Abstract
The application of antagonistic fungi for plant protection has attracted considerable interest because they may potentially replace the use of chemical pesticides. Antipathogenic activities confirmed in volatile organic compounds (VOCs) from microorganisms have potential to serve as biocontrol agents against pre- and post-harvest diseases. In the present study, we investigated Galactomyces fungi isolated from rotten leaves and the rhizosphere of cherry tomato (Lycopersicon esculentum var. cerasiforme). VOCs produced by Galactomyces fungi negatively affected the growth of phytopathogenic fungi and the survival of nematodes. Mycelial growths of all nine examined phytopathogenic fungi were inhibited on agar plate, although the inhibition was more intense in Athelia rolfsii JYC2163 and Cladosporium cladosporioides JYC2144 and relatively moderate in Fusarium sp. JYC2145. VOCs also efficiently suppressed the spore germination and mycelial growth of A. rolfsii JYC2163 on tomatoes. The soil nematode Caenorhabditis elegans exhibited higher mortality in 24 h in the presence of VOCs. These results suggest the broad-spectrum activity of Galactomyces fungi against various plant pathogens and the potential to use VOCs from Galactomyces as biocontrol agents.
1. Introduction
Globally, we are observing trends moving toward improving the quality and safety of fresh fruits and vegetables by using biocontrol agents and natural materials. Extensive studies have been performed to explore and develop strategies based on microbial antagonists to biologically control pathogens [Citation1,Citation2]. The mechanisms of these microbial antagonists underlying the biocontrol activity, including competition for nutrients and space, enzyme secretion, toxin production, mycoparasitism, volatile organic compound (VOC) release, and resistance induction in plants, are likely to be involved in the antagonistic function [Citation3]. VOCs are low-molecular-weight organic compounds that easily reach the target by vaporizing and diffusing in air. Recently, an increasing number of studies have focused on determining the function of VOCs [Citation4] and their efficiency in reducing the viability and proliferation of devastating plant pathogens [Citation5–7].
The Galactomyces genus of fungi (Saccharomycetales: Dipodascaceae) has long been considered a potential biological control agent owing to its capacity to inhibit the growth of other phytopathogenic fungi by using nonvolatile antimicrobial compounds [Citation8–10]. The inhibition of mycelial growth acts not only on living planted crops but also on harvested fruits, which makes them potentially valuable in controlling both pre- and post-harvest diseases. In our previous study, we observed that G. candidum JYC1146 could inhibit the growth of coexisted fungi by producing VOCs. Such capability was not displayed by the other five genera of the fungi examined in the same study [Citation11]. Moreover, because all the three phytopathogenic fungi (Botrytis cinerea, Fusarium incarnatum, and Colletotrichum gloeosporioides) showed significant inhibition in growth, VOCs from G. candidum JYC1146 exhibited a potentially broad spectrum of activities against sympatric phytopathogens [Citation11].
Broad-spectrum antipathogenic activity has been noted in some bacterial strains but has rarely been observed in VOCs from fungi. In the case of the bacteria, the broad-spectrum antipathogenic activity can be achieved by releasing lytic enzymes, causing permeability changes in the membrane, producing hydrogen cyanide, and secreting siderophores [Citation12]. These mechanisms act on structures shared by several cellular organisms. Thus, despite these studies mainly focusing on phytopathogenic fungi, antipathogenic activities might also occur in other phytopathogens. Although numerous studies have examined the effects of VOCs on pathogenic fungi, their effects on the free-living stages of nematodes have rarely been studied [Citation13,Citation14]. Nematodes cause crop losses by both directly attacking host plants and acting as the vectors of other pathogens [Citation15]. Various methods have been developed against the damage from nematodes including genetic host resistance, cultural practices, chemical applications, government regulatory measures, and biological control [Citation16,Citation17]. However, as the only metazoan phytopathogen with the largest body mass, nematodes are usually harmful for the agricultural environment [Citation18].
