Abstract
The genus Aspergillus is commonly isolated from various marine and terrestrial environments; however, only a few species have been studied in rhizosphere soil. As part of the Korean indigenous fungal excavation project, we investigated fungal diversity from rhizosphere soil, focusing on Aspergillus species. A total of 13 strains were isolated from the rhizosphere soil of three different plants. Based on phylogenetic analysis of β-tubulin and calmodulin and morphological characteristics, we identified five Aspergillus species. A. calidoustus and A. pseudodeflectus were commonly isolated from the rhizosphere soil. Four species were confirmed as unrecorded species in Korea: A. calidoustus, A. dimorphicus, A. germanicus, and A. pseudodeflecuts. The detailed morphological descriptions of these unrecorded species are provided.
Keywords:
1. Introduction
Aspergillus is one of the most common fungi in various environments worldwide. Aspergillus is known as plant and human pathogen, mycotoxin producer, and food spoiler. However, it plays important roles in the ecological and industrial systems by producing antibiotics and organic acids, and degrading starches, celluloses, and other polysaccharides [Citation1–5]. Morphological characters such as growth rate, color of the colony, thermotolerance, and size of conidial heads and conidia are known to be important features for initial identification of Aspergillus [Citation6]. However, morphological feature is not enough to recognize species because their morphological characteristics vary by their ecological habitats [Citation6,Citation7]. For accurate identification of Aspergillus, standardized methods including morphology, molecular analysis, and extrolite profiling have been proposed. DNA markers such as the internal transcribed spacer region, calmodulin (CaM), β–tubulin (BenA), and the RNA polymerase II second largest subunit (RPB2) have been used in Aspergillus identification and phylogeny [Citation8]. According to the current research, the genus consists of six subgenera, 27 sections, and 446 species worldwide [Citation9]. In Korea, 69 Aspergillus species have been reported [Citation10–14]. Although some species have been reported from soil in terrestrial, marine, and clinical environments [Citation15–18], many Aspergillus were isolated from food fermentation such as meju and nuruk [Citation12,Citation19–21]. Nonetheless, study on Aspergillus from rhizosphere soil in Korea is limited [Citation22].
Fungi in rhizosphere environments play an important role in plant growth and adaptation [Citation23,Citation24]. Aspergillus is one of the common fungi in rhizosphere soil [Citation25–31]. Some Aspergillus are known to produce plant promoting chemicals such as gibberellic acid and indole acetic acid [Citation27,Citation28]. Many Aspergillus strains were only identified at the genus level, as previous studies mainly focused on bioactive compounds [Citation27–30]. Therefore, the diversity of Aspergillus in in rhizosphere soil is unclear.
This project is organized by the National Institute of Biological Resources to excavate Korean indigenous fungi from the rhizosphere soil. We explored fungal diversity from rhizosphere soil of various plants; Aspergillus, Penicillium, Trichoderma, and Fusarium were common genera. Recently, we reported on diversity of Penicillium, revealing eight unrecorded species in Korea [Citation32]. The main purpose of this study was to focus on Aspergillus in the rhizosphere of various plants and to identify them based on BenA and CaM loci. We discovered four unrecorded species: A. calidoustus, A. dimorphicus, A. germanicus, and A. pseudodeflectus.
2. Materials and methods
2.1. Sample collections and isolation
Rhizosphere soil of three plants (Calystegia soldanella, Orobanche coerulescens, and Sorbus commixta) were collected from five sites in Korea in 2019 (). Five grams of soil for each sample was diluted tenfold in sterile water. A 100 µL of each dilute was plated on dichloran rose bengal chloramphenicol agar (DRBC; Difco, Becton Dickinson). All plates were incubated at 25 °C for 7 days. Based on morphology, Aspergillus-like strains were transferred to potato dextrose agar (PDA; Difco, Becton Dickinson) plate. Strains were stored in 20% glycerol at −80 °C at the Seoul National University Fungus Collection (SFC).
Table 1. The information of Aspergillus strains isolated from rhizosphere soil.
