Abstract
The fungal-like family Saprolegniaceae (Oomycota), also called “water mold,” includes mostly aquatic saprophytes as well as notorious aquatic animal pathogens. Most studies on Saprolegniaceae have been biased toward pathogenic species that are important to aquaculture rather than saprotrophic species, despite the latter’s crucial roles in carbon cycling of freshwater ecosystems. Few attempts have been made to study the diversity and ecology of Saprolegniaceae; thus, their ecological role is not well-known. During a survey of oomycetes between 2016 and 2021, we investigated the diversity and distribution of culturable Saprolegniaceae species in freshwater ecosystems of Korea. In the present study, members of Saprolegniaceae were isolated and identified at species level based on their cultural, morphological, and molecular phylogenetic analyses. Furthermore, substrate preference and seasonal dynamics for each were examined. Most of the species were previously reported as animal pathogens; however, in the present study, they were often isolated from other freshwater substrates, such as plant debris, algae, water, and soil sediment. The relative abundance of Saprolegniaceae was higher in the cold to cool season than that in the warm to hot season of Korea. This study enhances our understanding of the diversity and ecological attributes of Saprolegniaceae in freshwater ecosystems.
1. Introduction
Members of Oomycota (kingdom Straminipila) were previously classified under the kingdom Fungi, with which they share several similar traits, such as osmotrophic nutrition, conidia formation, host-infection strategy, and mycelial growth. However, it was revealed recently that these traits are indeed a result of convergent evolution; hence, oomycetes are often called “fungus-like organism” or “pseudo-fungi” [Citation1,Citation2]. Saprolegniales is a monophyletic order of oomycetes and entirely depends on water, thus often called “water mold.” This order has evolved both pathogenic and saprophytic lifestyles and consists of three families: Achlyaceae, Saprolegniaceae, and Verrucalvaceae [Citation3–6].
Saprolegniaceae is characterized by fusiform, filiform, or irregular sporangia; centric or subcentric oospores; and androgynous, monoclinous, diclinous, hypogynous, or epigynous antheridia [Citation3,Citation6]. This family has been reported to inhabit aquatic environments, where many members act as important decomposers that behave as saprophytes on animal and plant debris [Citation7–10]; however, some are notorious pathogens that are responsible for high-profile declines in animal wildlife and aquaculture populations [Citation11,Citation12].
Saprolegnia, the largest genus of the Saprolegniaceae family, comprising approximately 25 species [Citation13–15], is widely distributed throughout the world and possesses pathogenic species that attack the embryonic and adult stages of fishes [Citation16–18] and amphibians [Citation19–21]. In Korea, there have been few reports of infections associated with Saprolegnia parasitica in live fishes and their eggs [Citation22,Citation23] and S. ferax in the Asiatic toad (Bufo gargarizans) eggs [Citation24]. However, the latter species has only been identified by BLASTn searches for the internal transcribed spacer (ITS) rDNA sequence in GenBank, without a detailed morphological description. Therefore, the present study officially records this taxon based on morphological analysis combined with molecular phylogenetic analysis.
Leptolegnia is a well-known parasite in invertebrates and has been researched for its potential as a biological control agent for mosquitoes [Citation25]. Other members of the genus Leptolegnia have been reported to be associated with fishes, amphibians, and cladocerans [Citation26,Citation27]. Filamentous sporangia, elongated spores, and oogonium filled with spherical oospores are characteristics that differentiate Leptolegnia from other genera of Saprolegniaceae [Citation28,Citation29]. This genus was first established by de Bary [Citation28] based on Leptolegnia caudata. It has been reported in Europe [Citation28,Citation30,Citation31], America [Citation26,Citation29,Citation32], Oceania [Citation33], and Asia [Citation28] but was previously unrecorded in Korea.
Geolegnia, introduced by Harvey [Citation34] is characterized by the absence of flagella [Citation35], a unique trait within oomycetes. Compared with other members of Saprolegniaceae, this genus seems to have a high preference for edaphic environments; all Geolegnia species have been found in soils [Citation13,Citation34,Citation36], except for Geolegnia helicoides that was isolated from mosquito larvae in freshwater environments [Citation35], but has not been reported as an aquatic pathogen. To our knowledge, this genus has been previously reported mainly in Europe [Citation36] and America [Citation34,Citation35], but was not recorded in Asia.
Recent diversity and ecological studies of oomycetes have largely been biased toward economically important plant pathogens, such as Peronosporales [Citation37–39], whereas aquatic oomycetes have failed to gain appropriate attention [Citation40,Citation41]. Between 2016 and 2021, numerous oomycete isolates were collected from diverse freshwater sub-locations, such as water, soil sediment, algae, plants, and animal debris in rivers, ponds, or mountain streams, in Korea. Consequently, members of Achlyaceae [Citation7], Peronosporaceae [Citation42], and Pythiaceae [Citation43] were recently revealed. In the present study, we analyzed the diversity and ecology of Saprolegniaceae, which accounted for 13% of the collection. First, we aimed to clarify the identity of Saprolegniaceae species based on cultural, morphological, and molecular phylogenetic analyses. Next, we examined their substrate preferences and seasonal distributions. This study contributes to our knowledge on the diversity and ecology of Saprolegniaceae in freshwater environments.
2. Materials and methods
2.1. Oomycete isolates
Between 2016 and 2021, oomycete samples were collected from diverse freshwater sub-locations in Korea, including water, soil sediment, plant debris, moss, algae, fish, and snails in rivers, ponds, and mountain streams. The sampling site locations are shown in , and the obtained sampling information is listed in . To isolate the oomycete strains from fish, shrimp, and snails, they were washed with distilled water three times and placed on potato dextrose agar (PDA; Difco, Detroit, MI, USA) and V8 agar (V8A; 200 mL clarified V8 juice, 10 g CaCO3, 15 g agar, 800 mL deionized water). A simple plating technique was used for freshwater and soil sediments. The samples were distributed throughout thin layers of PDA and V8A agar plates. For plant debris, like leaves, stems, and twigs, the samples were washed three times with distilled water [Citation43,Citation44] and cut into 3–5 mm2 sections before placing them on the two types of solid growth media. Bacterial and fungal growth were suppressed by adding 15 ppm rifampicin and 20 ppm nystatin to the medium. After 48 h, hyphal tips were isolated and transferred onto a new agar plate. Representative isolates were deposited in the Culture Collection of the Nakdonggang National Institute of Biological Resources (NNIBR, Sangju, South Korea).
