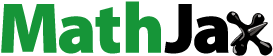
Abstract
Cinnamomum migao plants often face different degrees of drought in karst habitats, which can lead to plants’ death, especially in the seedling stage. Widespread of arbuscular mycorrhizal (AM) fungi in karst soils have the potential to address this drought, which is a threat to C. migao seedlings. We inoculated C. migao seedlings with spores from Glomus lamellosum and Glomus etunicatum, two AM fungi widely distributed in karst soils, to observe seedling growth response after simulated drought. Our results showed that 40 g of G. lamellosum and G. etunicatum significantly promoted the growth of C. migao seedlings, 120 days after inoculation. Following a 15-day drought treatment, root colonization of the seedlings with G. lamellosum or G. etunicatum had lower the accumulation of malondialdehyde (MDA) and increased the accumulation of enzymes and osmotic substances in the seedlings. The relative water content in different organs (roots, stems, and leaves) of the drought-stressed seedlings was higher in plants with G. lamellosum or G. etunicatum than in plants without AM fungi colonization. Our results showed that inoculation with AM fungi was an effective means to improve the drought resistance of C. migao seedlings.
1. Introduction
One of the main challenges facing today in natural resources is water shortage caused by the increasing human population and global climatic change. The Intergovernmental Panel on Climate Change (IPCC) concluded that rising greenhouse gas concentrations lead to general dryness in subtropical regions by the end of this century [Citation1], which will increase drought pressure in forest ecosystems and the global shortage of water resources, threatening the natural regeneration of sustainable forests [Citation2]. Similarly, global climate change has exacerbated drought in karst areas [Citation3], and even when rainfall is sufficient, water holding capacity of the retained soil field can only be used by plants to maintain transpiration for 7–14 days, and the degree of drought is similar to that of long-term dry regions [Citation4,Citation5]. The karst habitat in southwest China has high-rock exposure, shallow and discontinuous soil layers, and frequent substantial drought [Citation6]. Frequent drought stress restricts the growth and distribution of plants in this area. Low-survival rate and poor plant growth are commonly observed during vegetation restoration in karst regions [Citation7].
Cinnamomum migao H. W. Li is a medicinal plant, endemic to southwest China and is only distributed in the dry and hot valleys of the karst transition zone of the three southwestern provinces [Citation8]. C. migao fruits are important raw materials for the pharmaceutical industry and are also used as spices [Citation5]. However, the harvest and utilization of C. migao resources has caused its wild population to become scarce [Citation9]. Artificial planting is an important means to protect C. migao populations in the wild and to address resource shortages. However, the land available for planting in southwest China is mostly karst, with serious rocky desertification and with a weak soil water retention capacity [Citation10,Citation11]. In the early stage of afforestation in the karst region, seedlings often face high-arid conditions, impairing their ability to grow normally. Thus, understanding how to improve the drought resistance of C. migao seedlings is important for artificial cultivation in karst regions [Citation11,Citation12].
Previous research has shown that the symbiotic relationship between plants and arbuscular mycorrhizal (AM) fungi, which are widespread in karst soils, can enhance the drought resistance in most plants [Citation13,Citation14]. AM fungi form stable symbiotic relationships with plant roots, and the extended hyphae can increase the plant’s water-use efficiency. At the same time, these relationships can regulate plant metabolism (such as antioxidant enzymes and peroxides) to alleviate drought damage to plants, thereby improving the tolerance of plants to drought stress. This phenomenon has been observed in C. camphora and C. bodinieri [Citation15,Citation16]. Furthermore, previous research has confirmed that C. migao can co-exist with some AM fungi [Citation17]. If AM fungi symbiosis with C. migao seedlings to improve the drought resistance of the plant, then this will improve our ability to artificially cultivate C. migao in karst regions.
In the present study, we selected Glomus lamellosum and Glomus etunicatum, which can colonize the roots of C. camphora and C. erinaceum, to inoculate seedlings of C. migao. The aim of our study was to explore whether AM fungi improved the drought resistance of C. migao seedlings and to provide a feasible strategy for their artificial cultivation in karst regions.
