Abstract
Mushroom strains of Polyporales from the genera Coriolus, Trametes, Pycnoporus, Ganoderma, and Formitella were explored in terms of mycelial growth characteristics for the application of mushroom mycelia as alternative sources of materials replacing fossil fuel-based materials. Among the 64 strains of Polyporales, G. lucidum LBS5496GL was selected as the best candidate because it showed fast mycelial growth with high mycelial strength in both the sawdust-based solid medium and the potato dextrose liquid plate medium. Some of the Polyporales in this study have shown good mycelial growth, however, they mostly formed mycelial mat of weak physical strength. The higher physical strength of mycelial mat by G. lucidum LBS5496GL was attributed to its thick hyphae with the diameter of 13 µm as revealed by scanning electron microscopic analysis whereas the hyphae of others exhibited less than 2 µm. Glycerol and skim milk supported the best mycelial growth of LBS5496GL as a carbon and a nitrogen source, respectively.
Fossil fuels have been used as an important source of energy and material production in human life since they appeared in human history as an important energy source for the Industrial Revolution, which began in the mid-eighteenth century. However, with industrial development and population growth, the demand–supply of materials has exploded, and humans face two risks: resource depletion and environmental destruction due to resource abuse. Therefore, securing eco-friendly energy sources that minimize the use of fossil fuels, developing new recycling methods of materials, and developing restoration technologies for the destroyed environment are key tasks to open a sustainable future.
Fossil fuels are known to be originated primarily from plant materials that flourished in the carboniferous period by creating underground sedimentary layers without decomposition before the agaricomycetes started to break down dead plants [Citation1,Citation2], suggestively together with climate and crustal fluctuations [Citation3]. The capability of agaricomycetes to decompose dead plant is attributed to the destruction of lignin by the activity of several ligninolytic enzymes [Citation4]. This suggests that agaricomycetes have served as a key link between carbon dioxide in the atmosphere and plant biomass as primary decomposers [Citation4]. Apart from their role in the ecosystem, agaricomycetes are grown as edible crops and are recognized as important agricultural products. Recently, efforts have been underway to develop new eco-friendly industrial materials, away from the limited use of fungi, including mushrooms, in traditional industries such as food, antibiotics, and enzyme production. In particular, various mushrooms have been studied in the development of bio-composite materials using mushroom mycelia and agricultural byproducts [Citation5–7]. Common agricultural byproducts such as rice straw, wheat straw, and sawdust are combined with mushroom mycelia and used in the production of various living and industrial components such as insulation, interior materials, furniture, and decorative items. These mycelial biocomposites, combined with 3D printing technology, show the potential to develop into an important eco-friendly material for new industry [Citation8]. In addition, research on the production of fungal meat and leather, which mimic animal meat and leather, respectively, have been conducted and partially commercialized [Citation9,Citation10].
The filamentous growth characteristics of fungi enable the formation of mycelial networks. If fungal network grows vertically and horizontally together with occasion hyphal fusion, it can create thick mycelial tissue which can show the characteristics of animal skins or meat if properly processed. In addition, the mycelial network penetrates into plant substrates, it strengthens the connection between the substrates, enabling the formation of plant-based biocomposite. The physicochemical properties of the fungal biocomposite depend on the unique physiological properties expressed by the genetic information of the fungus as well as physicochemical conditions for the production of the composite, such as substrate type, growth temperature, pH, and carbon dioxide concentration. For example, the mycelial network of Schizophillum commune has been shown to be affected by the expression of hydrophobin and carbon dioxide concentration [Citation11]. For the development of industrial material using the fungal mycelial network, the growth rate of fungal strain, density of cultured mycelium, and strength of individual hyphae are important.
In this study, we explored mushroom strains belonging to the order Polyporales which have been known to grow well and form dense mycelial network. To this end, 64 strains of Polyporales, including Coriolus versicolor, Trametes, Pycnoporus coccineus, Ganoderma lucidum, and Formitella fraxinea, stocked in the Center for Mushroom Molecular Genetics, GNU were screened in terms of mycelial growth. The strains were routinely grown on potato dextrose agar medium (PDA; Oxoid, Hampshire, UK) at 25 °C. The mushroom strains were firstly screened in a sterilized sawdust medium containing 50 g of oak tree sawdust and 100 mL of water in a polypropylene container (120 mm x 80 mm, Phytohealth, SPL Life Sciences, Pocheon, Korea) by inoculating the mycelial culture broth grown in potato dextrose broth (PDB; Oxoid) for a week at 25 °C. The inoculated medium was incubated at 25 °C for 2 weeks under 80% relative humidity. The degree of mycelial growth was measured by a 5-point scale. As a result, five strains of F. fraxinea, including LBS9639FF, LBS9630FF, LBS9257FF, LBS2337FF, and LBS9541FF, and a single strain of C. versicolor, LBS2279CV, were found to be the fastest growing strains ( and Supplementary Table S1). On the other hand, LBS1894CB (C. brevis), LBS8925CV (C. versicolor), LBS1819CV (C. versicolor), LBS9327CH (C. hirsutus), LBS2291TG (T. gibbosa), and LBS9680TG (T. gibbosa) grew poorly ( and Supplementary Table S1).