Figure 1. Location of the 34 sampling sites (red dot) in South Korea. The map was created using QGIS v.3.24.
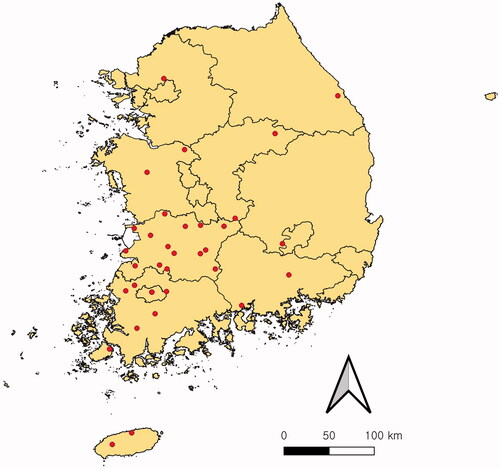
Table 1. Collection details and GenBank accession number for Saprolegniaceae isolates investigated in this study.
2.2. Morphological analysis
Cultural characteristics were investigated three days after inoculating the isolates onto PDA, V8A, and corn meal agar (CMA; Difco) at 25 °C in the dark. After a week, a morphological investigation was performed under a Zeiss Axio Imager A2 microscope (Carl Zeiss, Oberkochen, Germany). All the taxonomically meaningful structures were identified and subsequently photographed using an Axiocam 512 color camera (Carl Zeiss).
2.3. Molecular phylogenetic analysis
Genomic DNA was extracted from the mycelia using the MagListo 5M plant Genomic DNA Extraction Kit (Bioneer, Daejeon, South Korea), according to the manufacturer’s instructions. The ITS rDNA, cytochrome c oxidase subunit I (cox1), and cytochrome c oxidase subunit II (cox2) mtDNA regions were amplified by PCR (polymerase chain reaction) with the following primer pairs ITS1/ITS4 [Citation45], OomCox1-levup/OomCox1-levlo [Citation46], and cox2-F [Citation47]/cox2-RC4 [Citation48], respectively. PCR amplicons were sequenced by Macrogen Inc. (Seoul, South Korea). They were subjected to the NCBI BLAST search to identify related sequences in GenBank; sequences similar to those of the PCR amplicons were downloaded. In addition, previously published sequences of authentic isolates of Geolegnia, Leptolegnia, and Saprolegnia species were retrieved from GenBank. All sequences were aligned using the Q-INS-i algorithm [Citation49] of MAFFT 7 [Citation50]. Phylogenetic analysis was performed using MEGA X [Citation51] for minimum evolution (ME) and maximum-likelihood (ML) inferences using the Tamura–Nei model with 1000 bootstrap (BS) replicates. For multilocus phylogenetic analysis based on a combined dataset of cox1 and cox2, SequenceMatrix v1.7.8 [Citation52] was used to concatenate the sequences of individual markers. Subsequently, a multilocus phylogenetic tree was constructed as described above.
2.4. Statistical analysis of seasonal variation in abundance and composition
The monthly mean air and water temperatures in the sampling area were provided by the Korean Meteorological Administration (Weather Data Portal; https://data.kma.go.kr) and the National Institute of Environmental Research (Water Environment Information System; http://water.nier.go.kr). Seasonal richness and diversity of Saprolegniaceae were evaluated using PAST 4.03 [Citation53]. Analyses of similarity (ANOSIM) on Bray-Curtis and Jaccard distances were conducted using PRIMER-E 7.0.17 [Citation54] and PAST 4.03 to compare Saprolegniaceae structures among different seasons.
3. Results
3.1. Morphological analysis
The colonies of the Geolegnia, Leptolegnia, and Saprolegnia isolates were colorless and formed a radiating pattern with short and dense aerial mycelia on PDA at 25 °C (). On the other hand, they exhibited submerged growth, with sparse mycelia on both the V8A and CMA. The sporangia of G. helicoides were cylindrical or fusiform, straight, or bent, while those of L. caudata were filamentous (). In Saprolegnia species, the sporangia were cylindrical, clavate, or fusiform. Oogonia were found to be spherical to subspherical and contained one or more oospores that were mostly centric or subcentric. However, sporangia, oogonia, and oospores varied in size with species. The taxonomic description below provides detailed morphological features that define the individual species.
Figure 2. Cultural characteristics of Saprolegniaceae isolates obtained from this study. Geolegnia helicoides W732 (A); Leptolegnia caudata W1297 (B); Saprolegnia aenigmatica W1247 (C); Saprolegnia diclina W724 (D); Saprolegnia ferax W956 (E) on PDA (1, 2), V8A (3, 4), CMA (5, 6), after 72 h at 25 °C (1, 3, 5: observed view and 2, 4, 6: reverse view).
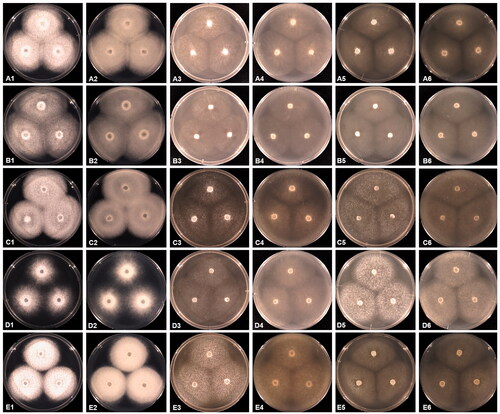
Figure 3. Morphological characteristics of Saprolegniaceae isolates obtained from the present study. Microscopic structures observed under a microscope: filiform sporangium (A) and oospore contenting finely granular (K) of Geolegnia helicoides W732, filamentous sporangium (B) of Leptolegnia caudata W1297, clavate sporangium of Saprolegnia aenigmatica W1247 (C), and S. ferax W956 (E) cylindrical sporangium (D), oogonia (G, H) and oogonia with centric oospores (L) of S. diclina W724, pyriform gemmae (F) and oogonia (J) of S. ferax W956, pyriform oogonia of S. aenigmatica W1247 (I) (scale bars: A–D, F, G, I, J, L = 20 μm, E, H, K = 10 μm).