The antipathogenic activity of VOCs from yeast-like fungi, Galactomyces, was confirmed in our previous study to be potentially broader than that from other Galactomyces species. In the present study, we further examined the effect of VOCs from three Galactomyces strains, G. candidum JYC1146, G. candidum JYC546, and G. geotrichum JYC549, on nine phylogenetically distant phytopathogenic fungi (belonging to the three classes: Agaricomycetes, Dothideomycetes, and Sordariomycetes) grown on agarose plates. To more directly simulate adoption on postharvest diseases, the effects of VOCs were examined in situ with the phytopathogenic fungi growing on the plate and harvested fruits of marketed cherry tomato. The mortality of the soil nematode Caenorhabditis elegans was also examined to estimate the protection of VOCs against the infection of plant nematodes.
2. Materials and methods
2.1. Fungal inoculum
Three Galactomyces strains were isolated from rotten leaves (G. candidum JYC1146) and the rhizosphere of cherry tomato (L. esculentum var. cerasiforme) (G. candidum JYC546 and G. geotrichum JYC549) for examining the antagonistic effects of VOCs on fungi.
Nine phytopathogenic fungi used for examining the response to VOCs included four fungi, which caused tomato wilt disease (F. proliferatum JYC2153 and JYC2155 and F. incarnatum JYC2156) and southern blight disease (Athelia rolfsii JYC2163); Col. gloeosporioides JYC2139, which causes ripe rot in grapes, bitter rot in apples, and several diseases in fruits and vegetables; and four fungal strains, namely F. incarnatum JYC2140, Cladosporium uredinicola JYC2141, Cla. cladosporioides JYC2144, and Fusarium sp. JYC2145 isolated from rotten strawberries collected from the northern and central regions of Taiwan. Both Galactomyces and phytopathogenic fungi were grown on yeast extract peptone dextrose (YPD) agar plates containing 1% yeast extract, 2% peptone, 2% dextrose, and 2% agar.
2.2. Effects of VOCs on the mycelial growth inhibition of the nine phytopathogenic fungi
The Galactomyces strains used for testing the mycelial growth inhibition were first cultured for 24 h to an OD660nm of 0.8 (approximately 1.26 × 107 cells/mL); 20 μL of the Galactomyces culture was spotted on the center of a 9-cm plate. The plate was placed “mouth to mouth” with another plate by using a piece of pathogenic mycelium (approximately 5 × 5 mm2) in the center. The two plates were wrapped together with parafilm [Citation11]. Each of the nine examined phytopathogenic fungi was tested using all the three Galactomyces strains and one control (paired with the plate without the Galactomyces culture) with six replications.
Areas of the mycelium occupying the plate surface were photographed and measured using Image J after being incubated at 28 °C for 6 days [Citation19]. The mycelial growth inhibition (MGI) was calculated using ([Rc – Rexp]/Rc) × 100%, where Rc is the mean area of the control fungi and Rexp is that of the examined phytopathogenic fungi treated with the candidate Galactomyces strains [Citation20].
2.3. Effects of VOCs on Athelia rolfsii JYC2163
Among the nine phytopathogenic fungi, A. rolfsii JYC2163 intensively responded to VOCs from the Galactomyces fungi and was the only fungus generating spores under laboratory conditions. We selected it for further investigation of spore germination and mycelial growth in situ on tomatoes under treatments of VOCs.
For the investigation of the effects of VOCs on spore germination, conidial suspensions were prepared by pouring 3 mL of Tween 80 solution (Sigma-Aldrich, St. Louis, MO) on 9-cm potato dextrose agar (PDA) plates colonized by A. rolfsii JYC2163. The conidia were gently scratched with a sterile scalpel to pull out spores into Tween 80 solution. The concentrations of the suspensions were measured and adjusted at approximately 5 × 105 conidia/mL by counting the conidia by using a hemocytometer (Paul Marienfeld, Lauda-Königshofen, Germany). The conidial suspension (100 μL) was spread on a YPD agar plate (approximately 5 × 104 conidia per plate) and the cell culture grown in YPD broth of each Galactomyces strain (approximately 1.26 × 107 cells/mL) was inoculated on another YPD plate. The two plates were then placed “mouth to mouth” and wrapped with parafilm. All the three treatments and one control whose conidial plate was paired with a blank YPD plate were repeated six times. After incubation at 25 °C for 24 h, the spores were washed from the plate by using 100 μL of sterile water, and the number of germinated spores in 1 μL of the conidia suspension was counted using a hemocytometer.