Figure 1. Growth characteristics of the selected strains of Polyporales in sawdust medium (A,B) and in PDB plate medium (C,D). The results are summarized in Supplementary Table S1.
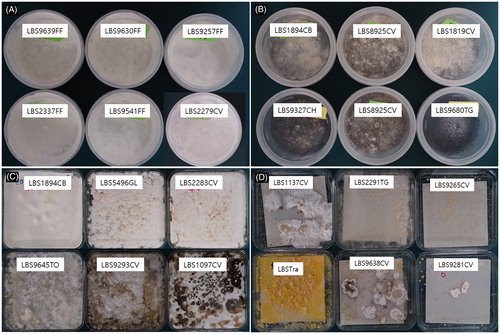
Because the mycelial growth in the sawdust medium took too long incubation time, we next investigated the mycelial growth in potato dextrose liquid plate medium in a sterile rectangular culture plate (126.4 × 126.4 × 20 mm, Square dish, SPL Life Sciences), containing 50 mL PDB plus 50 mL of 1 week PDB grown inoculum. The mushroom strains were incubated at the same conditions as the sawdust medium and the mycelial growth was recorded by a 5-point scale. As a result, the strains of C. versicolor (LBS3442CV, LBS9665CV, LBS5512CV, LBS2283CV, and LBS1140CV), T. gibbosa (LBS1162TG, LBS9303TG, and LBS9421TG), C. brevis (LBS1672CB and LBS1894CB), and G. lucidum (LS5496GL) showed better growth (, Supplementary Table S1). Among them, LBS3442CV, LBS1672CB, LBS9665CV, and LBS5512CV were equally well-growing fungi in sawdust mediums, but LBS1894CB and LBS1162TG rarely grew in sawdust medium (, Supplementary Table S1). C. versicolor LBS9783CV and LBS9281CV, which showed excellent growth in sawdust medium, showed, in contrast, poor growth in liquid culture. Unlike sawdust medium, F. fraxinea LBS9639FF, LBS9630FF, LBS9257FF, LBS2337FF, and LBS9541FF showed moderate growth characteristics. Meanwhile, the color of mycelia formed in the liquid medium also differed significantly depending on the type of mushroom strains. Most of the strains used in the experiment were brown, with the LBS9524CV, LBS9787CV, LBS9293CV, and LBS1819CV making yellow mycelia. Among them, three strains, LBS1894CB, LBS5496GL, and LBS2283CV, produced a relatively uniform white mycelium. These results show that the growth characteristics of mushroom strains and the nature of mycelial mats can vary significantly depending on mushroom strains, substrate, and environmental conditions.
Scanning electron microscopy (SEM) analysis was conducted after drying the mycelium for 24 h at 60 °C obtained through the above liquid culture. The dried mycelial mat was gold-coated using vacuum sputter coater (DSR; element Pi, Beaverton, OR) and examined under a SEM microscope (Jeol JSM-7610F; Joel, Tokyo, Japan). The surface analysis of the fungal mat of F. fraxinea showed a thin layer of primary crumbly membranes, with a thin layer of mycelium (within 1 µm diameter) at the bottom, with a low mycelial density (). C. brevis LBS1894CB, which produces a uniform white mycelium, had very uniform mycelial tissue at around 2.6 µm in hyphal diameter, but the strength of mycelium was weak enough to break by hand (). In the case of LBSCVEYCV, which has a relatively good growth characteristic, the strength of the mycelium was stronger than that of the C. brevis, and the mycelial density was relatively higher ((). Finally, in the case of G. lucidum LBS5496GL, the strength of the mycelium was incomparably stronger than that of other fungi. The mycelial mat was characterized by the formation of a thick hyphae with a diameter of 13 µm () and by the coverage of the mat with a large amount of uncharacterized macromolecules. In fact, the mycelial mat of LBS5496GL was strongest among all examined strains in this study. Mechanical strength of mycelial network is important factor in the industrial application [Citation12]. Tacer-Caba et al. demonstrated the generation of bio-composites with high compressive strength when mushroom strains, such as Agaricus bisporus, Pleurotus ostreatus, and G. lucidum, were cultivated on rapeseed cake [Citation7]. Ganoderma SP. grown on cotton plant materials was also applied to produce biodegradable packaging material [Citation13].