2. Materials and methods
2.1. Materials
2.1.1. Aseptic seedlings of C. migao
Cinnamomum migao seeds with uniform size and particle size were selected and treated with 200 mg L−1 of Gibberellic acid or Gibberellic A3 (GA3) solution for 48 h. The surface was disinfected with 5% NaClO for 10 min and rinsed with sterile water for four times. The sand was stored in a constant temperature climate chamber at 25 °C for germination. After 20 days of seed germination, the seedlings were removed from the incubator for planting.
2.1.2. AM fungi
Glomus. lamellosum and G. etunicatum were purchased from the Beijing Academy of Agriculture and Forestry (China) and were derived from the propagation of Oxalis corniculata. The soil comprised of spores, hyphae, and mycorrhizal segments on culture medium, and every 20 g of soil contained about 60 spores.
2.1.3. Cultivation substrate
Lime soil (passed through 10-mesh sieve to remove stones and other residue), river sand (particle size 0.7–1 mm), and perlite (particle size 2–4 mm) were mixed in a volume ratio of 5:1:1 mm3. The mixture was sterilized continuously for 2 h at 0.14 MPa and 121 °C [Citation18].
2.2. Experimental design
Glomus lamellosum and G. etunicatum were inoculated into C. migao seedlings separately with five inoculation amounts: 0 (control), 20, 40, 60, and 80 g. The container was a plastic basin with a hole in the bottom (inner diameter of the bottom, 24 cm; inner diameter of the basin, 29 cm; height, 27 cm). Sterile seedlings of 60 days old with the same growth progress (26 ± 2 cm in height and 6 ± 0.5 leaves) were inoculated. After inoculation with AM fungi, during the seedling management period, there was no shading treatment. When the evaporation was strong, 300 ml distilled water was poured into each pot for 1 day. When the evaporation was weak, 300 ml distilled water was poured into each pot for every 3 days, until 150 days after seedling growth. According to previous research on drought tolerance, 1-year-old C. migao seedlings reach their endurance limit when the actual soil water content is about 5% [Citation9]. Therefore, the C. migao seedlings were treated with natural drought stress for 15 days, based on the actual soil water evaporation in the test site.
Seedling height and crown width were measured with a tape measure (precision 0.01 cm), and the stem diameter was measured with a Vernier caliper (precision 0.02 mm). To measure biomass, the complete seedlings were separated into roots, stems, and leaves, and the fresh weight was measured. Then, they were oven dried at 85 °C for 30 mins, and then cooled to 70 °C and baked to a constant weight, to determine the dry weight [Citation19].
The relative water content of leaves was calculated using the following equation:
where RWC is the relative water content, FW is the fresh weight, DW is the dry weight, and SFW is the saturated fresh weight of the leaves [Citation20].
Soluble sugar, soluble protein, proline, malondialdehyde (MDA), superoxide dismutase (SOD), catalase (CAT), and peroxidase (POD) were measured using traditional methods [Citation21].
The mycorrhizal infection rate was determined according to the method of [Citation22]. Trypan blue and acid fuchsin were used for staining, and the colonization rate was expressed by the length of root colonization as follows:
2.3. Statistical analysis
Inter-group difference test (Tukey test) and the Spearman correlation analysis at different indicators were analyzed by using SPSS (version 22.0) with p < 0.05 indicate significant differences. All data visualizations are plotted using Origin (2019; Origin Lab, Northampton, MA, USA).
3. Results
3.1. Colonization rate and growth response of AM fungi after inoculation
After 120 days of inoculation, G. lamellosum or G. etunicatum were colonized successfully in all the treatments (). In general, increasing the amount of inoculation promoted the infection rate of G. lamellosum or G. etunicatum in the root system of C. migao, but increasing the inoculation amount above 40 g G. etunicatum did not improve colonization rate (). Furthermore, microscopic cross-sections of C. migao roots showed the formation of spores and hyphae, confirming successful G. lamellosum or G. etunicatum colonization ().