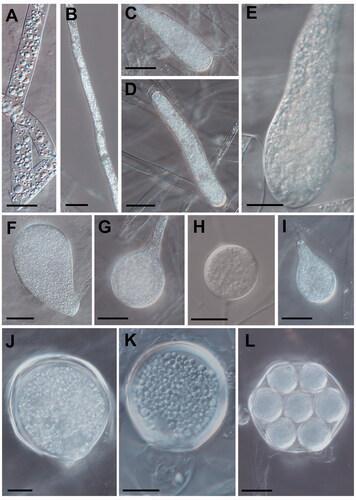
3.2. Molecular phylogenetic analysis
ITS rDNA, cox1, and cox2 mtDNA sequences were used to verify the morphology-based identification and infer the phylogenetic relationships between the Korean and authentic isolates of Geolegnia, Leptolegnia, and Saprolegnia species. In the ITS-based tree (), the Korean strains formed a well-supported group with G. helicoides, L. caudata, S. aenigmatica, S. asterophora, S. australis, S. diclina, S. ferax, S. parasitica, and S. torulosa, with the maximum supporting value in the ME and ML analyses. However, nine Saprolegnia strains, namely W54, W453, W455, W689, W690, W696, W698, W699, and W1957, clustered within a complex group containing two different species, S. delica and S. salmonis; to disentangle this at the species level, phylogenetic analysis using two mitochondrial markers, cox1 and cox2, was performed. In the multilocus tree based on cox1 and cox2 genes (), the strains W453, W455, W689, W690, W696, W698, and W699 were revealed to be closest to S. delica, with a high BS value of 99% in the ME and ML analyses. W54 was separated from the S. delica group, including strain CBS34462. The cox1 and cox2 sequences of S. salmonis are not available in the public databases. The cox2 sequence for S. delica, too, was unavailable in GenBank, and hence just the cox1 sequence (strain CBS34462) was used to analyze its phylogenetic position. A BLASTn search revealed that the ITS sequence of the strain W732 shared a high sequence similarity (99.9% (672/673 bp)) with the ex-type strain of G. helicoides (LPS48465). The strains of Leptolegnia (W307, W328, W1295, W1297, W1301, W1302, and W2018) exhibited 100% (641/641 bp) sequence similarity with L. caudata (CBS68069). The Korean strains 20F-1229, W1765, and W1055 were tagged as “new species” of Saprolegniaceae. While the 20F-1229 and W1765 strains were found to be closest to the Saprolegnia sp. JM59 (MT473826), the W1055 strain was found to be related to two unknown isolates of the Saprolegniaceae (GenBank numbers, AM947031 and KF748559).
Figure 4. Phylogenetic tree of Saprolegniaceae species from the minimum evolution analysis based on the internal transcribed spacer (ITS) rDNA sequences. The large branches of Saprolegnia ferax and S. diclina were compressed. Multi-gene phylogenetic tree in a blue box was inferred using the partial cytochrome c oxidase subunit I (cox1), and cytochrome c oxidase subunit II (cox2) mtDNA sequences of taxa in a green box of the ITS tree. Bootstrapping values (minimum evolution BS/maximum-likelihood BS) higher than 70% were given above or below the branches (1000 replicates). Aphanomyces laevis was used as outgroup. The strains isolated in Korea are shown in bold. The scale bar equals the number of nucleotide substitutions per site.
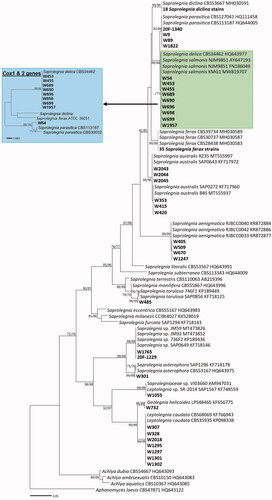
3.3. Substrate preference
A total of 89 isolates assigned to the family Saprolegniaceae were obtained from diverse freshwater substrates, including water, soil sediment, algae, fish, and snails, with plant substrates, such as decaying leaves, stems, and twigs, being the dominant isolation sources (56%; ). All Saprolegniaceae species were isolated from plant sources, except S. australis which held its roots in fishes (Oryzias sp.) and snails (Semisulcospira spp.). Saprolegnia diclina and S. ferax have also been isolated from animal sources, while G. helicoides, L. caudata, S. asterophora, S. salmonis, and S. torulosa were found only in decaying plants. In contrast, S. parasitica and S. ferax were isolated from diverse substrates, except for soil sediment. S. aenigmatica and S. delica were isolated from the soil sediment. Further, S. aenigmatica, S. delica, S. diclina, S. parasitica, and S. ferax were found in the water as well.
3.4. Seasonal abundance and composition
The abundance of Saprolegniaceae differed with season. In the cold to cool (CC) season (January–April) of Korea, the monthly mean air and water temperatures at the sampling sites were below 15 °C (average water temperature: 9 °C, air temperature: 7 °C), whereas in the warm to hot (WH) season (May–September), it crossed above 15 °C (average water temperature: 23 °C, air temperature: 22 °C). Consequently, the relative abundance of Saprolegniaceae was higher in the CC season than that the WH season (): 58 isolates (65%) in CC vs. 31 isolates (35%) in WH season. A total of nine Saprolegniaceae species, including two unknown species, were found in the CC season, of which S. ferax was predominant one (48%) followed by S. diclina (31%). In the WH season, six species were isolated, of which L. caudata and S. ferax were the most prevalent species (23%). Fisher’s alpha diversity (3 vs. 2.2) and Chao1 (11 vs. 6) richness were higher in the CC than in the WH season. However, the Shannon (1.4 vs. 1.7) and Simpson (0.7 vs. 0.8) indexes were lower in the CC than in the WH season. In the CC season, the dominance rates of S. ferax and S. diclina are very high. Geolegnia helicoides, S. parasitica, S. salmonis, S. torulosa, and two unknown species of Saprolegniaceae were isolated exclusively in the CC season, whereas L. caudata, S. aenigmatica, S. asterophora, and S. australis were exclusive to the WH season. Three species of Saprolegnia – S. delica, S. diclina, and S. ferax – were ubiquitous in both the seasons. However, there was a difference in the overall abundance and distribution of Saprolegniaceae between the two seasons. A statistically significant difference in the structure of Saprolegniaceae between the two seasons was found by ANOSIM based on both the abundance data computed with Bray–Curtis distances and the presence/absence data computed with Jaccard distances (R = 0.1, p < 0.01).