2.2. DNA extraction, amplification, and sequencing
Genomic DNA was extracted from isolated Aspergillus using a modified cetyltrimethylammonium bromide extraction protocol [Citation33]. For the primer sets, Bt2a/Bt2b for BenA and CF1/CF4 or cmd5/cmd6 for CaM, were used [Citation34–36]. PCR was performed in a C1000 thermal cycler (Bio-Rad, Richmond, CA, USA) with previously described methods [Citation37]. The PCR products were purified using the ExpinTM PCR Purification Kit (GeneAll Biotechnology, Seoul, Korea), according to the guideline. DNA sequencing was performed using the PCR primers at Macrogen (Seoul, Korea), using an ABI Prism 3730 genetic analyzer (Life Technologies, Gaithersburg, MD, USA).
2.3. Phylogenetic analysis
The sequences were assembled, proofread, and aligned using MEGA7 [Citation38] and were deposited in GenBank (accession numbers in ). Hamigera avellanea CBS 295.48 was used as the outgroup [Citation39]. Multiple alignments were performed using the default settings of the Multiple Alignment Fast Fourier Transform (MAFFT ver. 7) [Citation40]. Then, each sequence was manually checked and adjusted. Maximum likelihood (ML) phylogenetic tree was performed with RAxML [Citation41] implemented on CIPRES web portal [Citation42], using the GTR + GAMMA model of evolution with 1000 bootstrap replicates.
Table 2. Strains used for phylogenetic analyses in this study.
2.4. Morphological analysis
Morphological analysis of the four unrecorded species was performed on three different culture media using previously described methods: Czapek yeast autolysate agar (CYA; yeast extract, Difco), malt extract agar (MEA; Oxoid), and yeast extract sucrose agar (YES; yeast extract, Difco). The Methuen Handbook of Color was used for the color names and alphanumeric codes for macromorphological characteristics [Citation43]. The microscopic observation was processed under a light microscope (Eclipse 80i, Nikon, Tokyo, Japan) using the samples grown on MEA and CYA.
3. Results
3.1. Species identification
A total of 13 Aspergillus strains were isolated from rhizosphere of three plants. Based on the combined dataset of BenA and CaM sequences, they were identified as five species in two sections with four unrecorded species in Korea ( and and ). Twelve strains were included in section Usti and were identified as four species: A. calidoustus (5 strains), A. germanicus (1), A. insuetus (1), and A. pseudodeflectus (5). A. calidoustus, A. germanicus, and A. pseudodeflectus were unrecorded species in Korea. For section Cremei, one strain was discovered and identified as A. dimorphicus, which was unrecorded species in Korea.
Figure 1. Maximum likelihood (ML) phylogenetic tree of Aspergillus sect. Cremei based on the combined data set of BenA and CaM sequences. Bootstrap values >70 are presented at the nodes. The scale bar represents the number of nucleotide substitutions per site. “T” indicates the ex-type strains. Aspergillus reported in this study are represented in bold. The unrecorded Aspergillus species are accented in color box.
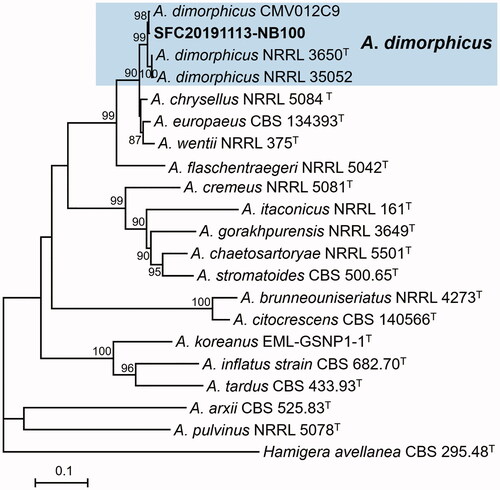
Figure 2. Maximum likelihood (ML) phylogenetic tree of Aspergillus sect. Usti based on the combined data set of BenA and CaM sequences. Bootstrap values >70 are presented at the nodes. The scale bar represents the number of nucleotide substitutions per site. “T” indicates the ex-type strains. Aspergillus reported in this study are represented in bold. The unrecorded Aspergillus species are accented in color box.