Figure 2. Scanning electron microscopy analyses of mycelial mats from different polypore mushrooms in two different magnifications (×250 and ×900). (A,B) Coriolus brevis LBS1894CB. (C,D) Fomitella fraxinea LBS4388FF. (E,F) Ganoderma lucidum LBS5496GL. (G,H) F. fraxinea LBS2351FF. (I,J) C. versicolor LBSCVEYCV. (K,L) F. fraxinea LBS2349FF. The samples were prepared using the mycelial mats obtained from the PDB plate medium culture. Arrows indicate the hyphal diameters of the mycelium in the mycelial mat. The hyphal diameter of F. fraxinea was less than 1 µm, as indicated.
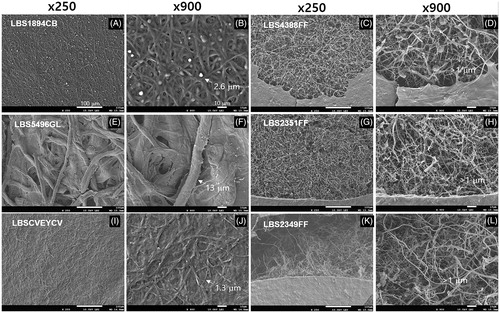
Since G. lucidum is known as a medicinal mushroom, and thus is primarily interested in the fruiting body cultivation, there are very few studies on mycelial growth in solid culture [Citation14] and liquid culture for the production of bio-active compounds [Citation15,Citation16]. Because our sceening experiments confirmed that G. lucidum LBS5496GL produced the best physical characteristic of mycelium, we investigated the effects of carbon and nitrogen sources focusing on the growth of G. lucidum LBS5496GL. First, 5 mL of PDB-grown culture broth was inoculated to the minimal medium composed of yeast nitrogen base (6.7 g/L) and 4 g/L or 10 g/L of individual carbon sources, including galactose, glucose, glycerol, maltose, sorbitol, and sucrose. Nitrogen sources (5 g/L), such as casein, peptone, skim milk, and soytone, were also examined under the same conditions. As a result, galactose, glucose, maltose, and glycerol showed similar mycelial growth while skim milk was the best nitrogen source (). The mycelial growth was dependent on the concentration of skim milk, resulting in the maximum growth at the concentration of 60 g/L (). Based on these results, we conduct a scale-up experiment for large-scale mycelial mat production. First, G. lucidum LBS5496GL was cultured in 500 mL PDB supplementary with 10 g/L glycerol and 30 g/L skim milk. The culture was poured into a polypropylene container (24 × 18 × 6 cm) which contained layers of synthetic cotton in 2 cm and natural cotton in 0.5 cm. The container was incubated for one week at 30 °C with 85% relative humidity. The experiment resulted in a mycelial mat of 24 × 18 × 0.6 cm ().
Figure 3. Effects of carbon and nitrogen sources on the mycelial growth of Ganoderma lucidum LBS5496GL. (A) Effect of carbon sources. Two different concentrations (4 and 10 g/L) of different carbon sources were examined in minimal medium. (B) Effect of nitrogen sources. 5 g/L of each nitrogen source was examined in minimal medium. (C) Effect of the concentrations of skim milk. All the experiments were triplicated. The error bars indicate standard errors of each experimental sets. (D) Mycelial mat generated from scale-up experiment using the optimal conditions.