4. Taxonomy
Geolegnia helicoides Steciow, E. Lara, Belbahri, Pillonel & Pelizza, IMA Fungus 4(2): 170 (2013) [MB#805937]
Description: Colonies growing colorlessly, with a radiate pattern with short and dense aerial mycelia on PDA, but with sparse mycelia on V8A and CMA. Main hyphae straight or sinuous and up to 25 µm wide. Sporangia cylindrical to broadly filiform or fusiform, straight or curved, and 10–20 × 150–800 µm. Zoospores non-motile, ellipsoid, fusiform or cylindrical, forming in a single row and 25–30 × 10–18 µm. Oospore hyaline, spherical or ellipsoidal, with finely granular contents, and 20–40 µm in diameter. Oogonia terminal or lateral, spherical or pyriform, and 25–45 µm in diameter. Antheridial branches slender, abundantly branched, mainly diclinous, and forming helicoidal spirals.
Isolate examined: Korea, Jeollanam-do; Yeongam-gun; Gunseo-myeon (34°44′48″N, 126°39′45″E), ex a decaying moss in freshwater, March 14 2019, B. Nam and Y.-J. Choi (W732 = NNIBRFG21808).
Note: Isolate W732 was found to be morphologically and phylogenetically identical to the ex-holotype of G. helicoides (LPS48465) [Citation35]. Considering the fact that this species was initially isolated from mosquito larvae living in water-holding tanks at a river and that the Korean isolate W732 was isolated from decaying moss in a mountain stream, it seems likely for them to have a strong substrate preference for freshwater. Geolegnia was introduced by Harvey [Citation34], and it is distinguished from the other genera of Saprolegniaceae by unique characteristics of sporangia and spores (cylindrical to filiform sporangia and non-motile zoospores). Compared with other Saprolegniaceae members, Geolegnia species (G. inflata, G. intermedia, G. septisporangia) have been isolated from edaphic environments [Citation13,Citation34,Citation36]; only G. helicoides has been found in freshwater environments [Citation35]. The antheridial coiled around the main hyphae is a key morphological characteristic of G. helicoides [Citation35]. To date, this genus has been reported in Europe [Citation36] and America [Citation34,Citation35]. To the best of our knowledge, the present study is the first report of the genus Geolegnia in Asia.
Leptolegnia caudata de Bary, Botanische Zeitung 46: 609 (1888)
[MB#212440]
Description: Mycelium moderately dense. Hyphae slender, sparingly to moderately branched. Sporangia filamentous, usually unbranched, sometimes branched, and 200–950 × 15–19 µm. Zoospores dimorphic, behavior leptolegnoid and 12–14 µm in diameter. Oogonia usually lateral, sometimes terminal, spherical or subspherical, and 25–45 µm in diameter. Oogonial wall unpitted, smooth, often extended into short beak at the connected part to antheridial cells. Oospores spherical in an oogonium and 22–40 µm in diameter. Antheridial branches slender, unbranched or sometimes branched. Antheridial cells clavate, bent, and apically attached.
Isolate examined: Korea, Jeollabuk-do; Iksan-si; Yongdong-myeon (36°06′26.5″N, 126°59′31.7″E), ex a decaying plant leaf in freshwater, June 19 2020, B. Nam and Y.-J. Choi (W1297 = NNIBRFG31700). See for other material examined.
Note: Morphological and sequence characteristics of seven Korean isolates (W307, W328, W1295, W1297, W1301, W1302, and W2018) matched those of L. caudata (CBS68069), the type species of the genus Leptolegnia. Since its first description in mountain lakes in Germany by de Bary [Citation28], this species has been reported in diverse freshwater environments in association with algae [Citation29], amphibians [Citation26,Citation55], crustaceans [Citation56], fish eggs [Citation31], and mosquito larvae [Citation57,Citation58]. Thus, the recovery of the Korean isolates from decaying leaves in a reservoir is not surprising. de Bary [Citation28] established the genus Leptolegnia based on L. caudata. Their sporangia are similar to those of Aphanomyces (filamentous), but the zoospores are motile and elongated like those of Saprolegnia with the spherical oospores completely filling the oogonium. These characteristics differentiate Leptolegnia from other Saprolegniaceae. Leptolegnia is widely distributed in Europe [Citation28,Citation30,Citation31], America [Citation26,Citation29,Citation32], and Asia [Citation33], but was not previously recorded in Korea.
Saprolegnia aenigmatica Sandoval-Sierra and Diéguez-Uribeondo, PloS ONE 10(7): e0132999: 9 (2015) [MB#812742]
Description: Mycelium delicate. Hyphae slender, smooth and moderately branched. Gemmae catenulate, spherical, or pyriform, often terminal and generally branched. Sporangia clavate, sometimes filiform and 105–220 × 18–24 µm. Mostly terminal and renewal by proliferation with an apical papilla before zoospore discharge. Zoospore primary pyriform, discharge saprolegnoid, secondary reniform, and lateral biflagellate. Cysts spherical and 12–13 µm in diameter. Oogonia globose, pyriform and rarely forming short moniliform chains, and 60–118 × 47–79 µm. Oogonial wall unpitted, except points of attachment of antheridial cells. Oogonia axillary, terminal or intercalary. Oospores centric, globose to subglobose, filling the oogonium, sometimes do not filling the oogonium and 23–27 µm in diameter. Antheridial branches well developed and diclinous. Antheridia numerous, tubular to ampullaceous, and often encircling the oogonia, sometimes branched.