Figure 1. Colonization of arbuscular mycorrhizal fungi in the roots of Cinnamomum migao seedling. (a) Glomus lamellosum, (b) Glomus etunicatum, (c) and (d) Seedling growth under different treatments.
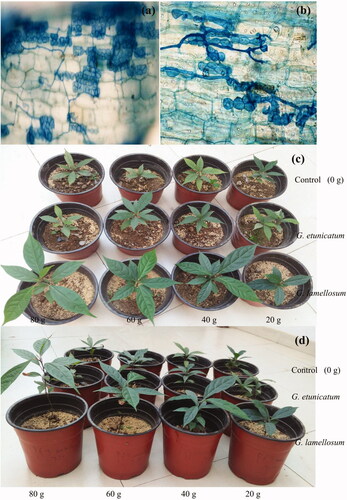
Table 1. Colonization rate of arbuscular mycorrhizal (AM) fungi under different inoculation amounts.
Growth analyses of the seedlings after 120 days of inoculation showed that the inoculation amounts of 20 g and 40 g of G. lamellosum promoted increases in the height, stem diameter, crown width, and number of leaves of C. migao seedlings, but the higher amounts (60 g and 80 g) showed a significant (p < 0.05) inhibitory effect (). In contrast, all inoculation levels of G. etunicatum had growth-promoting effects on all measured variables, except for plant height in the 80 g treatment, which was slightly lower. The improvement to seedling growth with G. etunicatum was the highest in the 40 g treatment (p < 0.05), but gradually weakened when the inoculation levels were increased to 60 g and 80 g. ().
Figure 2. Growth of Cinnamomum migao seedlings under different inoculation treatments at 120 days after inoculation of Glomus etunicatum, Glomus lamellosum. (a) Mean height of C. migao seedlings, (b) Stem diameter of C. migao seedlings, (c) Crown breadth of C. migao seedlings, (d) Number of leaves of C. migao seedlings. Different lowercase letters indicate significant difference (p < 0.05).
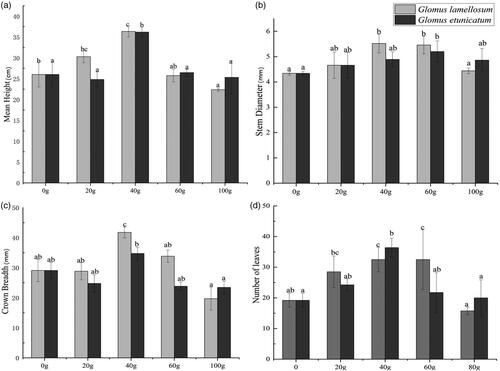
3.2. Physiological response of C. migao seedlings under drought stress
MDA accumulation was significantly reduced in C. migao seedlings (p < 0.05) after inoculating with G. lamellosum or G. etunicatum. Both AM fungi produced a trend of decreasing MDA concentration with minimum levels in seedlings that are treated with 40 g of either AM fungi. However, MDA levels increased again at 60 g and 80 g inoculum of either of the fungi. However, even at the highest inoculation amount (80 g), MDA was significantly lower than that in the control (0 g), indicating that the two AM fungi effectively reduced the accumulation of MDA caused by drought stress ().
Figure 3. Changes in osmoreceptors and enzymes in different inoculation treatments of Cinnamomum migao seedlings after 15 days of drought. (a) MDA of C. migao seedlings, (b) CAT of C. migao seedlings, (c) POD of C. migao seedlings, (d) SOD of C. migao seedlings, (e) SS of C. migao seedlings, (f) SP of C. migao seedlings. Different lowercase letters indicate significant difference (p < 0.05). Abbreviations: MDA: malondialdehyde; CAT: catalase; POD: peroxidase; SOD: superoxide dismutase; SS: soluble sugar; SP: soluble protein.