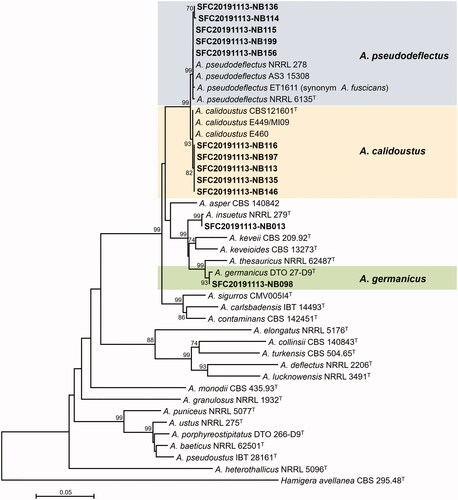
Figure 3. The unrecorded Aspergillus species in Korea: A. calidoustus (SFC20191113-NB146), A. dimorphicus (SFC20191113-NB100), A. germanicus (SFC20191113-NB098) and A. pseudodeflectus (SFC20191113-NB114). (a–c) Colonies grown on Czapek yeast autolysate agar (CYA), malt extract agar (MEA), and yeast extract sucrose agar (YES) from left to right (top = obverse, bottom = reverse). (d–f): Conidiophores; (g) Conidia (scale bar = 10 μm).
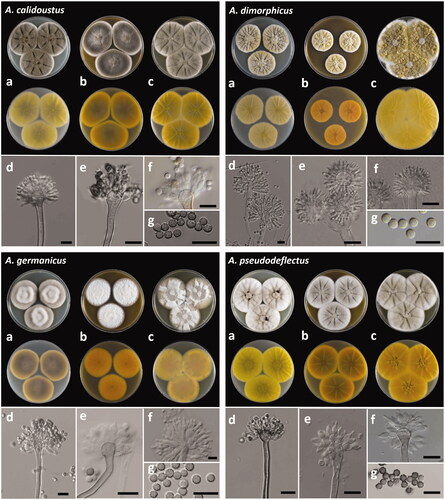
A. calidoustus and A. pseudodeflectus were commonly isolated from the rhizosphere soil (). Aspergillus diversity was higher in rhizosphere soil of Calytegia soldanella compared to others. Although A. pseudodeflectus was commonly isolated from C. soldanella and Sorbus commixta, generally, the Aspergillus diversity was found unique for each plant.
3.2. Taxonomy
Aspergillus calidoustus Varga, Houbraken, & Samson (2008)
Description: Colony diameter, at 25 °C for 7 days, in mm: CYA 50–51; CYA 15 °C 12–13; CYA 30 °C 60–66; CYA 37 °C 8–12; MEA 51–54; YES 50–51 ().
Colonies on CYA, lightly sulcate, moderate to good sporulation, floccose, greenish gray (27D2) elsewhere with 1 mm white margin, exudate brownish orange (6D8) to dark brown (6F8) droplets, soluble pigment absent, reverse color olive brown (4D3) at center and light yellow (1A4) elsewhere. Colonies on MEA, lightly sulcate, moderate sporulation, velvety with floccose at center, central part gray (27B1) at center and greenish gray (27E2) elsewhere with 1 mm white margin, no exudates, soluble pigment absent, reverse color olive brown (4E4) and orange yellow (4B8) elsewhere. Colonies on YES, lightly sulcate, moderated sporulation, central floccose, gray (3C1) to yellowish gray (3C2) elsewhere with 1 mm white margin, no exudates, soluble pigment light yellow (3A4), reverse color olive (3D3) to yellow (3A7).
Conidiophores biseriate with thick, smooth-walled, brown, (2.4–) 3.3 (–4.9) µm wide; vesicles pyriform to broadly spathulate, (8.6–) 9.3 × 9.8 (–10.5) µm; conidial heads loosely columnar; metulae covering the upper half to three-fourths of upper surface, (3.1–) 4.3 × 5.6 (–7.1) µm; phialides (2.8–) 3.2 × 5.4 (–6.7) µm; conidia rough walls, inner and outer wall visible, globose, (3.2–) 3.6 to 3.7 (–4.1) µm.
Strains examined: SFC20191113-NB113, SFC20191113-NB116, SFC20191113-NB135, SFC20191113-NB146, and SFC20191113-NB197
Remarks: A. calidoustus is morphologically similar to A. pseudodeflectus and A. ustus. A. calidoustus was able to grow at 37 °C, but A. ustus was not [Citation44]. A. calidoustus can be distinguished from A. pseudodeflectus by narrow margin and moderate or good sporulation in CYA.
Aspergillus dimorphicus B.S. Mehrotra & R. Prasad (1969)
Description: Colony diameter, at 25 °C for 7 days, in mm: CYA 40–43; CYA 15 °C 10–12; CYA 30 °C 37–39; No growth at CYA 37 °C; MEA 30–31; YES 74–75 ().