Isolate examined: Korea, Chungcheongnam-do; Yesan-gun; Daeheung-myeon (36°36′03.5″N, 126°46′54.3″E), ex soil sediment in freshwater, May 19 2017, B. Nam and Y.-J. Choi (W509 = CNFG_3196). See for other material examined.
Note: Sandoval-Sierra and Diéguez-Uribeondo [Citation15] recovered S. aenigmatica from the eggs of fishes, adult fishes themselves, and amphibians in freshwater, which hints toward their pathogenicity in aquatic animals. The Korean isolates (W405, W509, W670, and W1247) are consistent with the morphological and phylogenetic descriptions of S. aenigmatica, but differ in their sources of isolation; the Korean isolates were recovered from diverse substrates in reservoirs, rivers, and mountain streams, such as soil sediments, decaying mosses, and leaves. This species has been recorded in North and South America and Europe [Citation15]. To the best of our knowledge, this is the first record of S. aenigmatica in Asia.
Saprolegnia asterophora de Bary, Jahrbücher für Wissenschaftliche Botanik 2: 189 (1860)
[MB#242512]
Description: Mycelium diffuse. Hyphae thin flaccid or flexuous. Sporangia clavate or fusiform, renewed internally and 120–820 × 12–40 µm. Spores dimorphic, discharge and behavior saprolegnoid. Primary spore cysts 12–14 µm in diameter. Gemmae sparse, clavate or irregular, terminal or intercalary. Oogonia lateral, sometimes terminal, rarely intercalary, spherical, subspherical, oval or obpyriform and 30–65 µm in diameter, inclusive of wall ornamentations. Oogonial wall unpitted, densely papillate, or provided with papillae tuberculate projections. Oogonial stalks straight, branched, unbranched or irregular. Oospores subcentric, spherical to subspherical. 1–2(–6) oospores per oogonium, usually not filling it and (17–)25–33(–45) µm in diameter, and germination not observed. Antheridial branches androgynous, rarely monoclinous. Antheridial cells simple, short-clavate or tuberous, apically or laterally appressed, persisting and fertilization tubes present or absent, not persisting.
Isolate examined: Korea, Jeollanam-do; Damyang-gun; Yong-myeon (35°27′11.2″N, 127°01′08.7″E), ex a decaying tree twig in freshwater, August 3 2016, B. Nam and Y.-J. Choi (W301 = CNFG_3197). See for other material examined.
Note: Isolate W301 was morphologically and phylogenetically identical to isolate CBS53167 of S. asterophora. Since de Bary [Citation59] initially found this species in water, it has been isolated from diverse substrates in freshwater, such as water, soil [Citation14,Citation60,Citation61], fishes [Citation56], and their eggs [Citation62]. The Korean isolate was isolated from decaying twigs in a reservoir.
Saprolegnia australis R.F. Elliott, New Zealand Journal of Botany 6: 103 (1968)
[MB#338787]
Description: Mycelium dense and diffuse. Hyphae slender or stout. Sporangia cylindrical, renewed internally, primary ones 250 × 32 µm, secondary ones usually shorter, up to 600 µm long. Gemmae abundant, clavate, terminal or intercalary, and usually single. Oogonia terminal, lateral or intercalary, when intercalary 59–80 µm in diameter. Oogonial stalks in length, unbranched, straight, curved, twisted, or irregular. Oospores subcentric, spherical to subspherical. Four to 12 oospores per oogonium, usually not filling, and 22–27 µm in diameter. Antheridial branches, diclinous, monoclinous or androgynous. Antheridial cells simple or branched, persisting, tubular or attached in a digitated fashion.
Isolate examined: Korea, Jeollabuk-do, Gimje-si, Geumsan-myeon (35°43′07.5″N, 127°01′47.9″E), ex a snail in freshwater, September 22 2016, B. Nam and Y.-J. Choi (W415 = CNFG_3198). See for other material examined.
Note: Isolates W353, W415, W420, W2043, W2044, and W2045 were identified as S. australis, which was first described in freshwater by Elliott [Citation63]. Considering the high pathogenicity of crayfish [Citation64,Citation65], Prussian carp eggs (Carassius gibelio) [Citation66], and trout [Citation16,Citation17,Citation67,Citation68] and the present finding that S. australis has been attributed to saprolegniosis-infected rice fish (Oryzias sinensis) and a freshwater snail in Korea, this species could adversely affect the Korean aquaculture industry.
Saprolegnia delica Coker, The Saprolegniaceae, with notes on other water molds: 30 (1923)
[MB#262922]
Description: Mycelium delicate, lax, and hyphae much branched. Sporangia cylindrical, abundant in most young cultures, later irregularly inflated or bent. Gemmae plentiful or few, spherical, clavate, pyriform to fusiform, in moniliform chains. Oogonia terminating the main branches, typically spherical and 40–60 µm in diameter. Oogonial wall smooth and pitted, with few pits, centric, dark when young, and lighter at full maturity. Antheridial branches abundant, long and rambling. Antheridia present on nearly all the oogonia, diclinous. Antheridial tubes present and not inconspicuous.
Isolates examined: Korea, Jeollabuk-do, Gimje-si, Mangyeong-eup (35°51′08.3″N, 126°49′21.8″E), ex a decaying plant stem in freshwater, January 4 2017, B. Nam and Y.-J. Choi (W453 = CNFG_3199). See for other material examined.
Note: Isolates W453, W455, W689, W690, W696, W698, W699, and W1957 are morphologically and phylogenetically identical to S. delica (CBS34462). In the phylogenetic analysis, their ITS sequences were insufficient to distinguish them from S. salmonis, but cox1 and cox2 sequences can be identified at the species level. After S. delica was first described in the marsh by Coker [Citation69], it has often been reported to attack freshwater fishes [Citation16,Citation70]. The Korean isolates were isolated from water, soil sediments, algae, decaying plant leaves, and stems in reservoirs, mountain streams, and rivers.
