 EXP® GUARD CARTRIDGE CAPCELL CORE C18 S-2.7 (2.1 × 5 mm, 2.7 µm); mobile phase: (A) 0.01 M aqueous ammonium acetate; (B) methanol. Gradient elution: 0–15 min, 0–5% B; 15–35 min, 5–80% B; 35–40 min, 80% B; 40–45 min, 80%–100% B; 45–50 min, 100% B; 50–55 min, 100%–0 B; 55–60 min, 100% A; flow rate: 0.25 mL/min; injection volume: 10 µL; column temperature: 40 °C. The absorbance of the eluates was monitored at 295 nm.
HRMS conditions: Waters Xevo G2-quadrupole time-of-flight (QTOF) MS/MS in electrospray positive ionization mode; source temperature: 100 °C; full scan acquisition mode; scan range: m/z 50–1000; capillary voltage: 3 kV; sample cone voltage: 30 V; extraction cone voltage: 4 V; cone flow: 50 L/h; desolvation temperature: 300 °C; desolvation gas: 600 L/h; mass calibration: lockspray with 556.2771 Da of leucine encephalin.
2.5. Standard samples, linear regression equation, and quantitative analysis of α-AMA and β-AMA
Standard samples of α-amanitin (α-AMA, 90% purity, purchased from Merck-Calbiochem, Merck KGaA, Darmstadt, Germany) and β-amanitin (β-AMA, 90% purity, Sigma-Aldrich, St. Louis, MO, USA) were dissolved in doubly distilled water to a concentration of 0.1 mg mL−1 stock solution. Each of 50 μL α-AMA and β-AMA was taken and mixed in a tube and a concentration of 0.05 mg mL−1 α-AMA and β-AMA solution were obtained. A five-point curve was achieved with injections of 2, 4, 6, 8, and 10 μL (corresponding to 0.09, 0.18, 0.27, 0.36, and 0.45 μg each toxin) of mixed standard samples, and the linear regression equation between the injection amounts of toxins and peak area of each standard toxin and correlation coefficient were calculated using Origin 7.5 software. The regression equations and correlation coefficients were: Y = 23870X + 1370.5 and R = 0.9878 for α-AMA, Y = 16272X – 468.3 and R = 0.9938 for β-AMA. The concentrations of α-AMA and β-AMA in the samples were calculated using peak areas. The concentrations of α-AMA and β-AMA are expressed as the mean ± SD.
3. Results
3.1. Mushroom identification and phylogenetic analysis based on ITS sequences
The macroscopic and microscopic characteristics of L. brunneoincarnata are in accordance with the previous literatures [Citation13,Citation21], and the macroscopic and microscopic characteristics of L. venenata MHHNU 31031 has been described in our previous paper [Citation14]. The identifications were further confirmed by ITS sequences and the results strongly indicated that the samples of MHHNU 31026, MHHNU 31030, and MHHNU 31032 are L. brunneoincarnata with a strong bootstrap support (100%), and L. venenata MHHNU 31031 sample represents an independent species ().
Figure 2. Phylogenetic tree generated from maximum likelihood analysis based on ITS sequences. Maximum likelihood bootstraps over 50% and Bayesian posterior probabilities over 0.90 are reported on the branches. ITS sequences of four Lepoita species in this study are indicated in boldface, and the other ITS sequences of related taxa were retrieved from the GenBank.
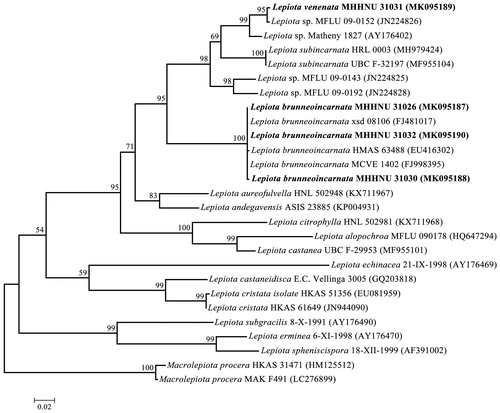
3.2. Identification of the amatoxins based on HPLC-HRMS
The amatoxins extractions of four samples of Lepoita species were analyzed using HPLC-QTOF MS/MS. The HPLC profiles are shown in . The identification results for the six compounds are shown in . Peaks 1, 2, 3, and 4 represent masses corresponding exactly to those of β-amanitin, α-amanitin, amanin, and amaninamide, respectively. Among them, Peaks 3 and 6 represent a mass that corresponds to that of amanin (). We therefore concluded that Peak 6 is likely to be an analog of amanin, which we term “amanin II” hereafter. Peak 4 generated an [M + H+] ion of m/z 903.3681, which could correspond to either amaninamide or γ-amanitin, both of which have a mass of 902.3592. According to the previous report of Sgambelluri et al. [Citation12], Peak 4 is identified as amaninamide. Peak 5 generated an accurate mass, but there are no corresponding known compounds that have been reported.
