Abstract
Obligate endoparasitic oomycetes are known to ubiquitously occur in marine and freshwater diatoms, but their diversity is still largely unexplored. Many of these parasitoids are members of the early-diverging oomycete lineages (Miracula, Diatomophthora), others are within the Leptomitales of the Saprolegniomycetes (Ectrogella, Lagenisma) and some have been described in the Peronosporomycetes (Aphanomycopsis, Lagenidium). Even though some species have been recently described and two new genera were introduced (Miracula and Diatomophthora), the phylogeny and taxonomy of most of these organisms remain unresolved. This is contrasted by the high number of sequences from unclassified species, as recently revealed from environmental sequencing, suggesting the presence of several undiscovered species. In this study, a new species of Miracula is reported from a marine centric diatom (Minidiscus sp.) isolated from Skagaströnd harbor in Northwest Iceland. The morphology and life cycle traits of this novel oomycete parasite are described herein, and its taxonomic placement within the genus Miracula is confirmed by molecular phylogeny. As it cannot be assigned to any previously described species, it is introduced as Miracula islandica in this study. The genus Miracula thus contains three described holocarpic species (M. helgolandica, M. islandica, M. moenusica) to which likely additional species will need to be added in the future, considering the presence of several lineages known only from environmental sequencing that clustered within the Miracula clade.
1. Introduction
Straminipilous parasites of phytoplankton are widely occurring and diverse (e.g., plamodiophorids, labyrinthulids, oomycetes), both in marine and freshwater environments [Citation2–5]. These organisms often recur seasonally in parallel blooms their algal host and can cause significant epidemics, for example, in marine diatoms [Citation6–8]. Despite their widespread occurrence, their potential effects on the regulation of diatoms population dynamics during blooms still remain speculative, and general aspects of their biology remain poorly understood [Citation8]. Endobiotic oomycete pathogens are known to infect various centric and pennate diatom species from diverse aquatic habitats, in both limnic and marine environments [Citation9]. Primarily holocarpic, oomycete parasitoids of diatoms are ubiquitous, but mostly known from temperate regions [Citation9–11]. These organisms are classified into six genera (Aphanomycopsis, Diatomophthora, Ectrogella, Lagenidium, Lagenisma, Miracula) scattered throughout the oomycete tree of life, and are known to infect diverse species of centric (e.g., Cosconidiscus granii, Pleurosira laevis) and pennate diatoms (e.g., Gyrosigma acuminatum, Synedra ulna) [Citation12–19]. So far, most of the described species were documented from freshwater, often from studies conducted many decades ago [Citation9,Citation10]. To this, several species from marine environments have been added, which have been assigned to the genera Diatomophthora [Citation19], Ectrogella [Citation12], Lagenisma [Citation15], and Miracula [Citation16]. However, the taxonomy and phylogeny of these organisms remain largely unresolved, since only four marine species (Diatomophthora drebesii, D. perforans, Lagenisma coscinodisci, Miracula helgolandica) have been studied for their molecular phylogeny, while for several others such information is still lacking (e.g., Ectrogella licmophorae, E. eurychasmoides, Petersenia sp.) [Citation14,Citation16,Citation20–23].
While the early-diverging oomycete genus Diatomophthora may contain the largest assemblage of diatom infecting species, the genus Miracula is also diverse and possibly the earliest-diverging lineage of the phylum Oomycota [Citation8]. Miracula was only described recently [Citation16] and currently contains two species, M. helgolandica and M. moenusica, which are obligate endobiotic parasitoids of the marine diatom Pseudo-nitzschia pungens, a producer of the toxin domoic acid [Citation6,Citation16], and the invasive freshwater diatom Pleurosira laevis [Citation18], respectively. Both parasitoids develop simple holocarpic thalli, produce a single discharge tube with a somewhat thickened base, and broadly reniform to grape seed-shaped heterokont zoospores [Citation6,Citation16]. The cellular ultrastructure of the type species, M. helgolandica, has also been documented, showing several features characteristic of the early-diverging oomycetes [Citation6]. Both known species of Miracula were observed to be seasonally recurring along with their diatom host [Citation8,Citation24]. Prior to the introduction of the genus Miracula, its type species, M. helgolandica, has been initially speculated to be a member of either Ectrogella, Olpidiopsis or related to the Eurychasma/Haptoglossa lineage [Citation6,Citation25,Citation26], but was found to be distinct, clustering together with divergent environmental sequences corresponding organisms that still remain to be discovered [Citation16,Citation25,Citation26].