Saprolegnia diclina Humphrey, Transactions of the American Philosophical Society 17: 109 (1892) [MB#146386]
Description: Mycelium sparingly to moderately branched. Sporangia cylindrical, clavate or fusiform, straight or slightly curved, and 80–1050 × 20–80 µm. Gemmae clavate, pyriform, obpyriform or cylindrical, terminal or intercalary and single or catenulate. Oogonia spherical, subspherical, obpyriform or irregular, terminal, lateral or intercalary, and spherical ones 30–130 µm in diameter and subspherical ones 55–145 × 18–70 µm. Oogonial wall pitted or unpitted, and pits occasionally inconspicuous. Oospores spherical, centric or subcentric, 12–45 µm in diameter. Antheridial branches diclinous, sometimes monoclinous, slender, and sparingly branched or unbranched. Antheridial cells simple, tubular, clavate, often irregular, and persisting or deliquescing.
Isolates examined: Korea, Jeollanam-do, Yeongam-gun, Gunseo-myeon (34°44′54″N, 126°39′41″E), ex a decaying plant leaf and shrimp in freshwater, March 14 2019, B. Nam and Y.-J. Choi (W724 = NNIBRFG21807). See for other material examined.
Note: Eighteen isolates (W724, W728, W730, W746, 20F-1228, 20F-1234, 20F-1235, 20F-1239, W1502, W1504, W1510, W1525, W1526, W1712, W1813, W1819, W1820, and W1854) were morphologically and phylogenetically identical to S. diclina (CBS53667), as described by Humphrey [Citation71]. This species has been implicated in significant oomycete infections in fish eggs [Citation16,Citation68] and amphibians [Citation19,Citation55]. The Korean isolates were found in water, decaying leaves, algae, mosses, and shrimps in mountain streams and rivers. To our knowledge, this is the first report of crustaceans inhabiting S. diclina.
Saprolegnia ferax (Gruith) Kütz., Phycologia generalis 35: 157 (1843)
[MB#146619]
Description: Hyphae stout and sparingly branched. Sporangia cylindrical, clavate, fusiform or irregular, straight or bent, and 30–620 × 18–65 µm. Gemmae filiform, pyriform or irregular, terminal or intercalary, single or catenulate. Zoosporangia filiform and renewed by internal proliferation. Zoospore discharge saprolegnoid. Oogonia spherical, pyriform or clavate, terminal or lateral, occasionally intercalary or catenulate, and 40–140 µm in diameter. Oogonial wall pitted, and often with basal in growth. Oospores spherical or ellipsoidal, centric or subcentric, and 20–30 µm in diameter. Antheridial cells tubular or clavate, sometimes irregular, usually persisting, and laterally appressed.
Isolates examined: Korea, Gwangju, Gwangsan-gu, Singa-dong (35°10′36.94″N, 126°50′15.04″E), ex freshwater, February 15 2016, H.B. Lee (EML-RIWB19 = CNFG_2017). See for other material examined.
Note: Saprolegnia ferax is ubiquitous in freshwater environments worldwide. Since its first description by Kützing [Citation72], it has been reported in various places including America, Asia, Australia, and Europe [Citation13]. This species is pathogenic to fishes [Citation16,Citation73] and amphibians [Citation21,Citation26,Citation55]. In Korea, this species was previously recorded as a pathogenic agent for Asiatic toad (Bufo gargarizans) eggs [Citation24], but identified using only ITS sequences without mycological description. Therefore, in the present study, we have provided detailed morphological characteristics combined with molecular phylogenetic analysis. The Korean isolates were morphologically identical to S. ferax according to the description by Thuret [Citation74] and phylogenetically identical to the authentic isolates CBS30537 [Citation46] and P1.5.14 [Citation48]. The isolates were found in water, algae, soil sediments, fishes, snails, mosses, decaying plant stems, and leaves in reservoirs, mountain streams, and rivers.
Saprolegnia salmonis Hussein & Hatai, Mycoscience 40 (5): 388 (1999)
[MB#459819]
Description: Hyphae delicate, slender and moderately branched. Sporangia clavate or straight, rarely curved, and 175–186 × 24–35 µm. Zoospore’s discharge saprolegnoid. Oogonia abundant, ovate, pyriform, mostly spherical ranging 50–95 µm in diameter, but often elongated as 85–150 × 50–70 µm, with or without antheridial attachment. Oogonial wall smooth and conspicuously pitted not only under the point of attachment of antheridial cell but also in absence of antheridial attachment. Oospores spherical, and centric or subcentric. Antheridial branches diclinous. Antheridial cells simple, tubular, delicate, not branched. Gemmae not observed.
Isolates examined: Korea, Gyeongsangbuk-do, Goryeong-gun, Gaejin-myeon (35°42′26.59″N, 128°21′23.40″E), ex a decaying plant stem in freshwater, March 26 2016, B. Nam and Y.-J. Choi (W54 = CNFG_2021). See for other material examined.
Note: Isolate W54 was morphologically and phylogenetically identical to S. salmonis (NJM 9851) described by Hussein et al. [Citation18]. It has been reported as a freshwater fish pathogen and was isolated from the sockeye salmon (Oncorhynchus nerka) [Citation18] and Atlantic salmon (Salmo salar) [Citation75]. The Korean isolate was obtained from a decaying plant stem in a river. In the phylogenetic analysis, the ITS sequences of the W54 strain and those of S. delica were indistinguishable; however, the cox1 and cox2 sequences discriminated it from S. delica. Morphologically, it was possible to distinguish them from their larger oogonia. Gemmae was not observed in S. salmonis but was abundantly produced in S. delica.
Saprolegnia torulosa de Bary, Botanische Zeitung 41: 56 (1883)
[MB#150224]
Description: Mycelium dense or sparse. Hyphae sparingly to moderately branched. Sporangia sparse or abundant, cylindrical, clavate, fusiform, and straight, curved, or bent, terminal or intercalary and moniliform, renewed internally, and 80–455 × 40–45 µm. Gemmae sparse or abundant, spherical, pyriform, obpyriform, cylindrical or clavate, and terminal or intercalary. Oogonia lateral, terminal or intercalary, spherical, subspherical, ovoid, obpyriform, or cylindrical, and (40–)50–65(–105) µm in diameter. Oogonial wall unpitted or with a few inconspicuous pits, and smooth. Oospores centric, spherical or ellipsoidal, and 12–35 µm in diameter. Antheridial branches diclinous, slender, sparingly branched or unbranched.