Colonies on CYA, moderately sulcate, moderate sporulation, floccose, yellowish gray (3C2) at center, pastel yellow (3A5) elsewhere with 1 mm white margin exudates yellowish white (3A2), soluble pigment absent, reverse color yellowish white (2A2). Colonies on MEA, lightly sulcate, moderate sporulation, floccose, pastel yellow (3A4) elsewhere with 1 mm white margin, exudates yellowish white (3A2), soluble pigment absent, reverse color light orange (5A5). Colonies on YES, lightly sulcate, good sporulation, floccose, olive yellow (3D6) elsewhere with 1 mm white margin, no exudates, soluble pigment absent, reverse color pastel yellow (3A4).
Conidiophores biseriate with smooth-walled, sinuous, light yellow, (3.7–) 4.4 (–6.1) µm wide; vesicles mostly globose to subglobose, (8.0–) 9.9 × 10.3 (–13.4) µm; conidial heads globose to loosely radiate, phialides (3.0–) 3.6 × 9.6 (–11.5) µm; conidia subglobose to globose with rough wall, 3.5 to 4.7 µm.
Strain examined: SFC20191113-NB100
Remarks: A. dimorphicus is morphologically similar to A. wentii, it can be distinguished from A. wentii by branched conidiophore with two vesicles [Citation45].
Aspergillus germanicus Varga, Frisvad & Samson (2011)
Description: Colony diameter, at 25 °C for 7 days, in mm: CYA 42–43; CYA 15 °C 13–14; CYA 30 °C 47–48; CYA 37 °C 8–9; MEA 40–42; YES 45–48 ().
Colonies on CYA, poor sporulation, floccose, orange gray (6B2) to white (26C1) at center, no exudates, soluble pigment yellowish gray (3B2), reverse color brownish gray (4E4) to pale yellow at margin (3A3). Colonies on MEA, poor sporulation, velvety, white, no exudates, soluble pigment absent, reverse color orange (5A7). Colonies on YES, poor sporulation, floccose to velvety, central part color white to grayish yellow (4B4) to white at center, no exudates, soluble pigment pale yellow (3A3), reverse color grayish orange (5B4) at center and grayish yellow (3C4) and light yellow (3A5).
Conidiophores biseriate with thick, smooth-walled, brown, (3.7–) 4.7 (–5.4) µm wide; vesicles septulate, (8.5–) 10.1 × 10.3 (–12.4) µm; conidial heads loosely columnar; metulae covering the upper half to three-fourths of upper surface, (2.3–) 3.2 × 5.2 (–5.8) µm; phialides (2.3–) 2.7 × 5.0 (–6.1) µm; conidia globose with brown smooth wall, (2.8–) 3.1 to 3.4 (–3.9) µm.
Strain examined: SFC20191113-NB098
Remarks: A. germanicus is morphologically similar to A. thesauricus, it can be distinguished from A. thesauricus by growth at 37 °C, thicker conidiophore, and smaller vesicle diameter [Citation46].
Aspergillus pseudodeflectus Samson & Mouchacca (1975)
Description: Colony diameter, at 25 °C for 7 days, in mm: CYA 49–51; CYA 15 °C 10–13; CYA 30 °C 57–59; CYA 37 °C 14–18; MEA 47–48; YES 52–54 ().
Colonies on CYA, lightly sulcate, poor to moderate sporulation, floccose, grayish orange (5B3) at center and white elsewhere, exudates dark brown (8F4), soluble pigment pale yellow (3A3), reverse color olive (3D3) at center and light yellow (2A5) elsewhere. Colonies on MEA, moderately sulcate, poor to moderate sporulation, floccose, brownish gray (5C2) and white at margin, no exudates, soluble pigment absent, reverse color brown (6E5) and golden yellow at margin (5B7). Colonies on YES, radially sulcate and wrinkled at center, poor to moderate sporulation, floccose, yellowish gray (4B2) and white at margin, no exudates, soluble pigment pale yellow (3A3), reverse color deep yellow (4A8).