While screening for diatom-infecting oomycetes from marine phytoplankton sampled along the coastline of Skagaströnd in Northwest Iceland during autumn of 2019, endobiotic holocarpic parasites were found sporadically occurring in a centric diatom of the genus Minidiscus (Thalassiosira s. lat.), which has not been previously reported as host for an oomycete parasitoid. It was the aim of this study to characterize the morphology and life cycle of this biotrophic pathogen as well as to obtain sequence data to infer its phylogenetic relationships with other holocarpic oomycetes.
2. Materials and methods
2.1. Sampling, isolation, and characterization
Diatom samples were collected during autumn on the 11th of September 2019 from Skagaströnd harbor, Northwest Iceland (65°49′29.4′′N 20°19′04.3′′W), using a 20 µm mesh plankton net (Hydro-Bios, Kiel, Germany) horizontally towed at 1–2 m depth, and the plankton concentrate was poured into a 250 mL plastic bottle for transportation. Subsequently, about 10 mL of the plankton concentrate was poured into several 9 cm Petri dishes (Sarstedt, Nümbrecht, Germany) and screened for oomycete-infected diatoms using an inverted compound light microscope (AE31, Motic, Xiamen, China). Infected diatoms were individually picked using a 10 µL micropipette (Brandt, Wertheim, Germany), transferred multiple times through autoclaved seawater, and transferred to 2 mL tubes containing 0.5 mL RNAlater solution (Invitrogen, Thermo Fisher Scientific, Waltham, USA) or 70% ethanol for subsequent DNA extraction or deposition, respectively. Approximately 40–50 diatoms infected with Miracula were collected this way for DNA extraction. Samples preserved in 70% ethanol were deposited in the herbarium collection of the Senckenberg Museum of Natural History (Herbarium Senckenbergianum, FR), Cryptogams Section, Frankfurt am Main, Germany (accession numbers: FR-0046129 for isolate 1, FR-0046130 for isolate 2, both established from the same sample). Infected cells were also mounted onto glass slides using sterile seawater for morphological characterization and DIC micrographs of life cycle stages using a compound light microscope (Imager2, Carl Zeiss, Göttingen, Germany) equipped with a Zeiss Axiocam MRc5 (Carl Zeiss). The identity of the diatom host was determined by light microscopy and 18S rRNA gene sequence barcoding as outlined for the oomycetes below. Axenic host and parasite dual culture of the isolated endoparasitoid was also attempted in Guillard’s f/2 medium [Citation27], however, until the axenic host culture was successfully established, the parasitoid had already vanished from the mixed culture.
2.2. Molecular methods
DNA extraction of the oomycete infecting Minidiscus sp. was performed using an innuPREP Plant DNA extraction Kit (Analytikjena, Jena, Germany), as previously described [Citation16]. Isolated diatoms were initially centrifuged at 19,000 g for 2 min at 22 °C to concentrate the cells. Subsequently, RNAlater was carefully removed using 1000 µL pipette tips, and 400 µL SLS buffer solution from the extraction kit was added. About 100 mg of sterile 0.1 mm Silica Glass Beads (Carl Roth GmbH, Germany) were added into each of the 2 mL tubes (Sarstedt, Nümbrecht, Germany) and homogenized at 25 Hz for 25 min in a Retsch Mixer Mill MM 200 (Retsch GmbH, Haan, Germany). The amplification of the nuclear encoding small subunit (nrSSU) was performed as described earlier [Citation17] using Mango-Taq DNA Polymerase (Meridian Bioscience, Memphis, USA) with the 18S primer pair EUK422-445 and EUK1422-1440_R [Citation28]. PCR cycling was carried out on an Eppendorf Mastercycler proS (Eppendorf AG, Hamburg, Germany) equipped with a vapo.protect lid, with an initial denaturation at 95 °C for 4 min, 40 cycles at 95 °C for 20 s, 58 °C for 20 s and 72 °C for 60 s, and concluding with a final elongation at 72 °C for 8 min. Amplicons were sent for sequencing to the laboratory center of the Senckenberg Biodiversity and Climate Research Center, Frankfurt am Main (SBiK-F, Frankfurt am Main, Germany) using the 18S primer pairs used in the PCR. In addition, single cell PCRs were also done as described in Buaya et al., [Citation17] using single infected cells directly added to 5 µL of molecular grade water (Life Technologies, Thermo Fisher Scientific, Waltham, USA) and supplemented with PCR reaction mixes. To obtain high-quality nrSSU sequences of the oomycete infecting Thalassiosira spp., positive PCR amplicons were also cloned as previously described [Citation23]. For this, single bacterial colonies were picked into 20 µL molecular