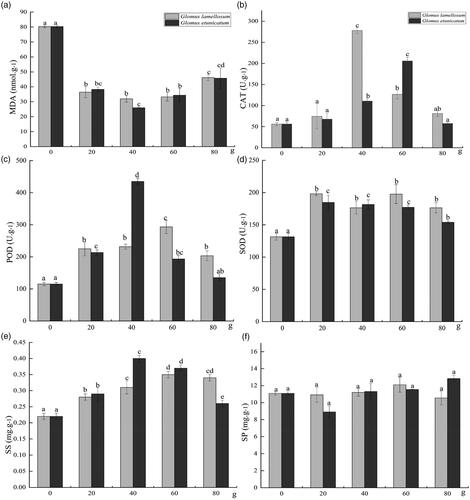
The activity of protective enzymes, CAT and POD, in the seedlings, increased with inoculation level, reaching maximum activities at 40 g inoculation of either AM fungi, but decreased with 60 g and 80 g of AM fungi (). The activities of CAT and POD enzymes in the treatment groups were significantly higher than 40 g and 60 g treated (p < 0.05) (). The SOD enzyme activity in the control group was significantly lower than that in all of the AM fungal treatment groups. Furthermore, there was no difference in SOD between any of the G. lamellosum inoculation levels, the G. etunicatum treatments produced slightly higher SOD activity from 20 g inoculation with gradually decreasing SOD activity, when the inoculation level increased to 80 g (). Treatment with any level of G. lamellosum or G. etunicatum resulted in significantly higher soluble sugar accumulation in C. migao seedlings than untreated control seedlings. G. lamellosum led to a sequential increase in soluble sugar accumulation with increasing inoculation levels. However, seedlings treated with G. etunicatum increased soluble sugar accumulation to a maximum at 40 g before decreasing again to 60 g and 80 g inoculum (). However, there was no significant difference in soluble protein under drought stress after inoculation with G. lamellosum and G. etunicatum in each treatment compared with the control ().
3.3. Water content of C. migao seedlings under drought stress
Under drought stress, plant’s water content changed greatly (). C. migao roots with G. lamellosum or G. etunicatum treatment had greater fresh and dry weights than the control group, showing a trend of increasing first to 40 g of AM fungi and then decreasing at higher inoculum concentrations. In particular, the fresh weight of roots in the G. etunicatum treatment were significantly higher than those in the control group. The change of dry and fresh weight in the stem also increased initially before decreasing. The stem fresh weight in the G. lamellosum and G. etunicatum treatments was significantly higher than that of the control group. The stem dry weight was significantly higher in the control at AM fungi inoculation levels of 40 g, but at high inoculation amounts (60 g and 80 g), stem dry weight was lower than that of the control group. Leaf dry and fresh weight were significantly higher than those in the control group under the two AM fungi treatments, but they also showed a trend of increasing first and then decreasing, peaking at the 40 g inoculation treatment.
Figure 4. Changes in component water content in different treatments of Cinnamomum migao seedlings after 15 days of drought. Left columns represent Glomus lamellosum, and right columns represent Glomus etunicatum. (a) Proportion of fresh weight in different parts of C. migao seedlings, (b) Proportion of dry weight in different parts of C. migao seedlings. Different lowercase letters indicate significant difference (p < 0.05).
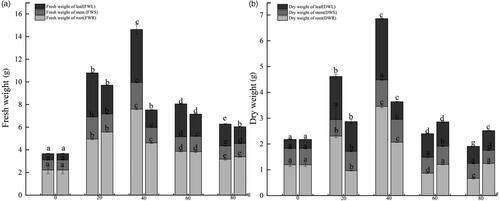
Compared with the control group, inoculation of G. lamellosum and G. etunicatum increased the relative water content of C. migao leaves (). Similar to many other measured parameters, increasing the inoculation levels of G. lamellosum produced a trend of relative water content first increasing and then decreasing with the highest relative water content at 40 g treatment. The dry and fresh weight ratio of the roots of C. migao seedlings treated with G. etunicatum gradually decreased with increasing inoculation amount, indicating enhanced water storage in the roots when G. etunicatum inoculum increased. This decrease in the dry-to-fresh weight ratio was statistically significant at 60 g and 80 g in G. etunicatum when compared to the control group.
Table 2. Water content composition of seedling components of Cinnamomum migao.