The effect of the Galactomyces VOCs in the postharvest control of A. rolfsii JYC2163 on tomatoes was examined according to the modified method suggested by Huang et al. [Citation21]. Cherry tomato fruits with no apparent diseases or visible wounds were bought from a local market. Tomatoes of a similar size were surface sterilized in 70% ethanol for 5 s, washed twice with sterile distilled water, and blotted on paper towels to remove water. Three wounds, approximately 2-mm deep, were made on the stalk, bottom, and side of the tomato on its skin by using sterile pipette tips. The fungus was transported by injecting 20 μL of A. rolfsii suspension with 1.7 × 107 conidia into each wound. Each of the five infected tomatoes was placed in a plastic box along with four YPD plate. In each of the treatments, the plates were cultured by one of the three Galactomyces strains (for examining the effect of VOCs) and the blank YPD as control. Each of the four treatments was repeated six times, and finally, 30 tomatoes were examined in total. The boxes were covered; high relative humidity was maintained using a moistened tissue paper to favor the postharvest onset of the disease. After incubation for 8 days at 25 °C, each tomato was photographed, and the symptom and health areas were calculated using Image J. The areas of five tomatoes sharing the same condition in one box were summarized, and the disease severity was estimated by the ratio of symptom area [symptom area/total area].
2.4. Lethal effect of VOCs on free-living nematodes
The lethal effect of VOCs on the fourth larval stage (L4) of the C. elegans strain N2 was examined using the aforementioned “mouth to mouth” method. Approximately 100 nematodes were each examined in the nematode growth medium (NGM; 3 g of NaCl, 2.5 g of peptone, 17 g of agar, 5 mg of cholesterol, 1 mL of 1 M CaCl2, 1 mL of 1 M MgSO4, 25 mL of 1 M KH2PO4, and 1 L of H2O) covering with a YPD plate cultured by each of the Galactomyces strains, Saccharomyces cerevisiae (for examining the common VOCs of the yeast such as ethanol), or a blank plate as control. All the treatments were repeated six times. For maintaining the growth of C. elegans, the plates were inoculated with using E. coli OP50 cells as the food resource. All the nematodes were treated for 24 h in dark at 25 °C, and survival of 30 randomly selected worms was recorded by their movements or response to touch by using a needle.
2.5. Statistical analysis
Four parameters (MGI values of the nine phytopathogenic fungi, number of germinated spores of A. rolfsii JYC2163, disease severity of tomatoes infected by A. rolfsii JYC2163, and mortality rate of nematodes) were adopted to represent the effects of VOCs and analyzed for statistical significance. Different MGI values obtained using the same VOC treatment were compared using the beta regression model for inherently proportional data with the phytopathogenic fungi acting as the fixed effect. To fit the model, three data points with the minus value closed to 0 were considered as 10−8. Significant differences between the treatments were compared using the Tukey's all-pairwise comparisons. A similar model was also adopted for comparing disease severity with the treatment of fungal VOCs as the fixed effect. Numbers of germinated spores of A. rolfsii JYC2163 were first modeled using the generalized linear model (GLM) with Poisson’s error, and post-hoc multiple comparisons were performed using Tukey's all-pairwise comparisons. The mortality rates of nematodes were modeled using the GLM with binomial error and Tukey's all-pairwise comparisons as the post-hoc analysis. All the analyses were performed using R [Citation22] with packages lmtest, betareg, emmeans, multcompView, and multcomp.
3. Results
3.1. Effects of VOCs on the mycelial growth inhibition of the nine phytopathogenic fungi
The growth of all the nine phytopathogenic fungi was inhibited by the presence of VOCs from the Galactomyces fungi. However, the inhibition levels varied among the treatments (Table S1 and and ). Compared with the other examined fungi, Fusarium sp. JYC2145 was less inhibited in response to VOCs from each of the three Galactomyces strains, whereas A. rolfsii JYC2163 and C. cladosporioides JYC2144 showed higher inhibition levels ().
3.2. Effects of VOCs on the spore germination of Athelia rolfsii JYC2163
Spores treated with VOCs showed significantly lower germination (likelihood ratio test: df = 3, X2 = 113.23, p < 0.001). The number of germinated spores observed in 1 μL of the conidia suspension was 44.83 ± 21.11 for the control and 18.33 ± 7.37, 19.00 ± 14.53, and 17.33 ± 6.56 for treatments with G. candidum JYC1146, G. candidum JYC546, and G. geotrichum JYC549, respectively (). Compared with the control treatment, approximately 38%–42% of spores germinated under VOC treatments, and no significant difference was observed among the different Galactomyces strains.