Isolates examined: Korea, Jeollabuk-do, Jangsu-gun, Cheoncheon-myeon (35°40′40.2″N, 127°28′39.1″E), ex a decaying plant stem in freshwater, March 16 2017, B. Nam and Y.-J. Choi (W485 = CNFG_3200). See for other material examined.
Note: Isolate W485 is morphologically and phylogenetically identical to S. torulosa (CBS110064) [Citation76]. It poses the greatest threat to the Atlantic salmon, rainbow, and brook trout farms [Citation77], but has also been reported to be involved in amphibian diseases [Citation78]. The Korean isolate was obtained from a decaying plant stem in a mountain stream.
5. Discussion
The ecological role of members of the Saprolegniaceae family in aquatic environments remains poorly understood compared with the true fungi that have been well established to dominate microbial decomposer communities in freshwater ecosystems [Citation79,Citation80]. This lack of knowledge is owing to the biased studies on pathogenic species causing economic damage to aquaculture. However, Saprolegniaceae species are widely distributed in freshwater ecosystems because of their diverse biological functions. In the present study, some Saprolegnia strains belonging to S. australis, S. diclina, and S. ferax were isolated from fishes and snails, but the main isolation sources were plant substrates, including decaying twigs, leaves, and stems. All Saprolegniaceae species were isolated from plant sources, except S. australis. In addition, algae, soil, and water were important isolation sources for S. aenigmatica, S. delica, S. diclina, S. ferax, and S. parasitica. These findings suggest that the freshwater ecosystem provides various habitats for Saprolegniaceae species. They may play a role in decomposing organic detritus related to biogeochemical cycles and food web dynamics in aquatic ecosystems. Further studies are necessary to understand their function in freshwater ecosystems.
Generally, Saprolegniaceae appears to be abundant at low temperatures. Previous case studies on their diversity and seasonality demonstrated that they are more abundant in cool temperatures, such as those observed during autumn, winter, and spring, than in hot temperatures in summer [Citation44,Citation81,Citation82]. The well-known “winter kill” syndrome in freshwater fish, caused by Saprolegnia [Citation83] also occurs in winter and early spring. Our results are in agreement with these studies. In the present study, the relative species abundance of Saprolegniaceae was higher in the CC season when the temperature was below 15 °C. S. ferax was isolated four times more in the CC season than in the WH season. Additionally, S. diclina, S. parasitica, S. salmonis, S. torulosa, and G. helicoides were found exclusively in the CC season. Moreover, the Chao1 richness index suggests that the taxa were undersampled in the CC season, as it estimated the proportion of missing species; thus, a higher diversity of Saprolegniaceae would be expected in the CC season. The abundance of oomycetes at low temperatures may be due to a dominant competition over True Fungi, another competent decomposer in the aquatic environment. In the CC season (below 15 °C) suitable for the growth of oomycetes, most of the Fungi have a remarkably slow growth rate, so oomycetes can easily dominate the aquatic environment. On the other hand, as the temperature rises above 15 °C, the dominance of oomycetes seems to decrease as the fungus dominates.
However, in the present study, L. caudata, S. aenigmatica, S. asterophora, and S. australis were observed only during the WH season. According to Liu et al. [Citation84] and Tandel et al. [Citation85], the optimum temperature for the growth of S. australis is 30 °C, and it cannot thrive at or below 7 °C. In the present study, S. australis was isolated from fishes and snails during the WH season (∼30 °C). A previous study also revealed that L. caudata grows slowly below 18 °C, and hence the optimum growth temperature ranges from 25 to 30 °C [Citation86]. L. caudata was found on decaying leaves only during the WH season. This species-specific seasonal distribution could be a crucial factor in determining the ecological patterns of the microbial community and may impact the entire aquatic ecosystem. It is particularly noteworthy that the WH season species obtained from this study are the pathogenic species of aquatic animals, such as fish [Citation17,Citation31,Citation65], frogs [Citation26,Citation55], shellfish [Citation56], and mosquitoes [Citation57,Citation58]. Recovering the WH season-species isolated in this study revealed the potential pathogenicity of Saprolegniaceae, which might impact warm freshwater ecosystems by infecting aquatic animals.
Temperature could be the most crucial factor determining the ecological dynamics of Saprolegniaceae; however, other environmental factors might also affect this community. Czeczuga et al. [Citation81,Citation87] revealed that the diversity of Saprolegniaceae is correlated with the physical and chemical properties of water, such as water hardness. Further studies on environmental influences are necessary to understand their ecological patterns in aquatic ecosystems. Environmental factors, including temperature, pH, and water flow, also have a significant impact on the pathogenicity of Saprolegniaceae [Citation11,Citation88]. The germination and colonization of pathogenic species in fish and fish eggs are mediated by environmental factors [Citation88]. However, even though it is pathogenic, Saprolegniaceae mostly infects immunocompromised or injured animals. In addition, the fitness of animal hosts is largely influenced by alterations in the environmental conditions. Diverse species of Saprolegniaceae are distributed in freshwater ecosystems, and they could pose a potential risk for the occurrence of aquatic animal diseases owing to optimal conditions for their pathogenesis. A relationship between climate change and Saprolegniaceae has also been reported. The epidemic caused by Saprolegnia is exacerbated by climate change as host fitness deteriorates [Citation89–91], in the sense that epidemic diseases caused by Saprolegniaceae are worsening due to global warming. Hence, future studies are warranted to further investigate the diversity, distribution, and pathogenicity of Saprolegniaceae and their correlation with environmental factors.
Overall, this study contributes toward the determination of the diversity and distribution of culturable Saprolegniaceae in freshwater ecosystems and identifies them at the species level based on cultural, morphological, and molecular phylogenetic analyses. Furthermore, substrate preferences and seasonal dynamics were investigated through long-term monitoring. This finding necessitates further studies on the role of aquatic Saprolegniaceae species. For example, they may play an essential role in biogeochemical cycles and food web dynamics, threatening the major biota that composes the healthy freshwater ecosystem under consideration.