The change in dry-to-fresh weight ratio of leaves was similar to that observed in stems, and the ratio was significantly lower than that in the control group after the inoculation treatment with G. etunicatum. The dry and fresh leaf weight of roots, stems, and leaves under the G. lamellosum treatment were lower than those of the control group. The dry-to-fresh weight ratio was not significantly different from that in the control under the 40 g treatment. The dry and fresh weight of the stem in the G. lamellosum treatment were significantly lower than that in the control group, and the lowest was observed in the 20 g treatment. The dry-to-fresh weight ratio of leaves was also significantly reduced after inoculation with G. lamellosum, and the lowest ratio was observed in the 40 g treatment.
3.4. Correlation of different indicators under drought stress
The results of the Spearman correlation analysis () showed that the dry and fresh weight of G. etunicatum under drought stress were significantly positive, which are correlated with POD. The leaf fresh weight was positively correlated with SOD and colonization, and negatively correlated with MDA, significantly (). When treated with G. lamellosum, the root dry weight was significantly positively correlated with CAT, and the stem dry weight was significantly positively correlated with POD and soluble polysaccharides. Other factors following inoculation with these AM fungi had different degrees of influence on the seedling biomass and plant water content of C. migao, but they did not reach a significant level ().
Figure 5. Spearman analysis of growth indexes and related influencing factors under drought treatment. (a) Glomus etunicatum, (b) Glomus lamellosum. Abbreviations: *significant correlation; L-D/FWR: leaf dry/fresh weight ratio; S-D/FWR: stem dry/fresh weight ratio; R-D/FWR: root dry/fresh weight ratio; DWL: dry weight of leaves; FWL: fresh weight of leaves; DWS: dry weight of stem; FWS: fresh weight of stem; DWR: dry weight of root; FWR: fresh weight of root; IR: infection rate; RCW: relative water content; SP: soluble protein; SS: soluble sugar; MDA: malondialdehyde; POD: peroxidase; SOD: superoxide dismutase; CAT: catalase.
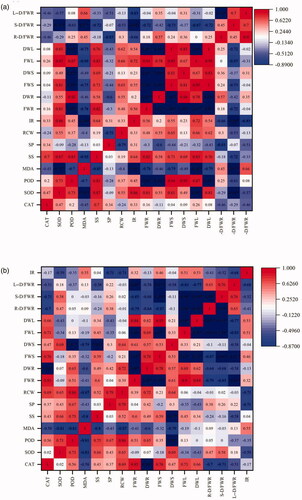
4. Discussion
In this study, we confirmed that both G. lamellosum and G. etunicatum can enhance drought resistance and can promote the growth of C. migao seedlings (). Overall, the strongest drought resistance was observed when G. lamellosum and G. etunicatum were inoculated at 40 g. The same effect was also observed in watermelon and Mesua ferrea [Citation23,Citation24]. These findings are of great importance for early stage seedlings subjected to the arid growing environments of karst regions [Citation25,Citation26].
Plants have evolved a series of defensive mechanisms to adapt to drought stress, including enzymatic reactions and osmotic substances. Long-term drought stress inevitably leads to the excessive production of reactive oxygen species, which cause lipid, DNA, RNA, and protein peroxidation that can eventually lead to cell death. However, inoculation with AM fungi can help slow the damage caused by peroxidation to plant cells. Our results showed that C. migao seedlings inoculated with G. lamellosum and G. etunicatum had increased POD and CAT activity, reduced sugar and osmoreceptor content, and reduced accumulation of MDA under drought stress. A similar physiological response was observed in Cupressus arizonica seedlings inoculated with AM fungi [Citation27]. Previous studies have also reported that AM fungi can eliminate reactive oxygen species by regulating enzymes, like GmarCuZnSOD and GintSOD1, and non-enzymes, such as GintMT1, GinPDX1, and GintGRX1, antioxidant in the fungal network [Citation28–30]. Spearman correlation analysis showed significant correlation between plant fresh weight, SOD, and POD, which also supports the above results (). Other studies have shown that inoculation with AM fungi can increase the levels of osmotic substances in walnut seedlings under drought conditions, which helps to slow down the damage caused by drought stress. However, for C. migao, this effect is reflected in soluble sugar, but is not obvious in soluble protein. This suggests that osmotic substance regulation is not the primary route for enhancing drought tolerance in walnut seedlings after inoculation with AM fungi [Citation31].