Figure 3. Germination of spores from Athelia rolfsii JYC2163 after the treatment of VOCs secreted by antagonistic fungi for 24 h. The dashed line indicates the median number of germinated spores without the effect of the antagonistic yeast. Letters indicate significant pairwise differences (Tukey’s all-pair comparisons using the generalized linear regression model with Poisson’s error, p < 0.05).
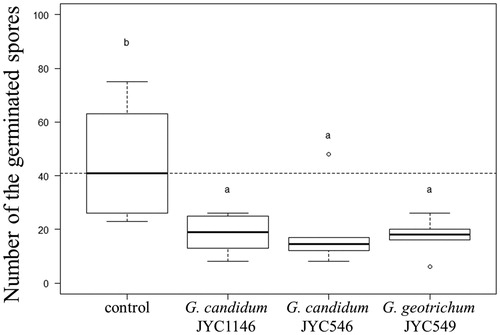
3.3. Effects of VOCs on the mycelial growth of Athelia rolfsii JYC2163 on tomatoes
The infected tomatoes treated with VOCs from all the three Galactomyces strains demonstrated significantly lower disease severity compared with the control (likelihood ratio test: df = 3, X2 = 22.28, p < 0.001). Without VOC treatment, 30.08% ± 8.52% of the fruit surface was occupied by the mycelium, whereas for treated fruits, 8.02% ± 3.33%, 14.30% ± 5.84%, and 12.96% ± 7.54% of the surface were occupied by the mycelium when treated with VOCs from G. candidum JYC1146, G. candidum JYC546, and G. geotrichum JYC549, respectively. Although G. candidum JYC1146 displayed the most efficient reduction in disease severity, no statistical significance was observed among the three Galactomyces strains ().
Figure 4. Efficacy of VOCs produced by the antagonistic fungi in postharvest control of Athelia rolfsii JYC2163 on the marketed cherry tomato. The dashed line indicates the median number of disease severity [symptom area/total area] without the effect of the antagonistic yeast. Letters indicate significant pairwise differences (Tukey's all-pair comparisons using the beta regression model, p < 0.05).
![Figure 4. Efficacy of VOCs produced by the antagonistic fungi in postharvest control of Athelia rolfsii JYC2163 on the marketed cherry tomato. The dashed line indicates the median number of disease severity [symptom area/total area] without the effect of the antagonistic yeast. Letters indicate significant pairwise differences (Tukey's all-pair comparisons using the beta regression model, p < 0.05).](/cms/asset/ef275489-fdf3-4fa4-bc81-69e47dce9e98/tmyb_a_1857042_f0004_c.jpg)
3.4. Lethal effects of VOCs on free-living nematodes
VOCs from all the three Galactomyces strains significantly increased the mortality rate of C. elegans in the L4 stage (likelihood ratio test: df = 4, X2 = 158.32, p < 0.001). The background mortality at 24 h was 7.78% ± 5.44% without VOC treatment. Treatment with S. cerevisiae showed a slightly increased mortality (17.22% ± 6.47%), which was marginally significant under the statistical analysis. The lethal effects exerted by the three Galactomyces strains were significantly higher than those exerted by treatments with both the control and S. cerevisiae. Although the intensities were significantly different among the three Galactomyces strains (52.22% ± 12.59% for G. candidum JYC1146, 55.56% ± 14.86% for G. candidum JYC546, and 40.56% ± 8.28% for G. geotrichum JYC549), their effects might be rather similar, because extreme high mortality was observed in one sample treated with G. candidum JYC546 ().
Figure 5. Lethal effects of the VOCs produced by the fungi on the fourth larval stage of C. elegans strain N2. The dashed line indicates the median mortality rate without the effect of the yeast. Letters indicate significant pairwise differences (Tukey’s all-pair comparisons using the generalized linear regression model with binomial error, p < 0.05). The p value between the control and treatment of S. cerevisiae suggests a marginal significance.