Conidiophores biseriate with short, curved, rough-walled, brown, (3.4–) 3.9 (–4.2) µm wide; vesicles globose to clavate, (6.7–) 7.9 × 9.5 (–10.5) µm; conidial heads brown, radiate; metulae more or less cylindrical, (2.4–) 3.6 × 4.6 (–5.4) µm; phialides (2.8–) 3.4 × 6.4 (–8.1) µm; conidia globose to ellipsoidal with thick-walled, brown, rough wall, (3.3–) 3.7 to 4.5 (–5.3) µm.
Strains examined: SFC20191113-NB114, SFC20191113-NB115, SFC20191113-NB136, SFC20191113-NB156, and SFC20191113-NB199
Remarks: A. pseudodeflectus is morphologically similar to A. calidoustus. A. pseudodeflectus can be distinguished from A. calidoustus by wide margin and poor sporulation in CYA.
4. Discussion
The rhizosphere soil is a complex and dynamic environment that provides a close relationship between plants and microbes. A total of 13 Aspergillus strains were isolated from rhizosphere soil of three plants and were identified as five species in two sections including four unrecorded species based on BenA and CaM sequences. Although 12 species in Aspergillus section Fumigati have been reported from arable soil [Citation17], many previous studies only focused on limited environments, such as meju and nuruk [Citation12,Citation19–21]. Only Aspergillus terreus has previously been reported from rhizosphere soil of paprika plants in Korea [Citation47]. Five additional species (A. calidoustus, A. dimorphicus, A. germaincus, A. insuetus, and A. pseudodeflectus) are reported for the first time in this study, from the rhizosphere soil in Korea.
Four species were unrecorded in Korea: A. calidoustus, A. dimorphicus, A. germanicus, and A. pseudodeflectus. A. calidoustus is commonly found in clinical environments, indoor air, and forest soil [Citation44,Citation48,Citation49]. It has been isolated from Acanthospermum austral and is known for its antifungal and cytotoxic activity [Citation50]. In this study, A. calidoustus was isolated from the rhizosphere soil of Calystegia soldanella and Sorbus commixta. A. pseudodeflectus was previously isolated from desert soil and seaweed [Citation51,Citation52]. It produced pseudodeflectusin, which exhibited cytotoxic activity [Citation51]. In this study, A. pseudodeflectus strains were isolated from rhizosphere soil of Calystegia soldanella and Sorbus commixta. The A. pseudodeflectus strains isolated from Korea exhibited faster growth on YES compared to the other reported strains [Citation53,Citation54]. Some fungi isolated from different environments exhibit different metabolism and growth rates due to environment adaptation [Citation55,Citation56]. A. germanicus was first isolated from indoor air, but there are few reports of the species so far [Citation57]. In this study, A. germanicus was isolated from the rhizosphere soil of Orobanche coerulescens. Our study is the first report of the species from rhizosphere soil. A. dimorphicus was isolated from garden soil, loess soil, and deep-sea sediment [Citation58–61]. A. dimorphicus showed antitumor activities [Citation62] and proteolytic activities [Citation63]. A. dimorphicus strain was isolated from the rhizosphere soil of Orobanche coerulescens in this study.
Aspergillus species are well known for their potential for usage in industrial and medical compounds, but many strains remain at the genus level. Therefore, we believe that our study will provide the basis for the discovery of new compound based on accurate identification of Aspergillus. Although many Aspergillus species have been found in rhizosphere soils using the NGS method [Citation64–66], the role of Aspergillus in rhizosphere soil is unclear. To understand the interaction between Aspergillus and plants, further studies are needed to investigate the function of Aspergillus in rhizosphere soil.
Disclosure statement
No potential conflict of interest was reported by the author(s).
Additional information
Funding
References
- Planchot V, Colonna P, Gallant DJ, et al. Extensive degradation of native starch granules by alpha-amylase from Aspergillus fumigatus. J Cereal Sci. 1995;21(2):163–171.
- Hrmová M, Biely P, Vrs˘Anská M. Cellulose-and xylan-degrading enzymes of Aspergillus terreus and Aspergillus niger. Enzyme Microb Technol. 1989;11(9):610–616.
- de Vries RP, Visser J. Aspergillus enzymes involved in degradation of plant cell wall polysaccharides. Microbiol Mol Biol Rev. 2001;65(4):497–522.
- Scheckermann C, Wagner F, Fischer L. Galactosylation of antibiotics using the β-galactosidase from Aspergillus oryzae. Enzyme Microb Technol. 1997;20(8):629–634.