The mechanism that plants use to preserve water during drought conditions is the key to their ability to tolerate drought. Studies have shown that AM fungi can improve the growth of host plants by promoting nutrients and water absorption to relieve abiotic stresses, such as drought [Citation32]. After inoculation with G. lamellosum and G. etunicatum, the biomass and tissue water content of C. migao seedlings were higher than those of the control group. The overall water content of G. lamellosum- and G. etunicatum-inoculated plant tissues was the highest when the inoculation amount was at 40 g. Taken together, these results suggest that G. lamellosum and G. etunicatum improve the water-use efficiency of C. migao during drought conditions.
It is generally believed that beneficial symbiotic relationships with soil microorganisms are one of the most successful strategies that terrestrial plants have developed to deal with abiotic stresses [Citation33]. For example, the hyphae that indirectly increase the contact area between the plant roots and the soil. When drought occurs, these epitaxial mycelia can assist plant roots by absorbing water from the soil more efficiently [Citation34,Citation35], improving the water-use efficiency of the plants and enhancing the plants to withstand drought tolerance [Citation36,Citation37], which was confirmed by our results.
In our study, the most direct effect of the inoculation amount was on the colonization rate of our AM fungi with the roots of C. migao seedlings (). A larger inoculation amounts increased the colonization rate of G. lamellosum and G. etunicatum. However, the inoculation amounts of 60 g and 80 g of G. lamellosum or G. etunicatum inhibited the growth of C. migao seedlings (). It is possible that the higher densities of inoculated spores reduces or imbalances the concentrations of key plant hormones [Citation38].
At present, there is no uniform standard for the inoculation amount of AM fungi [Citation24]. Some common means of determining inoculation amount are decided by inoculation potential, the density of spores per unit volume, or the mass of inoculum [Citation39]. However, in actual large-scale agricultural and forestry practices, the AM fungi is used after enriching the culture, not only contain spores but also hyphae, vesicles, mycorrhizal segments, and other propagules, which should be taken into consideration during inoculation [Citation40,Citation41]. Moreover, spore quantification work is time-consuming and laborious, and it is difficult to accurately determine the spore density of fungal agents, which is inconvenient for the field of production practices [Citation42]. In the present study, we selected the traditional spore content as the evaluation index of inoculants. In our study, an inoculum level of 40 g produced the most favorable overall effect in terms of colonization rate, C. migao seedling growth, and drought resistance for both G. lamellosum and G. etunicatum. These results provide a theoretical foundation for the application of inoculants in the field.
Author contributions
Jiming Liu and Qingwen Sun directed the research. Jingzhong Chen and Ruiting Guan performed the experiments and analyzed the data. Xiaofeng Liao contributed to the data analysis and sample collection. Jingzhong Chen and Xiaofeng Liao prepared figures and drafted the manuscript.
Disclosure statement
The authors declare that they have no known competing financial interests or personal relationships that could influence the work reported in this paper.
Data availability statement
The datasets used or analyzed during the current study are available from the corresponding author on a reasonable request.
Additional information
Funding
References
- Drumond A, Stojanovic M, Nieto R, et al. Linking anomalous moisture transport and drought episodes in the IPCC reference regions. Bam Meteorol Soc. 2019;100(8):1481–1498.
- United Nations Environment Programme (UN). UNEP 2009 annual report. UN Environment; 2010.
- Liu B, Chen C, Lian Y, et al. Long-term change of wet and dry climatic conditions in the southwest karst area of China. Global Planetary Change. 2015;127:1–11.
- Zhu H, Wang H, Li P, et al. Biogeography and floristic affinities of the limestone flora in southern Yunnan. Ann Mo Bot Gard. 2003;90(3):444–465.