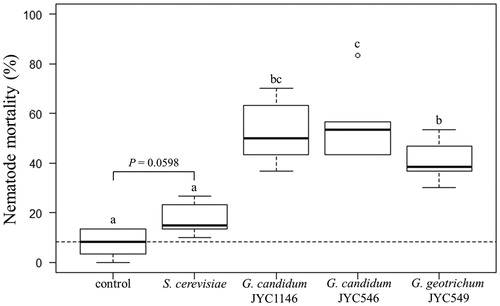
4. Discussion
Fungi commonly exhibit antagonistic activities to manipulate the surrounding microbiota for their own interests. Some of them have also been commercially applied in controlling pre- or post-harvest infections of plants [Citation23]. Galactomyces fungi have been found in various habitats, and some of them are widely applied in the food industry and enzyme production [Citation24]. They are also well known for flavor production [Citation25]. Although a few studies have revealed the effect of Galactomyces fungi on plant health, in our previous study, we observed their potential in controlling pre- or post-harvest diseases [Citation11]. In the present study, we further confirmed their broad-spectrum antagonistic activities against phytopathogenic fungi and free-living nematodes by their production of airborne VOCs.
4.1. Fusarium sp. JYC2145 was less inhibited by VOCs
The broad spectra of antagonistic activities have been reported in some bacterial and yeast species [Citation12,Citation26]. Herein, all the nine phytopathogenic fungi examined in the present study displayed growth inhibition in the presence of VOCs from the Galactomyces fungi; however, the levels of inhibition varied. Fusarium sp. JYC2145 showed a relatively moderate response to the presence of the Galactomyces fungi. The other four Fusarium strains examined in this study displayed moderate to high growth inhibition; therefore, such resistance might not be entirely because of the phylogeny. Except for Fusarium sp. JYC2145, which has never been noted to attack living plants, the other four Fusarium strains are common plant pathogens. To break the defense of living plants, several fungal strains display local adaptation to the current host cultivars and trade off those less encountered [Citation27,Citation28]. Although a similar tradeoff between the epidemic (onto or into the host) and survival (often outside the host) phases remains poorly documented [Citation28], the different inhibition levels among the Fusarium strains in the present study suggest a tradeoff between the capability of competition against aggressive fungi and exploitation of living plants.
4.2. Cladosporium cladosporioides JYC2144 and Athelia rolfsii JYC 2163 were highly inhibited
The growth of Cla. cladosporioides JYC2144 and A. rolfsii JYC 2163 was highly inhibited by VOCs from the Galactomyces strains. Cla. cladosporioides has been reported to occasionally cause blossom blight in strawberries and leaf spots in tomatoes [Citation29–31]. Despite causing plant diseases, the facultative infection and relatively low damage to the host plant make Cla. cladosporioides a less important phytopathogenic fungus. By contrast, it is also a potential biocontrol agent because of its antifungal activity against coexisting phytopathogenic fungi [Citation32]. Hence, growth inhibition caused by the Galactomyces fungi might be a negative factor in the adoption of multiple biocontrol agents. Biocontrol agents potentially offset each other’s potency. In the case of controlling infection in the invasive tropical shrub Mimosa pigr in Australia, the efficiency of the fungal plant pathogen Phloeospora mimosae-pigra was significantly reduced in the presence of another biocontrol agent, the stem-boring moth Neurostrota gunniella [Citation33]. Because the interaction network of microorganisms in the soil is more complex than that in the culture medium, the in vivo effect of the Galactomyces fungi on living plants may be worthwhile to investigate in the future.
The growth of A. rolfsii JYC 2163 was also highly inhibited by the Galactomyces fungi. A. rolfsii (syns. Sclerotium rolfsii) is a soil-borne facultative plant pathogen, which causes southern blight disease in several host plants. Members of Athelia cause economic crop (fruits and vegetables) losses in tropical, subtropical, and warm temperate countries and are difficult to control because of the variety in host range, fastidious growth, and ability to produce persistent sclerotia [Citation34,Citation35]. Fungal metabolites have been considered potential biocontrol agents to avoid negative effects on the environment and human health by chemical agents [Citation34,Citation36]. The reaction of VOCs from the Galactomyces fungi is similar to that of 6-pentyl-alpha-pyrone (6PAP), which is produced by numerous Trichoderma species. 6PAP has been previously noted to suppress the disease caused by A. rolfsii when applied onto the surface of the soil [Citation36]. Although the mechanism underlying the antifungal activity has never been examined in A. rolfsii disease, 6PAP has been observed to inhibit mycelial growth and conidia germination of F. moniliforme [Citation37]. Although the efficiency of VOCs from the Galactomyces fungi has not been examined in living plants, the reduced damage to tomatoes and the marginal efficiency in several developmental stages of disease from A. rolfsii JYC 2163 suggested their potential in controlling postharvest infections.