- Yang L, Lübeck M, Lübeck PS. Aspergillus as a versatile cell factory for organic acid production. Fungal Biol Rev. 2017;31(1):33–49.
- Geiser DM, Klich MA, Frisvad JC, et al. The current status of species recognition and identification in Aspergillus. Stud Mycol. 2007;59:1–10.
- Balajee SA, Houbraken J, Verweij PE, et al. Aspergillus species identification in the clinical setting. Stud Mycol. 2007;59:39–46.
- Samson RA, Visagie CM, Houbraken J, et al. Phylogeny, identification and nomenclature of the genus Aspergillus. Stud Mycol. 2014;78:141–173.
- Houbraken J, Kocsubé S, Visagie CM, et al. Classification of Aspergillus, Penicillium, Talaromyces and related genera (Eurotiales): an overview of families, genera, subgenera, sections, series and species. Stud Mycol. 2020;95:5–169.
- Kim HJ, Kim JS, Cheon KH, et al. Species list of Aspergillus, Penicillium and Talaromyces in Korea, Based on one fungus one name system. Kor J Mycol. 2016;44(4):207–219.
- Oh JY, Mannaa M, Han GD, et al. First report of Aspergillus awamori as a fungal pathogen of garlic (Allium sativum L.). Crop Prot. 2016;85:65–70.
- Kim KM, Lim J, Lee JJ, et al. Characterization of Aspergillus sojae isolated from meju, Korean traditional fermented soybean brick. J Microbiol Biotechnol. 2017;27(2):251–261.
- Nguyen TT, Pangging M, Bangash NK, et al. Five new records of the family Aspergillaceae in Korea, Aspergillus europaeus, A. pragensis, A. tennesseensis, Penicillium fluviserpens, and P. scabrosum. Mycobiology. 2020;48(2):81–94.
- National List of Species of Korea. 2019. National Institute of Biological Resources. [Internet] [cited 2021 Mar 5]. Available from: http://kbr.go.kr.
- Kim JD. Keratinolytic activity of five Aspergillus species isolated from poultry farming soil in Korea. Mycobiology. 2003;31(3):157–161.
- Kim DH, Kim SH, Kim YK, et al. Reidentification of Aspergillus spp. isolated from clinical specimens of patients suspected as pulmonary aspergillosis in Korea. Korean J Med Mycol. 2009;14(3):133–144.
- Hong SB, Kim DH, Park IC, et al. Isolation and identification of Aspergillus section Fumigati strains from arable soil in Korea. Mycobiology. 2010;38(1):1–6.
- Lee S, Park MS, Lim YW. Diversity of marine-derived Aspergillus from tidal mudflats and sea sand in Korea. Mycobiology. 2016;44(4):237–247.
- Hong SB, Lee M, Kim DH, et al. Aspergillus cibarius sp. nov., from traditional meju in Korea. J Microbiol. 2012;50(4):712–714.
- Yang S, Choi SJ, Kwak J, et al. Aspergillus oryzae strains isolated from traditional Korean nuruk: fermentation properties and influence on rice wine quality. Food Sci Biotechnol. 2013;22(2):425–432.
- Kim HR, Kim JH, Bai DH, et al. Identification and characterization of useful fungi with α-amylase activity from the Korean traditional nuruk. Mycobiology. 2011;39(4):278–282.
- Hong SB, Shin HD, Hong J, et al. New taxa of Neosartorya and Aspergillus in Aspergillus section Fumigati. Antonie Van Leeuwenhoek. 2008;93(1-2):87–98.
- Coats VC, Rumpho ME. The rhizosphere microbiota of plant invaders: an overview of recent advances in the microbiomics of invasive plants. Front Microbiol. 2014;5:368.
- Ehrmann J, Ritz K. Plant: soil interactions in temperate multi-cropping production systems. Plant Soil. 2014;376(1-2):1–29.
- Wijeratne EK, Turbyville TJ, Zhang Z, et al. Cytotoxic constituents of Aspergillus terreus from the rhizosphere of Opuntia versicolor of the Sonoran Desert. J Nat Prod. 2003;66(12):1567–1573.
- Jain R, Saxena J, Sharma V. Solubilization of inorganic phosphates by Aspergillus awamori S19 isolated from rhizosphere soil of a semi-arid region. Ann Microbiol. 2012;62(2):725–735.