- Zhou Y, Zhang R, Wang S, et al. Comparative analysis on responses of vegetation productivity relative to different drought monitor patterns in karst regions of southwestern China. Appl Ecol Env Res. 2019;17(1):85–105.
- Trivedi P, Leach JE, Tringe SG, et al. Plant-microbiome interactions: from community assembly to plant health. Nat Rev Microbiol. 2020;18(11):607–621.
- Ni J, Luo DH, Xia J, et al. Vegetation in karst terrain of southwestern China allocates more biomass to roots. Solid Earth. 2015;6(3):799–810.
- Li XW, Li J, Henk W. Flora of China. Beijing: Science Publishing House Missouri Botanical Garden Publishing Press; 2008.
- Zhang XB, Tao Z, Lanping G, et al. Volatile oil contents correlate with geographical distribution patterns of the miao ethnic herb Fructus cinnamomi. Acta Ecol Sin. 2011;31(18):5299–5306.
- Liu CC, Liu YG, Guo K, et al. Influence of drought intensity on the response of six woody karst species subjected to successive cycles of drought and rewatering. Physiol Plant. 2010;139(1):39–54.
- Liu CC, Liu YG, Guo K, et al. Comparative ecophysiological responses to drought of two shrub and four tree species from karst habitats of southwestern China. Trees. 2011;25(3):537–549.
- Wu M, Deng P, Zhao Y, et al. Effects of drought on leaf growth and chlorophyll fluorescence kinetics parameters in Cyclobalanopsis glauca seedlings of karst areas. Ying Yong Sheng Tai Xue Bao. 2019;30(12):4071–4081.
- Liang YM, Chen XY, Feng CY, et al. Influence of plant communities and soil properties during natural vegetation restoration on arbuscular mycorrhizal fungal communities in a karst region. Ecol Eng. 2015;82:57–65.
- Qiao G, Wen XP, Yu LF, et al. The enhancement of drought tolerance for pigeon pea inoculated by arbuscular mycorrhizae fungi. Plant Soil Environ. 2011;57(12):541–546.
- Oliveira JSFD, Xavier LP, Lins A, et al. Effects of inoculation by arbuscular mycorrhizal fungi on the composition of the essential oil, plant growth and lipoxygenase activity of Piper aduncum L. AMB Express. 2019;9:29.
- Zhou T, Yang ZN, Jiang WK, et al. Variation and regularity of volatile oil constituents in fruits of national medicine Cinnamomum migao. Chin J Chin Mater Med. 2010;35(7):852–856.
- Tong BL, Liu JM, Chen JZ, et al. Correlation between fungal diversity in rhizosphere soil and medicinal active components in fruits of Cinnamomum migao. Mycosystema. 2019;38(7):1058–1070.
- Wen AH, Liu JM, Gao P, et al. Effect of natural drought stress on microstructure and chlorophyll content of Cinnamomum migao H.W. Li seedlings. Northern Horticul. 2015;14:62–66.
- Bouzouina M, Kouadria R, Lotmani B. Fungal endophytes alleviate salt stress in wheat in terms of growth, ion homeostasis and osmoregulation. J Appl Microbiol. 2021;130(3):913–925.
- Mazloom N, Khorassani R, Zohury GH, et al. Lignin-based hydrogel alleviates drought stress in maize. Environ Exp Bot. 2020;175:104055.
- Hossain MS, Hossain MD, Hannan A, et al. Salt-induced changes in physio-biochemical and antioxidant defense system in mustard genotypes. Phyton. 2020;89(3):541–559.
- Phillips JM, Hayman DS. Improved procedures for clearing roots and staining parasitic and vesicular-arbuscular mycorrhizal fungi for rapid assessment of infection. Trans Br Mycol Soc. 1970;55(1):158–IN18.
- Geng YF, Qiu Q, Mao JH. Effects of arbuscular mycorrhizal fungi inoculation and different inoculation amount on seedlings of Mesua ferrea. J Fujian Sci Tech. 2016;43(003):67–71.
- Juan JW. The research of arbuscular mycorrhizal fungi on watermelon seedling growth and resistance of Rhizoctonia solani in greenhouse [master’s thesis]. Luoyang: Henan University of Science and Technology; 2014.