4.3. Lethal effects on the free-living nematodes
Some studies have suggested that animal or plant residues incorporated into the soil release VOCs, causing biofumigation, and some chemicals from plants have been observed to be directly toxic to the infectious stage of nematodes [Citation13,Citation38]. Such effects explain the efficiency of soil amendments, which are frequently adopted as environmentally friendly strategies to replace the application of chemical nematicides [Citation18]. However, little is known regarding chemicals released from soil microorganisms. Fungi which negatively affect the fitness of soil nematodes can be characterized into two main groups, nematophagous fungi, which exploit nematodes for nutritious substances, and, endophytic fungi, which colonize plant roots and reduce the incidence of plant disease by enhancing plant resistance or releasing enzymes or toxins against nematodes [Citation39,Citation40]. The Galactomyces fungi examined in the present study are more likely to be endophytic fungi, which release volatile toxins. Although a similar effect was observed in S. cerevisiae in our study, nematodes exposed to VOCs from the Galactomyces fungi demonstrated a more intense effect. Some chemicals released from the fungi were detected to be toxic to nematodes. Acetic acid, which inhibits the movement of infesting juvenile nematodes, was detected during the fungal growth of Paecilomyces lilacinus and T. longibrachiatum [Citation41]. Trans-2-decenedioic acid detected in P. ostreatus caused irreversible damage to the free-living nematode Panagrellus redivivus [Citation42]. Despite their potent effects on nematodes, these chemicals released from nematophagous or saprophagous fungi might also be disadvantageous to plants and less likely to be released from endophytic fungi. In addition to the Galactomyces fungi, F. oxysporum and Neotyphodium spp. are known to release toxic compounds against nematodes and enhance plant growth. However, mechanisms underlying nematode resistance in most of the plant–fungus associations are poorly understood [Citation40,Citation43]. Thus, precise analytical methods, such as solid-phase microextraction–gas chromatography–mass spectrometry, should be applied in future studies to confirm whether volatile compounds produced by these Galactomyces fungi.
5. Conclusion
Traditional methods of soil amendments (compost, oats, and straw) limit the incidence of plant disease by manipulating soil communities. However, the efficiency exhibited by opportunistically increasing the population of antagonistic or competitive soil microorganisms is uncertain [Citation34]. In the present study, VOCs from G. candidum JYC1146, G. candidum JYC546, and G. geotrichum JYC549 displayed significant antibiotic activity, which demonstrates potential in controlling plant diseases caused by phytopathogenic fungi and nematodes. The adoption of these yeast-like fungi isolated from the natural environment in agricultural systems is more environmentally friendly and less harmful for human health. The effect of Galactomyces, as a potential biocontrol agent, on living plants and stability of VOCs released in the environment is worth examining in future investigations.
Author contributions
STC and JYC originally formulated the idea and developed the methodology, STC collected samples and carried out experiments, MCC performed statistical analyses and built statistical models, STC, MCC and JYC wrote the manuscript. All authors read and approved the final manuscript.
Supplemental Material
Download MS Word (15.9 KB)Acknowledgments
The authors thank the members of the Chou Laboratory for their helpful discussions and comments on the manuscript. This manuscript was edited by Wallace Academic Editing.
Disclosure statement
No potential conflict of interest was reported by the author(s).
Additional information
Funding
References
- Spadaro D, Droby S. Development of biocontrol products for postharvest diseases of fruit: the importance of elucidating the mechanisms of action of yeast antagonists. Trends Food Sci Technol. 2016;47:39–49.
- Carmona-Hernandez S, Reyes-Pérez JJ, Chiquito-Contreras RG, et al. Biocontrol of postharvest fruit fungal diseases by bacterial antagonists: a review. Agronomy. 2019;9:121.