grade water and colony PCR was carried out using the MangoTaq DNA Polymerase and M13-F and M13-R plasmid primers with amplification conditions set to an initial denaturation at 96 °C for 10 min, 36 cycles at 96 °C for 20 s, 56 °C for 20 s and 72 °C for 60 s, and concluding with a final elongation at 72 °C for 10 min. Amplicons from the colony PCRs were sent for sequencing to the laboratory center of SBiK-F using M13 (M13-F, M13-R), T3, and T7 plasmid primers. The resulting sequences were edited and assembled using Geneious (version 5.6). The final sequence of the parasitoid was aligned together with reference sequences of oomycete species in the early diverging lineages, using the Q-INS-i algorithm of mafft [Citation29] on the TrEase webserver (http://thines-lab.senckenberg.de/trease/). The same server was also used for Maximum Likelihood inference using standard settings with 1000 bootstrap replicates. Bayesian inference was done there as well, using MrBayes, version 3.2 [Citation30], and 5 million generations, with all parameters set to default. Phylogenetic analyses using the Minimum Evolution algorithm were done using MEGA7 [Citation31] as described in Buaya et al. [Citation23], with 1000 bootstrap replicates and default parameters, apart from using pairwise deletion and the Tamura–Nei substitution model. The consensus partial 18S (nrSSU) sequence obtained in this study was deposited in GenBank (accession number MW620066).
3. Results
3.1. Parasite screening and detection
In the current study, oomycete endoparasitoids were found in the centric diatom Minidiscus sp. collected during autumn of 2019 from Skagaströnd harbor, Northwest Iceland. About 10–20% of the Minidiscus sp. individuals screened from Skagaströnd were observed to contain oomycete infections. Individuals of various other genera of centric diatoms (e.g., Coscinodiscus, Chaetoceros, Bacteriastrum, Odontella) and Pseudo-nitzschia spp. (previously reported as host for Miracula helgolandica [Citation16]) were abundant in the plankton sample, but none were observed to be parasitized during the examination period. To allow further propagation of the host diatom and to increase chances of re-isolating the target oomycete pathogen, plankton samples were further incubated for 4–6 weeks, supplemented with f/2 marine enrichment medium [Citation27] and kept at controlled conditions as previously described in Buaya et al. [Citation32]. These samples were screened daily until the density of Minidiscus sp. cells declined in the culture. Infections were observed to increase rapidly but disappeared quickly after infesting and consuming susceptible Minidiscus sp. cells.
3.2. Morphology and life-cycle traits
The development of the isolated parasite found in this study, from the start of infection until zoospore release was documented using several infected Minidiscus sp. individuals (). The host normally contained one or two spherical, subspherical, to ovoid endobiotic holocarpic thalli (). These amorphous silvery thalli where mostly globose to subglobose and measured 25–50 µm by 20–45 µm as viewed from above. They rarely pushed apart the host valves at maturity and did not cause hypertrophy. The thalli were surrounded by a thin, colorless wall. The life cycle of the parasite started when an encysted spore on the outer surface of the host frustules germinated at the girdle bands, developing a very fine, needle-like penetration tube that elongated into the host cytoplasm. In the first stages, the parasitoid developed usually close to the host nucleus (). Once established, thalli grew and developed rapidly, gradually deforming the host phaeoplasts and degrading them into orange-brown to chestnut-colored residues at the equatorial periphery (), or sometimes evenly around the thalli (). Subsequently, the amorphous thalli became highly granulated and differentiated into irregularly shaped zoospores initials (), which slowly underwent maturation into grape-seed-like biflagellate zoospores. At this stage, a single, usually 3–5 µm wide, conical discharge tube with a slightly thickened base developed close to the girdle region and exited between the diatom valves (, red arrows). Shortly before discharge, mature zoospores become briefly motile within the sporangium, before the majority of the zoospores were driven out slowly, swimming away randomly in all directions. The zoospores were about 3 µm broad and 5 µm long, with two subapically inserted oppositely directed flagella. After a few minutes of motility, zoospores came to a rest. If they assume movement again has not been observed.