- Islam S, Akanda AM, Sultana F, et al. Chilli rhizosphere fungus Aspergillus spp. PPA1 promotes vegetative growth of cucumber (Cucumis sativus) plants upon root colonisation. Arch Phytopathol. 2014;47(10):1231–1238.
- Pandya ND, Desai PV, Jadhav HP, et al. Plant growth promoting potential of Aspergillus sp. NPF7, isolated from wheat rhizosphere in South Gujarat, India. Environ Sustain. 2018;1(3):245–252.
- He W, Xu Y, Fu P, et al. Cytotoxic indolyl diketopiperazines from the Aspergillus sp. GZWMJZ-258, endophytic with the medicinal and edible plant Garcinia multiflora. J Agric Food Chem. 2019;67(38):10660–10666.
- Orfali R, Perveen S. Secondary metabolites from the Aspergillus sp. in the rhizosphere soil of Phoenix dactylifera (Palm tree). BMC Chem. 2019;13(1):1–6.
- Tavakol Noorabadi M, Babaeizad V, Zare R, et al. Isolation, Molecular identification, and mycotoxin production of Aspergillus species isolated from the rhizosphere of sugarcane in the South of Iran. Toxins. 2020;12(2):122.
- Park MS, Lee JW, Kim SH, et al. Penicillium from rhizosphere soil in terrestrial and coastal environments in South Korea. Mycobiology. 2020;48(6):431–442.
- Rogers SO, Bendich AJ. Extraction of total cellular DNA from plants, algae and fungi. In: Gelvin S, Schilperoort R, editors. Plant molecular biology manual. Dordrecht: Kluwer Academic; 1994.
- Glass NL, Donaldson GC. Development of primer sets designed for use with the PCR to amplify conserved genes from filamentous ascomycetes. Appl Environ Microbiol. 1995;61(4):1323–1330.
- Hong SB, Go SJ, Shin HD, et al. Polyphasic taxonomy of Aspergillus fumigatus and related species. Mycologia. 2005;97(6):1316–1329.
- Peterson SW, Vega FE, Posada F, et al. Penicillium coffeae, a new endophytic species isolated from a coffee plant and its phylogenetic relationship to P. fellutanum, P. thiersii and P. brocae based on parsimony analysis of multilocus DNA sequences. Mycologia. 2005;97(3):659–666.
- Park MS, Lee S, Oh SY, et al. Diversity and enzyme activity of Penicillium species associated with macroalgae in Jeju Island. J Microbiol. 2016;54(10):646–654.
- Kumar S, Stecher G, Tamura K. MEGA7: molecular evolutionary genetics analysis version 7.0 for bigger datasets. Mol Biol Evol. 2016;33(7):1870–1874.
- Visagie CM, Hirooka Y, Tanney JB, et al. Aspergillus, Penicillium and Talaromyces isolated from house dust samples collected around the world. Stud Mycol. 2014;78:63–139.
- Katoh K, Standley DM. MAFFT multiple sequence alignment software version 7: improvements in performance and usability. Mol Biol Evol. 2013;30(4):772–780.
- Stamatakis A. RAxML-VI-HPC: maximum likelihood-based phylogenetic analyses with thousands of taxa and mixed models. Bioinformatics. 2006;22(21):2688–2690.
- Miller MA, Pfeiffer W, Schwartz T. Creating the CIPRES science gateway for inference of large phylogenetic trees. Paper presented at: SC10 workshop on gateway computing environments (GCE10), New Orleans, LA; 2010. p. 1–8.
- Kornerup A, Wanscher JH. Methuen handbook of colour. 3rd ed. London: Methuen; 1978.
- Varga J, Houbraken J, Van Der Lee HA, et al. Aspergillus calidoustus sp. nov., causative agent of human infections previously assigned to Aspergillus ustus. Eukaryot Cell. 2008;7(4):630–638.
- Peterson SW. Phylogenetic analysis of Aspergillus sections Cremei and Wentii, based on ribosomal DNA sequences. Mycol Res. 1995;99(11):1349–1355.
- Novakova A, Hubka V, Saiz-Jimenez C, et al. Aspergillus baeticus sp. nov. and Aspergillus thesauricus sp. nov., two species in section Usti from Spanish caves. Int J Syst Evol Microbiol. 2012;62(Pt 11):2778–2785.