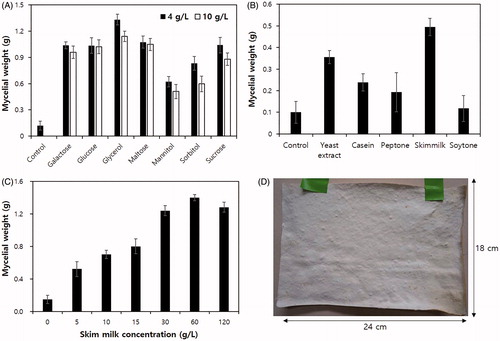
In an effort to replace fossil fuel-based materials, attempts to employ fungal mycelia in the fabrication of eco-friendly composite materials have emerged recently. Particularly, basidiomycetes, such as Daedaleopsis confragosa, A. bisporus, P. ostreatus, and G. lucidum, have been sought for the industrial application in coatings, papers, membranes, packaging materials, composite materials, and leathers due to their physical strength and growth characteristics [Citation17,Citation18]. Accordingly in this study, screening of 64 strains of Polyporales in terms of growth rate and the capability to form mycelial mat was performed, resulting in the finding of G. lucidum LBS5496GL as a potential candidate for further industrial application. Subsequent medium optimization and scale-up experiments allowed the formation of big size mycelial mat. These results are expected to serve as the basis for new industrial applications of fungi, including mushrooms, in the future.
Supplemental Material
Download MS Word (25.8 KB)Disclosure statement
The authors report no conflicts of interest.
Additional information
Funding
References
- Eastwood DC. Evolution of fungal wood decay. In: Schultz TP, Goodell D, Nicholas DD, editors. Deterioration and protection of sustainable biomass. Washington (DC): American Chemical Society (ACS Symposium Series; vol. 1158); 2014. p. 5–93.
- Hawksworth D. Coal measure formation and lignin-degrading fungi. IMA Fungus. 2012;3(2):55–58.
- Nelsen MP, DiMichele WA, Peters SE, et al. Delayed fungal evolution did not cause the Paleozoic peak in coal production. Proc Natl Acad Sci USA. 2016;113(9):2442–2447.
- Floudas D, Binder M, Riley R, et al. The paleozoic origin of enzymatic lignin decomposition reconstructed from 31 fungal genomes. Science. 2012;336(6089):1715–1719.
- de Lima GG, Schoenherr ZCP, Magalhães WLE, et al. Enzymatic activities and analysis of a mycelium-based composite formation using peach palm (Bactris gasipaes) residues on Lentinula edodes. Bioresour Bioprocess. 2020;7(1):58.
- Elsacker E, Vandelook S, Brancart J, et al. Mechanical, physical and chemical characterisation of mycelium-based composites with different types of lignocellulosic substrates. PLoS One. 2019;14(7):e0213954.
- Tacer-Caba Z, Varis JJ, Lankinen P, et al. Comparison of novel fungal mycelia strains and sustainable growth substrates to produce humidity-resistant biocomposites. Mater Des. 2020;192:108728.
- Bhardwaj A, Vasselli J, Lucht M, et al. 3D printing of biomass-fungi composite material: a preliminary study. Manuf Lett. 2020;24:96–99.
- Cho SY, Ryu GH. Effects of mushroom composition on the quality characteristics of extruded meat analog. Kor J Food Sci Technol. 2020;52(4):357–362.
- Ahirwar R, Jayathilakan K, Jalarama RK, et al. Development of mushroom and wheat gluten based meat analogue by using response surface methodology. Int J Adv Res. 2015;3(1):923–930.
- Appels FVW, Dijksterhuis J, Lukasiewicz CE, et al. Hydrophobin gene deletion and environmental growth conditions impact mechanical properties of mycelium by affecting the density of the material. Sci Rep. 2018;8(1):4703.
- Hartmann F, Baumgartner M, Kaltenbrunner M. Becoming sustainable, the new frontier in soft robotics. Adv Mater. 2020;2020:2004413.
- Holt G, Mcintyre G, Flagg D, et al. Fungal mycelium and cotton plant materials in the manufacture of biodegradable molded packaging material: evaluation study of select blends of cotton byproducts. J Biobased Mat Bioenergy. 2012;6(4):431–439.
- Jung IC, Kim SH, Kwon YI, et al. Cultural condition for the mycelial growth of Ganoderma lucidum on cereals. Kor J Mycol. 1996;24(1):81–88.
- Hu G, Zhai M, Niu R, et al. Optimization of culture condition for ganoderic acid production in Ganoderma lucidum liquid static culture and design of a suitable bioreactor. Molecules. 2018;23(10):2563.
- Meng L, Bai X, Zhang S, et al. Enhanced ganoderic acids accumulation and transcriptional responses of biosynthetic genes in Ganoderma lucidum fruiting bodies by elicitation supplementation. IJMS. 2019;20(11):2830.
- Jones M, Mautner A, Luenco S, et al. Engineered mycelium composite construction materials from fungal biorefineries: a critical review. Mater Des. 2020;187:108397.
- Jones M, Gandia A, John S, et al. Leather-like material biofabrication using fungi. Nat Sustain. 2021;4(1):9–16.