Figure 1. DIC light microscopy of Miracula islandica at different life cycle stages in the centric marine diatom Minidiscus sp. (A) Early infection stage, with developing endobiotic thallus close to the host nucleus; (B, C) developing thallus at an early stage of development (B), surrounded by orange-brown to chestnut-colored residues (C); (D) mature thallus surrounded by degraded phaeoplast residues in multiple infected host cells in chains of Minidiscus sp.; (E) final stage of zoospores maturation, with almost fully developed zoospores compacted within the thallus; (F) empty sporangium, each with a single short discharge tube with a slightly thickened base (red arrows) and surrounded by smooth colorless spherical wall. Scale bar = 50 µm in all photos.
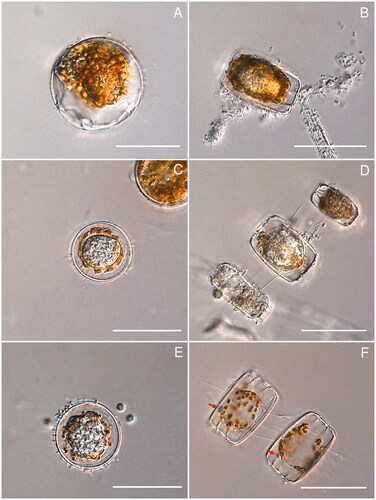
3.3. Molecular phylogeny
The phylogenetic reconstructions based on partial small ribosomal subunit sequences (18S) of the oomycete parasitoid of Minidiscus sp. resolved it as a member of the genus Miracula, forming a monophyletic group with a picoplankton environmental sequence from Helgoland [Citation33]. This lineage grouped together with M. helgolandica and several other sequences derived from environmental sequencing with maximum support in all analyses (). Collectively, together with M. moenusica and some other environmental sequences, they formed the earliest-diverging clade of the oomycetes, but with low support. The branching order of the early diverging orders Olpidiopsidales, Anisolpidiales, Diatomophthorales, and Pontismatales could not be resolved, but Eurychasmales and Haptoglossales consistently grouped together with moderate to strong support. Except for Aphanomycopsis bacillariacearum, Lagenisma coscinodisci, and Ectrogella bacillariacearum, all other holocarpic parasites of diatoms branched before the split of Saprolegniomycetes and Peronosporomycetes.
Figure 2. Molecular phylogeny based on minimum evolution analysis inferred of partial nrSSU (18S) sequences. Numbers on branches denote bootstrap values from maximum likelihood, minimum evolution, and Bayesian analyses, in the respective order. A dash “-” indicates less than 50% bootstrap support for the presented topology in ML and MN, and less than 0.9 posterior probability in BA, for the present or a conflicting topology.
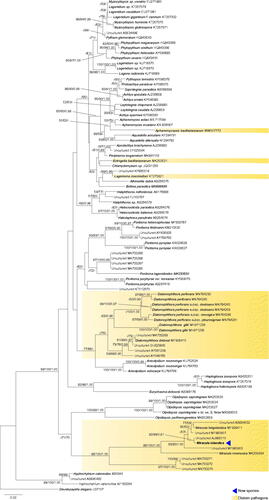
3.4. Taxonomy
Based on its unique life cycle traits, apparent host specificity, and its phylogenetic distinctiveness from Miracula helgolandica, a new species of Miracula is introduced here.
Miracula islandica A.T. Buaya & Thines, sp. nov. ().
Mycobank: MB838821
Etymology: Named after Ísland, the country and island from which the species was first isolated.
DNA barcode: nrSSU [MW620066]
Description: Thallus holocarpic, endobiotic, usually one, rarely two in Minidiscus sp., naked at early-development, with undifferentiated amorphous cytoplasm, later surrounded by a thin colorless smooth wall, mature thalli with spherical to ellipsoidal shape, mostly 25–50 µm by 20–45 µm in diameter, disintegrating the host phaeoplasts and cytoplasm into orange-brown to chestnut-colored residues, causing neither hypertrophy nor disintegration of the host frustule; discharge tube single, very short, 3–5 µm wide, with a very slightly thickened base, forming at the girdle band; zoospores broadly grape seed shaped, biflagellate, 3 µm long and 5 µm broad, forming within the thallus, with subapically inserted flagellum, swarming within sporangium prior to discharge, swimming in random directions upon discharge; resting spores not observed.