Table 2. Exact masses of compounds in this study.
3.3. Concentrations of α-AMA and β-AMA in L. brunneoincarnata and L. venenata
The contents of α-AMA and β-AMA of L. brunneoincarnata and L. venenata are shown in . The results show that the L. brunneoincarnata samples contain α-AMA and β-AMA and have total toxin contents ranging from 1.29 to 2.67 mg g−1 dry weight. By contrast, the L. venenata sample contains only α-AMA, β-AMA is absent, the α-AMA content is 1.97 mg g−1 dry weight. In addition, the amatoxin content varies significantly among L. brunneoincarnata samples collected from different locations, with the amount of amatoxin in tested L. brunneoincarnata MHHNU 31032 being twofold more than that of L. brunneoincarnata MHHNU 31030.
Table 3. Main amatoxin contents in two Lepiota species (mg g−1 dry weight).
4. Discussion
Amatoxins, which are responsible for fatal poisonings, are known to be present in some species of Amanita, Galerina, and Lepiota genus [Citation10,Citation22]. The composition and content of amatoxins in lethal Amanita species from Europe, North America, and East Asia by means of modern high-resolution methods have been reported in many studies in the past 30 years [Citation19,Citation20,Citation23–25]; however, up to now, only two studies have reported the amatoxins and phallotoxins in the genus Lepiota by liquid chromatography combined with UV absorbance and mass spectrometry [Citation12,Citation13]. Sgambelluri et al. [Citation12] reported that the α-AMA concentrations in L. brunneoincarnata and Lepiota josserandii were 0.69–0.82 mg/g dry weight and 3.99–4.39 mg/g dry weight, respectively. Yilmaz et al. [Citation13] reported that the α-AMA and β-AMA concentrations in L. brunneoincarnata were 2.38 ± 0.70 and 1.97 ± 0.52 mg/g dry weight, respectively. In this study, the α-AMA concentrations in L. brunneoincarnata and L. venenata were 0.72–1.97 mg/g dry weight, which is in the range of previous reports. The β-AMA concentrations in L. brunneoincarnata were 0.57–0.94 mg/g dry weight, and β-AMA was not detected in L. venenata. Sgambelluri et al. [Citation12] also reported that L. josserandii contained high levels of α-AMA, but β-AMA was absent.
Six compounds were identified in this study, of which β-AMA, α-AMA, amanin, and amaninamide were identified by exact mass. An analog of amanin, which we term “amanin II,” has the same mass as that of amanin but a different elution time, was identified from L. venenata. Additionally, an unknown compound was observed from L. venenata. L. brunneoincarnata contained β-AMA, α-AMA, amanin, and amaninamide, but L. venenata contained only α-AMA, amanin II, and an unknown compound. Lüli et al. [Citation26] recently analyzed the genome of L. venenata (the same sample MHHNU 31031) and illustrated that only one MSDIN gene, named LvAMA1 which codes for α-amanitin, exits in the genome, in addition, only α-amanitin was found in the extract by LC-MS. These results showed that the composition of amatoxins of L. venenata and L. brunneoincarnata is different, which provided a strong chemical evidence for L. venenata as a new species, which was described and illustrated based on morphological and molecular phylogenetic data [Citation14].
5. Conclusion
Six compounds were identified from L. brunneoincarnata and L. venenata by HPLC coupled with mass spectrometry, and the differences in the compounds between L. brunneoincarnata and L. venenata provide chemical evidence for L. venenata as a new species. α-AMA concentrations in L. brunneoincarnata and L. venenata were 0.72–1.97 mg/g dry weight, β-AMA concentrations in L. brunneoincarnata were 0.57–0.94 mg/g dry weight, and β-AMA was absent in L. venenata.
Acknowledgments
The authors are very grateful to Dr. He Xiaolan (Sichuan Academy of Agricultural Sciences, China) for providing the Lepiota brunneoincarnata sample MHHNU 31032 for this study.
Disclosure statement
No potential conflict of interest was reported by the author(s).
Additional information
Funding
References
- Diaz JH. Amatoxin-containing mushroom poisonings: species, toxidromes, treatments, and outcomes. Wilderness Environ Med. 2018;29(1):111–118.