- Freimoser FM, Rueda-Mejia MP, Tilocca B, et al. Biocontrol yeasts: mechanisms and applications. World J Microbiol Biotechnol. 2019;35:154.
- Morath SU, Hung R, Bennett JW. Fungal volatile organic compounds: a review with emphasis on their biotechnological potential. Fungal Biol Rev. 2012;26:73–83.
- Minerdi D, Bossi S, Gullino ML, et al. Volatile organic compounds: a potential direct long-distance mechanism for antagonistic action of Fusarium oxysporum strain MSA 35. Environ Microbiol. 2009;11:844–854.
- Cernava T, Aschenbrenner IA, Grube M, et al. A novel assay for the detection of bioactive volatiles evaluated by screening of lichen-associated bacteria. Front Microbiol. 2015;6:398.
- Irtwange SV. Application of biological control agents in pre- and postharvest operations. Int J Agric Eng. 2006;8:1–12.
- Eayre CG, Skaria M, Bull CT, et al. An avirulent Galactomyces species that controls green mold of citrus caused by Penicillium digitatum. Subtrop Plant Sci. 2003;55:46–50.
- McKay AH, Förster H, Adaskaveg JE. Toxicity and resistance potential of selected fungicides to Galactomyces and Penicillium spp. causing postharvest fruit decays of citrus and other crops. Plant Dis. 2012;96:87–96.
- Schisler DA, Janisiewicz WJ, Boekhout T, et al. Agriculturally important yeasts: biological control of field and postharvest diseases using yeast antagonists, and yeasts as pathogens of plants. In: Kurtzman CP, Fell JW, Boekhout T, editors. The yeasts. 4th ed. Amsterdam (The Netherlands): Elsevier; 2011. p. 45–52.
- Chen PH, Chen RY, Chou JY. Screening and evaluation of yeast antagonists for biological control of Botrytis cinerea on strawberry fruits. Mycobiology. 2018;46:33–46.
- Zhang L, Khabbaz SE, Wang A, et al. Detection and characterization of broad-spectrum antipathogen activity of novel rhizobacterial isolates and suppression of Fusarium crown and root rot disease of tomato. J Appl Microbiol. 2015;118:685–703.
- Silva JCP, Campos VP, Barros AF, et al. Plant volatiles reduce the viability of the root-knot nematode Meloidogyne incognita either directly or when retained in water. Plant Dis. 2018;102:2170–2179.
- Freire ES, Campos VP, Pinho RSC, et al. Volatile substances produced by Fusarium oxysporum from coffee rhizosphere and other microbes affect Meloidogyne incognita and Arthrobotrys conoides. J Nematol. 2012;44:321–328.
- Riedel RM. Interactions of plant-parasitic nematodes with soil-borne plant pathogens. Agric Ecosyst Environ. 1988;24:281–292.
- Akhtar M, Malik A. Roles of organic soil amendments and soil organisms in the biological control of plant-parasitic nematodes: a review. Bioresour Technol. 2000;74:35–47.
- Oka Y, Koltai H, Bar‐Eyal M, et al. New strategies for the control of plant‐parasitic nematodes. Pest Manag Sci. 2000;56:983–988.
- McSorley R. Overview of organic amendments for management of plant-parasitic nematodes, with case studies from Florida. J Nematol. 2011;43:69–81.
- Fuhrich DG, Lessey BA, Savaris RF. Comparison of HSCORE assessment of endometrial beta3 integrin subunit expression with digital HSCORE using computerized image analysis *(ImageJ). Anal Quant Cytopathol Histpathol. 2013;35:210–216.
- Michereff SJ, Silveira NSS, Reis A, et al. Epiphytic bacteria antagonistic to Curvularia leaf spot of yam. Microb Ecol. 1994;28:101–110.
- Huang R, Li GQ, Zhang J, et al. Control of postharvest Botrytis fruit rot of strawberry by volatile organic compounds of Candida intermedia. Phytopathology. 2011;101:859–869.
- R Core Team. R: a language and environment for statistical computing. Vienna (Austria): R Foundation for Statistical Computing; 2019. Available from: https://www.r-project.org/
- Knudsen GR, Dandurand LMC. Ecological complexity and the success of fungal biological control agents. Adv Agric. 2014;2014:1–11.