- Yoo SJ, Shin DJ, Won HY, et al. Aspergillus terreus JF27 promotes the growth of tomato plants and induces resistance against Pseudomonas syringae pv. tomato. Mycobiology. 2018;46(2):147–153.
- Slack GJ. Identification of secondary metabolites from some Eurotium species, Aspergillus insuetus and A. calidoustus from Canadian homes. Ottawa, Canada: Carleton University; 2008.
- dos Reis Celestino J, de Carvalho LE, da Paz Lima M, et al. Bioprospecting of Amazon soil fungi with the potential for pigment production. Process Biochem. 2014;49(4):569–575.
- Rodrigues de Carvalho C, Vieira MDLA, Cantrell CL, et al. Biological activities of ophiobolin K and 6-epi-ophiobolin K produced by the endophytic fungus Aspergillus calidoustus. Nat Prod Res. 2016;30(4):478–481.
- Samson RA, Mouchacca J. Additional notes on species of Aspergillus, Eurotium and Emericella from Egyptian desert soil. Antonie Van Leeuwenhoek. 1975;41(3):343–351.
- Ogawa A, Murakami C, Kamisuki S, et al. Pseudodeflectusin, a novel isochroman derivative from Aspergillus pseudodeflectus a parasite of the sea weed, Sargassum fusiform, as a selective human cancer cytotoxin. Bioorg Med Chem Lett. 2004;14(13):3539–3543.
- Houbraken J, Due M, Varga J, et al. Polyphasic taxonomy of Aspergillus section Usti. Stud Mycol. 2007;59:107–128.
- Romero SM, Comerio RM, Barrera VA, et al. Aspergillus fuscicans (Aspergillaceae, Eurotiales), a new species in section Usti from Argentinean semi-arid soil. Phytotaxa. 2018;343(1):67–74.
- Bidochka MJ, Menzies FV, Kamp AM. Genetic groups of the insect-pathogenic fungus Beauveria bassiana are associated with habitat and thermal growth preferences. Arch Microbiol. 2002;178(6):531–537.
- Baakza A, Vala AK, Dave BP, et al. A comparative study of siderophore production by fungi from marine and terrestrial habitats. J Exp Mar Biol Ecol. 2004;311(1):1–9.
- Samson RA, Varga J, Meijer M, et al. New taxa in Aspergillus section Usti. Stud Mycol. 2011;69(1):81–97.
- Mehrotra B, Prasad R. Aspergillus dimorphicus and Emericella cleisto-minuta spp. nov. from Indian soils. Trans Brit Mycol Soc. 1969;52(2):331–336.
- Tuthill DE, Christensen M. Aspergillus sepultus, a new species in the Aspergillus ochraceus group. Mycologia. 1986;78(3):475–477.
- Deshmukh SK, Prakash V, Ranjan N. Marine fungi: a source of potential anticancer compounds. Front Microbiol. 2017;8:2536.
- Visagie CM, Houbraken J. Updating the taxonomy of Aspergillus in South Africa. Stud Mycol. 2020;95:253–292.
- Xu R, Xu GM, Li XM, et al. Characterization of a newly isolated marine fungus Aspergillus dimorphicus for optimized production of the anti-tumor agent wentilactones. Mar Drugs. 2015;13(11):7040–7054.
- Rodarte MP, Dias DR, Vilela DM, et al. Proteolytic activities of bacteria, yeasts and filamentous fungi isolated from coffee fruit (Coffea arabica L.). Acta Sci Agron. 2011;33(3):457–464.
- Noor SO, Al-Zahrani DA, Hussein RM, et al. Assessment of fungal diversity in soil rhizosphere associated with Rhazya stricta and some desert plants using metagenomics. Arch Microbiol. 2020;202(9):1–9.
- Pattnaik SS, Busi S. Rhizospheric fungi: diversity and potential biotechnological applications, in recent advancement in white biotechnology through fungi. In: Yadav A, Mishra S, Singh S, Gupta A, editors. Recent advancement in white biotechnology through fungi. Fungal biology. Cham, Switzerland: Springer; 2019. p. 63–84.
- Wu N, Li Z, Wu F, et al. Microenvironment and microbial community in the rhizosphere of dioecious Populus cathayana at Chaka Salt Lake. J Soils Sediments. 2019;19(6):2740–2751.