- Ben Khelil M, Zhioua M, Bakir O, et al. Four cases of deadly intoxication by Lepiota brunneoincarnata. Ann Biol Clin (Paris). 2010;68(5):561–567. (French)
- Cervellin G, Comelli I, Rastelli G, et al. Epidemiology and clinics of mushroom poisoning in Northern Italy: a 21-year retrospective analysis. Hum Exp Toxicol. 2018;37(7):697–703.
- Roux X, Labadie P, Morand C, et al. Mushroom poisoning by brunneoincarnata: about two cases. Ann Fr Anesth Reanim. 2008;27(5):450–452. (French)
- Soltaninejad K. Outbreak of mushroom poisoning in Iran: April-May, 2018. Int J Occup Environ Med. 2018;9(3):152–156.
- Varvenne D, Retornaz K, Metge P, et al. Amatoxin-containing mushroom (Lepiota brunneoincarnata) familial poisoning. Pediatr Emerg Care. 2015;31(4):277–278.
- Meunier B, Messner M, Bardaxoglou E, et al. Liver transplantation for severe Lepiota helveola poisoning. Liver. 1994;14(3):158–160.
- Ramirez P, Parrilla P, Sanchez Bueno F, et al. Fulminant hepatic failure after Lepiota mushroom poisoning. J Hepatol. 1993;19(1):51–54.
- Mottram AR, Lazio MP, Bryant SM. Lepiota subincarnata J.E. Lange induced fulminant hepatic failure presenting with pancreatitis. J Med Toxicol. 2010; 6(2):155–157.
- Graeme KA. Mycetism: a review of the recent literature. J Med Toxicol. 2014;10(2):173–189.
- Wieland T. Peptides of poisonous amanita mushrooms. New York: Springer; 1986.
- Sgambelluri RM, Epis S, Sassera D, et al. Profiling of amatoxins and phallotoxins in the genus Lepiota by liquid chromatography combined with UV absorbance and mass spectrometry. Toxins (Basel). 2014;6(8):2336–2347.
- Yilmaz I, Bakirci S, Akata I, et al. Toxin content and toxicological significance in different tissues and development stages of Lepiota brunneoincarnata mushroom. Toxin Rev. 2015;34(3):109–114.
- Cai Q, Chen ZH, He ZM, et al. Lepiota venenata, a new species related to toxic mushroom in China. J Fungal Res. 2018;16(2):63–69.
- Zhang P, Chen ZH, Xiao B, et al. Lethal amanitas of East Asia characterized by morphological and molecular data. Fungal Divers. 2010;42(1):119–133.
- Cai Q, Tulloss RE, Tang LP, et al. Multi-locus phylogeny of lethal amanitas: implications for species diversity and historical biogeography. BMC Evol Biol. 2014;14(1):143.
- Stamatakis A. RAxML-VI-HPC: maximum likelihood-based phylogenetic analyses with thousands of taxa and mixed models. Bioinformatics. 2006; 22(21):2688–2690.
- Ronquist F, Huelsenbeck JP. MrBayes 3: Bayesian phylogenetic inference under mixed models. Bioinformatics. 2003;19(12):1572–1574.
- Tang SS, Zhou Q, He ZM, et al. Cyclopeptide toxins of lethal amanitas: compositions, distribution and phylogenetic implication. Toxicon. 2016;120(15):78–88.
- Wei J, Wu J, Chen J, et al. Determination of cyclopeptide toxins in Amanita subpallidorosea and Amanita virosa by high-performance liquid chromatography coupled with high-resolution mass spectrometry. Toxicon. 2017;133:26–32.
- Razaq A, Vellinga EC, Ilyas S, et al. Lepiota brunneoincarnata and L. subincarnata: distribution and phylogeny. Mycotaxon. 2013;126(1):133–141.
- Enjalbert F, Rapior S, Nouguier-Soulé J, et al. Treatment of amatoxin poisoning: 20-year retrospective analysis. J Toxicol Clin Toxicol. 2002;40(6):715–757.
- Enjalbert F, Gallion C, Jehl F, et al. Amatoxins and phallotoxins in Amanita species: high-performance liquid chromatographic determination. Mycologia. 1993;85(4):579–584.
- Garcia J, Oliveira A, de Pinho PG, et al. Determination of amatoxins and phallotoxins in Amanita phalloides mushrooms from northeastern Portugal by HPLC-DAD-MS. Mycologia. 2015;107(4):679–687.
- Kaya E, Yilmaz I, Sinirlioglu ZA, et al. Amanitin and phallotoxin concentration in Amanita phalloides var. alba mushroom. Toxicon. 2013;76:225–233.
- Lüli YJ, Cai Q, Chen ZH, et al. Genome of lethal Lepiota venenata and insights into the evolution of toxin-biosynthetic genes. BMC Genomics. 2019;20(1):198.