- Sacristán N, González L, Castro JM, et al. Technological characterization of Geotrichum candidum strains isolated from a traditional Spanish goats' milk cheese. Food Microbiol. 2012;30:260–266.
- Grondin E, Sing ASC, James S, et al. Flavour production by Saprochaete and Geotrichum yeasts and their close relatives. Food Chem. 2017;237:677–684.
- Pretscher J, Fischkal T, Branscheidt S, et al. Yeasts from different habitats and their potential as biocontrol agents. Fermentation. 2018;4:31.
- Frézal L, Jacqua G, Neema C. Adaptation of a fungal pathogen to host quantitative resistance. Front Plant Sci. 2018;9:1554.
- Le May C, Montarry J, Morris CE, et al. Editorial: plant pathogen life-history traits and adaptation to environmental constraints. Front Plant Sci. 2020;10:1730.
- Gubler WD, Feliciano CJ, Bordas AC, et al. X. fragariae and C. cladosporioides cause strawberry blossom blight. Calif Agr. 1999;53:26–28.
- Nam MH, Park MS, Kim HS, et al. Cladosporium cladosporioides and C. tenuissimum cause blossom blight in strawberry in Korea. Mycobiology. 2015;43:354–359.
- Robles-Yerena L, Ayala-Escobar V, Leyva-Mir SG, et al. First report of Cladosporium cladosporioides causing leaf spot on tomato in Mexico. J Plant Pathol. 2019;101:759–759.
- Wang X, Radwan MM, Taráwneh AH, et al. Antifungal activity against plant pathogens of metabolites from the endophytic fungus Cladosporium cladosporioides. J Agric Food Chem. 2013;61:4551–4555.
- Paynter Q, Hennecke B. Competition between two biological control agents, Neurostrota gunniella and Phloeospora mimosae-pigrae, and their impact on the invasive tropical shrub Mimosa pigra. Biocontrol Sci Technol. 2001;11:575–582.
- Osemwegie OO, Oghenekaro AO, Owolo LO. Effects of pulverized Ganoderma spp., on Sclerotium rolfsii Sacc and post-harvest tomato (Lycopersicon esculentum Mill.) fruits preservation. J Appl Sci Res. 2010;6:1794–1800.
- Jia XH, Fu JF, Wang WH, et al. First report of Athelia bombacina causing postharvest fruit rot on pear. J Integr Agric. 2018;17:2596–2599.
- Dodd SL, Hill RA, Stewart A. Control of Athelia rolfsii disease on lentil seedlings using 6-pentyl-α-pyrone. Soil Biol Biochem. 2000;32:1033–1034.
- El-Hasan A, Walker F, Schöne J, et al. Antagonistic effect of 6-pentyl-alpha-pyrone produced by Trichoderma harzianum toward Fusarium moniliforme. J Plant Dis Prot. 2007;114:62–68.
- Riga E. The effects of Brassica green manures on plant parasitic and free living nematodes used in combination with reduced rates of synthetic nematicides. J Nematol. 2011;43:119–121.
- Casas-Flores S, Herrera-Estrella A. Antagonism of plant parasitic nematodes by fungi. In: Kubicek CP, Druzhinina IS, editors. Environmental and microbial relationships. The Mycota. Vol. 4. Heidelberg: Springer; 2007. p. 147–157.
- Moosavi MR, Zare R. Fungi as biological control agents of plant-parasitic nematodes. In: Mérillon J, Ramawat K, editors. Plant defence: biological control. Progress in biological control. Vol. 12. Dordrecht: Springer; 2012. p. 67–107.
- Favre-Bonvin J, Ponchet M, Djian C, et al. Acetic acid: a selective nematicidal metabolite from culture filtrates of Paecilomyces lilacinus (Thom) Samson and Trichoderma longibrachiatum Rifai. Nematology. 1991;37:101–112.
- Kwok OCH, Plattner R, Weisleder D, et al. A nematicidal toxin from Pleurotus ostreatus NRRL 3526. J Chem Ecol. 1992;18:127–136.
- Hallmann J, Sikora RA. Occurrence of plant parasitic nematodes and non‐pathogenic species of Fusarium in tomato plants in Kenya and their role as mutualistic synergists for biological control of root‐knot nematodes. Int J Pest Manage. 1994;40:321–325.