Type: Iceland, Skagaströnd, 11th of September 2019, collected by Bettina Scholz, Marco Thines, and Anthony T. Buaya, isolated by Anthony T. Buaya, FR-0046129 (holotype). Ex-type partial nrSSU sequence MW620066.
Known distribution: Skagaströnd, Iceland and Helgoland, Germany [Citation33]
4. Discussion
Early-diverging oomycetes parasitizing diatoms are widespread and were reported from different centric [Citation15,Citation23] and pennate [Citation6,Citation34] hosts. Most of these organisms were described from freshwater habitats in cool temperate regions decades ago [Citation9,Citation10]. In marine environments, comparatively few species were reported until recently, which are belonging to four different genera, of which two are placed among the early-diverging oomycete lineages (Diatomophthora, Miracula), and two within the Saprolegniomycetes (Ectrogella, Lagenisma) [Citation12,Citation15,Citation16,Citation19]. The recently introduced genus Miracula might be the earliest-diverging oomycete clade, and contains two species (M. helgolandica, M. moenusica) [Citation16,Citation18] to which we add a third species in this study.
Phylogenetic inference from recent taxonomic and metabarcoding surveys [Citation16,Citation24–26], revealed an array of diverse sequences from widespread, undescribed holocarpic species. Most of these are a grouping within the early diverging genera Miracula and Diatomophthora. Those that grouped together with M. helgolandica, the type species of the genus Miracula have been observed from different temperate to arctic localities (e.g., Sagami Bay, Concarneau Bay, Atlantic Ocean, North Sea, Arctic Ocean). For some of these sequences, potential hosts were recorded as Pseudo-nitzschia spp. (e.g., P. australis), Melosira spp., and Licmophora spp. (e.g., M. nummuloides) [Citation16,Citation25,Citation26,Citation33,Citation35]. This suggests that the species is either polyphagic, or that there are several closely related species that have emerged after radiation in marine diatoms as a consequence of an infection trait innovation [Citation36]. As M. islandica was not seen to infect Pseudo-nitzschia spp. also present in the same plankton sample, even after prolonged incubation, the latter case seems more plausible.
Miracula islandica, with its roundish, simple holocarpic thallus resembles species classified in the genera Diatomophthora and Ectrogella (e.g., E. licmophorae, E. gomphonematis) [Citation14,Citation23,Citation33]. However, the morphology of its discharge tube and the mode of zoospores release hinted at its placement in Miracula as supported by molecular phylogenetic evidence. If other species with a similar zoospore release pattern and discharge tube development, for example E. bacillariacearum sensu Scherffel [Citation14], E. monostoma, and E. gomphonematis, also belong to Miracula needs to be clarified in future studies.
The diatom Thalassiosira s. lat. (including Minidiscus) to our knowledge has not been reported as host of an obligate holocarpic oomycete parasite before, but to various other marine eukaryotic epibiotic (e.g., Chytridiomycota, Aphelidae, Cercozoa) and endobiotic pathogens (e.g., Pirsonia) [Citation37–41]. As members of the host genus in a broad sense are widely studied as model diatom species [Citation42], the discovery of an oomycete pathogen in this group could also open up some opportunities to further investigations into the biology and molecular mechanisms of oomycete-diatom interactions in a genetically tractable system. However, for this axenic dual cultures would need to be established in the future, as already done for some other holocarpic parasitoids of diatoms (Lagenisma coscinodisci, Diatomophthora perforans subsp. destruens, D. perforans subsp. pleurosigmae) [Citation23,Citation32].
Acknowledgment
A travel grant by DAAD that seeded the collaboration that led to the current study is gratefully acknowledged.
Disclosure statement
No potential conflict of interest was reported by the author(s).
Additional information
Funding
Notes
† According to a recent suggestion by Thines et al. [Citation1], all scientific names are set in italics, regardless of their taxonomic rank.