- Liu L, Li D, Ma Y, et al. Combined application of arbuscular mycorrhizal fungi and exogenous melatonin alleviates drought stress and improves plant growth in tobacco seedlings. J Plant Growth Regul. 2021;40(3):1074–1087.
- Yang Y. Effect of AM fungi on the growth and nutrient utilization of Cinnamomum bodinieri seedling under increasing CO2 concentration [master’s thesis]. Guiyang: Guizhou University; 2018.
- Hamed A, Ali N, Nematollah E, et al. Biochemical response and interactions between arbuscular mycorrhizal fungi and plant growth promoting rhizobacteria during establishment and stimulating growth of Arizona cypress (Cupressus arizonica G.) under drought stress. Sci Hortic. 2020;261:108923.
- Duc NH, Zsolt C, Katalin P. Arbuscular mycorrhizal fungi mitigate negative effects of combined drought and heat stress on tomato plants. Plant Phy and Biochem. 2018;132:297–307.
- Ren A, Zhu Y, Chen Y, et al. Arbuscular mycorrhizal fungus alters root-sourced signal (abscisic acid) for better drought acclimation in Zea mays L. seedlings. Environ Exp Bot. 2019;167: 1–10.
- Zou YI, Wu QH, Kua K. Unraveling the role of arbuscular mycorrhizal fungi in mitigating the oxidative burst of plants under drought stress. Plant Biol. 2020;23(S1):50–57.
- Behrooz A, Vahdati K, Rejali F, et al. Arbuscular mycorrhiza and plant growth-promoting bacteria alleviate drought stress in walnut. HortSci. 2019;54(6):1087–1092.
- Li J, Meng B, Chai H, et al. Arbuscular mycorrhizal fungi alleviate drought stress in C3 (Leymus chinensis) and C4 (Hemarthria altissima) grasses via altering antioxidant enzyme activities and photosynthesis. Front Plant Sci. 2019;10:499.
- Xiong X, Liu JJ, Wen AH, et al. Physiological response of Cinnamomum migao to water stress. J Anhui Univ (Nat Sci). 2018;42(3):92–97.
- Asrar AA, Abdel GM, Elhindi KM. Improving growth, flower yield, and water relations of snapdragon (Antirhinum majus L.) plants grown under well-watered and water-stress conditions using arbuscular mycorrhizal fungi. Photosynthetica. 2012;50(2):305–316.
- Augé RM. Water relations, drought and vesicular-arbuscular mycorrhizal symbiosis. Mycorrhiza. 2001;11(1):3–42.
- Monneveux P, Ramírez DA, Khan MA, et al. Drought and heat tolerance evaluation in potato (Solanum tuberosum L). Potato Res. 2014;57(3–4):225–247.
- Tan FS, Song HQ, Fu PL, et al. Hydraulic safety margins of co-occurring woody plants in a tropical karst forest experiencing frequent extreme droughts. Agr Forest Meteorol. 2020;292–293:108107.
- Meixner C, Ludwig M, Miersch O, et al. Lack of mycorrhizal autoregulation and phytohormonal changes in the supernodulating soybean mutant nts1007. Planta. 2005;222(4):709–715.
- Carling DE, Brown MF. Relative effect of vesicular-arbuscular mycorrhizal fungi on the growth and yield of soybeans1. Soil Sci Soc Am J. 1980;44(3):528–532.
- Feng F, Sun J, Radhakrishnan GV, et al. A combination of chitooligosaccharide and lipochitooligosaccharide recognition promotes arbuscular mycorrhizal associations in Medicago truncatula. Nat Commun. 2019;10(1):5047
- Klironomos JN, Mccune J, Hart M, et al. The influence of arbuscular mycorrhizae on the relationship between plant diversity and productivity. Ecol Lett. 2000;3(2):137–141.
- Hempel S, Renker C, Buscot FO. Differences in the species composition of arbuscular mycorrhizal fungi in spore, root and soil communities in a grassland ecosystem. Environ Microbiol. 2007;9(8):1930–1938.