References
- Thines M, Aoki T, Crous PW, et al. Setting scientific names at all taxonomic ranks in italics facilitates their quick recognition in scientific papers. IMA Fungus. 2020;11(1):1–5.
- Raghukumar S. Ecology of the marine protists, the Labyrinthulomycetes (thraustochytrids and labyrinthulids). Eur J Protistol. 2002;38(2):127–145.
- Neuhauser S, Kirchmair M, Gleason FH. The ecological potentials of Phytomyxea (“plasmodiophorids”) in aquatic food webs. Hydrobiologia. 2011;659(1):23–35.
- Bennett RM, Honda D, Beakes GW, et al. Labyrinthulomycota. In: Archibald JM, Simpson AGB, Slamovits C, editors. Handbook of the protists. Cham (Switzerland): Springer; 2017. p. 507–542.
- Beakes GW, Thines M. Hyphochytriomycota and Oomycota. In: Archibald JM, Simpson AGB, Slamovits C, editors. Handbook of the protists. Cham (Switzerland): Springer; 2017. p. 435–505.
- Hanic LA, Sekimoto S, Bates SS. Oomycete and chytrid infections of the marine diatom Pseudo-nitzschia pungens (Bacillariophyceae) from Prince Edward Island, Canada. Can J Bot. 2009;87(11):1096–1105.
- Scholz B, Guillou L, Marano AV, et al. Zoosporic parasites infecting marine diatoms - a black box that needs to be opened. Fungal Ecol. 2016;19:59–76.
- Buaya AT, Thines M. An overview on the biology and phylogeny of the early-diverging oomycetes. Philipp J Syst Biol. 2020;14:1–20.
- Sparrow FK. Aquatic Phycomycetes. Ann Arbor (MI): The University of Michigan Press; 1960.
- Karling JS. The simple holocarpic biflagellate Phycomycetes. New York (NY): Published by Karling JS; 1942.
- Dick MW. Straminipilous fungi. Amsterdam (The Netherlands): Kluwer; 2001.
- Zopf W. Zur Kenntniss der Phycomyceten. I. Zur Morphologie und Biologie der Ancylisteen und Chytridiaceen. Nova Acta Academiae Caesareae Leopoldino-Carolinae Germanicae Naturae Curiosorum. 1884;47:143–236.
- Zopf W. Über einem neuen parasitischen. Phycomyceten Mitt V Prov Brandenburg. 1878;20(7):77.
- Scherffel A. Endophytische phycomyceten-parasiten der bacillariaceen und einige neue monadinen. Ein beitrag zur phylogenie der oomyceten (schroter). Arch Protistenk. 1925;52:1–141.
- Drebes G. Ein parasitischer phycomycet (Lagenidiales) in Coscinodiscus. Helgolander Wiss Meeresunters. 1966;13(4):426–435.
- Buaya AT, Ploch S, Hanic L, et al. Phylogeny of Miracula helgolandica gen. et sp. nov. and Olpidiopsis drebesii sp. nov. two basal oomycete parasitoids of marine diatoms, with notes on the taxonomy of Ectrogella-like species. Mycol Prog. 2017;16(11-12):1041–1050.
- Buaya AT, Ploch S, Thines M. Rediscovery and phylogenetic placement of Olpidiopsis gillii (de Wildeman) Friedmann, a holocarpic oomycete parasitoid of freshwater diatoms. Mycoscience. 2019;60(3):141–146.
- Buaya AT, Thines M. Miracula moenusica, a new member of the holocarpic parasitoid genus from the invasive freshwater diatom Pleurosira laevis. FUSE. 2019;3:19–33.
- Buaya AT, Thines M. Diatomophthoraceae - a new family of olpidiopsis-like diatom parasitoids largely unrelated to Ectrogella. Fungal Syst Evol. 2020;5:113–118.
- Feldmann J, Feldmann G. Observations sur quelques Phycomycetes marins nouveaux où peu connus. Revue Mycologique. 1955;20:231–251.
- Johnson TW. Monocentric fungi on species of Rhizosolenia from saline habitats. Mycopathol Mycol Appl. 1967;32(4):281–290.
- Thines M, Nam B, Nigrelli L, et al. The diatom parasite Lagenisma coscinodisci (Lagenismatales, Oomycota) is an early diverging lineage of the Saprolegniomycetes. Mycol Prog. 2015;14:75.
- Buaya AT, Ploch S, Kraberg A, et al. Phylogeny and cultivation of the holocarpic oomycete Diatomophthora perforans comb. nov., an endoparasitoid of marine diatoms. Mycol Prog. 2020;19(5):441–454.
- Hassett BT, Thines M, Buaya AT, et al. A glimpse into the biogeography, seasonality, and ecological functions of arctic marine Oomycota. IMA Fungus. 2019;10:6.
- Garvetto A, Perrineau M-M, Dressler-Allame M, et al. “Ectrogella” parasitoids of the Diatom licmophora sp. are polyphyletic. J Eukaryot Microbiol. 2020;67(1):18–27.
- Garvetto A, Nézan E, Badis Y, et al. Novel widespread marine oomycetes parasitising diatoms, including the toxic genus Pseudo-nitzschia: genetic, morphological, and ecological characterisation. Front Microbiol. 2018;9(2918):1–19.
- Guillard RR, Ryther JH. Studies of marine planktonic diatoms. I. Cyclotella nana Hustedt, and Detonula confervacea (cleve) Gran. Can J Microbiol. 1962;8:229–239.
- Wang Y, Tian RM, Gao ZM, et al. Optimal eukaryotic 18S and universal 16S/18S ribosomal RNA primers and their application in a study of symbiosis. PLoS One. 2014;9(3):e90053.
- Katoh K, Standley DM. MAFFT multiple sequence alignment software version 7: improvements in performance and usability. Mol Biol Evol. 2013;30(4):772–780.
- Ronquist F, Teslenko M, van der Mark P, et al. MrBayes 3.2: efficient Bayesian phylogenetic inference and model choice across a large model space. Syst Biol. 2012;61(3):539–542.
- Kumar S, Stecher G, Tamura K. MEGA7: Molecular Evolutionary Genetics Analysis Version 7.0 for bigger datasets. Mol Biol Evol. 2016;33(7):1870–1874.
- Buaya AT, Kraberg A, Thines M. Dual culture of the oomycete Lagenisma coscinodisci Drebes and Coscinodiscus diatoms as a model for plankton/parasite interactions. Helgo Mar Res. 2019;73(92):2.
- Medlin LK, Metfies K, Mehl H, et al. Picoeukaryotic plankton diversity at the Helgoland time series site as assessed by three molecular methods. Microb Ecol. 2006;52(1):53–71.
- Petersen HE. Contributions a la connaissance des Phycomycetes marins (Chytridineae Fischer). Oversigt over Det Kongelige Danske Videnskabernes Selskabs Forhandlinger. 1905;5:188–439.
- Kok SP, Kikuchi T, Toda T, et al. Diversity and community dynamics of protistan microplankton in Sagami Bay revealed by 18S rRNA gene clone analysis. Plankton Benthos Res. 2012;7(2):75–86.
- Thines M. An evolutionary framework for host shifts - jumping ships for survival. New Phytol. 2019;224(2):605–617.
- Gaertner A. Some fungal parasites found in the diatom populations of the Rosfjord area (South Norway) during March 1979. Veröff Inst Meeresforsch Bremerh. 1979;18:29–33.
- Schweikert M, Schnepf E. Electron microscopical observations on Pseudoaphelidium drebesii Schweikert and Schnepf, a parasite of the centric diatom Thalassiosira punctigera. Protoplasma. 1997;199(3-4):113–123.
- Schnepf E, Kuhn SF. Food uptake and fine structure of Cryothecomonas longipes spec. nov., a marine nanoflagellate incertae sedis feeding phagotrophically on large diatoms. Helgol Mar Res. 2000;54(1):18–32.
- Gutiérrez MH, Jara AM, Pantoja S. Fungal parasites infect marine diatoms in the upwelling ecosystem of the Humboldt current system off central Chile. Environ Microbiol. 2016;18(5):1646–1653.
- Guinder VA, Carcedo CM, Buzzi N, et al. Ephemeral parasitism on blooming diatoms in a temperate estuary. Mar Freshwater Res. 2018;69(1):128–133.
- Armbrust EV, Berges JA, Bowler C, et al. The genome of the diatom Thalassiosira pseudonana: ecology, evolution, and metabolism. Science. 2004;306(5693):79–86.