Disclosure statement
No potential conflict of interest was reported by the author(s).
Additional information
Funding
References
- Dick MW. Straminipilous fungi: systematics of the peronosporomycetes including accounts of the marine straminipilous protists, the plasmodiophorids and similar organisms. 1st ed. Dordrecht: Kluwer Academic Publishers; 2001.
- Beakes GW, Thines M, Honda D. Straminipile “fungi” – taxonomy. eLS; 2015. p. 1–9.
- Beakes GW, Honda D, Thines M. 3 Systematics of the Straminipila: Labyrinthulomycota, Hyphochytriomycota, and Oomycota. In: McLaughlin DJ, Spatafora JW, editors. Systematics and evolution: part A. Berlin, Heidelberg: Springer Berlin Heidelberg; 2014. p. 39–97.
- Beakes GW, Thines M. Hyphochytriomycota and Oomycota. In: Archibald JM, Simpson AGB, Slamovits CH, editors. Handbook of the protists. Cham: Springer International Publishing; 2016. p. 1–71.
- Molloy DP, Glockling SL, Siegfried CA, et al. Aquastella gen. nov.: a new genus of saprolegniaceous oomycete rotifer parasites related to Aphanomyces, with unique sporangial outgrowths. Fungal Biol. 2014;118(7):544–558.
- Rocha SCO, Lopez-Lastra CC, Marano AV, et al. New phylogenetic insights into Saprolegniales (Oomycota, Straminipila) based upon studies of specimens isolated from Brazil and Argentina. Mycol Prog. 2018;17(6):691–700.
- Choi Y-J, Lee S-H, Nguyen TTT, et al. Characterization of Achlya americana and A. bisexualis (Saprolegniales, Oomycota) isolated from freshwater environments in Korea. Mycobiology. 2019;47(2):135–142.
- Czeczuga B, Mazalska B, Godlewska A, et al. Aquatic fungi growing on dead fragments of submerged plants. Limnologica. 2005;35(4):283–297.
- Paul B, Steciow MM. Saprolegnia multispora, a new oomycete isolated from water samples taken in a river in the Burgundian region of France. FEMS Microbiol Lett. 2004;237(2):393–398.
- Dinçtürk Ε, Tanrikul TT, Birincioğlu SS. First report of Saprolegnia parasitica from a marine species: gilthead seabream (Sparus aurata) in Brackish Water conditions. J Hellenic Vet Med Soc. 2019;70(2):1503–1510.
- van den Berg AH, McLaggan D, Diéguez-Uribeondo J, et al. The impact of the water moulds Saprolegnia diclina and Saprolegnia parasitica on natural ecosystems and the aquaculture industry. Fungal Biol Rev. 2013;27(2):33–42.
- van West P. Saprolegnia parasitica, an oomycete pathogen with a fishy appetite: new challenges for an old problem. Mycologist. 2006;20(3):99–104.
- Johnson TW, Seymour RL, Padgett DE. Biology and systematics of the Saprolegniaceae. Wilmington (NC): University of North Carolina at Wilmington, Department of Biological Sciences; 2002.
- Seymour RL. The genus Saprolegnia. Nova Hedwigia. 1970;19:1–124.
- Sandoval-Sierra JV, Diéguez-Uribeondo J. A comprehensive protocol for improving the description of Saprolegniales (Oomycota): two practical examples (Saprolegnia aenigmatica sp. nov. and Saprolegnia racemosa sp. nov.). PLOS One. 2015;10(7):e0132999.
- Sandoval-Sierra JV, Latif-Eugenin F, Martín MP, et al. Saprolegnia species affecting the salmonid aquaculture in Chile and their associations with fish developmental stage. Aquaculture. 2014;434:462–469.
- Rezinciuc S, Sandoval-Sierra JV, Diéguez-Uribeondo J. Molecular identification of a bronopol tolerant strain of Saprolegnia australis causing egg and fry mortality in farmed brown trout, Salmo trutta. Fungal Biol. 2014;118(7):591–600.
- Hussein MMA, Hatai K, Nomura T. Saprolegniosis in salmonids and their eggs in Japan. J Wildl Dis. 2001;37(1):204–207.
- Fernández-Benéitez MJ, Ortiz-Santaliestra ME, Lizana M, et al. Saprolegnia diclina: another species responsible for the emergent disease ‘Saprolegnia infections’ in amphibians. FEMS Microbiol Lett. 2008;279(1):23–29.
- Perotti MG, Basanta MD, Steciow MM, et al. Early breeding protects anuran eggs from Saprolegnia infection. Austral Ecol. 2013;38(6):672–679.
- Romansic JM, Diez KA, Higashi EM, et al. Effects of the pathogenic water mold Saprolegnia ferax on survival of amphibian larvae. Dis Aquat Organ. 2009;83(3):187–193.
- Shin S, Kulatunga DCM, Dananjaya SHS, et al. Saprolegnia parasitica isolated from rainbow trout in Korea: characterization, anti-Saprolegnia activity and host pathogen interaction in zebrafish disease model. Mycobiology. 2017;45(4):297–311.
- Jee B-Y, Lee D-C, Kim N-Y, et al. Identification and chemotherapeutic effects of the fungi from three salmonid species and their eggs. J Fish Pathol. 2007;20:147–160.
- Groffen J, Oh SY, Kwon S, et al. High mortality in Bufo gargarizans eggs associated with an undescribed Saprolegnia ferax strain in the Republic of Korea. Dis Aquat Organ. 2019;137(2):89–99.
- Pelizza SA, Cabello MN, Tranchida MC, et al. Screening for a culture medium yielding optimal colony growth, zoospore yield and infectivity of different isolates of Leptolegnia chapmanii (Straminipila: Peronosporomycetes). Ann Microbiol. 2011;61(4):991–997.
- Petrisko JE, Pearl CA, Pilliod DS, et al. Saprolegniaceae identified on amphibian eggs throughout the Pacific Northwest, USA, by internal transcribed spacer sequences and phylogenetic analysis. Mycologia. 2008;100(2):171–180.
- Wolinska J, Giessler S, Koerner H. Molecular identification and hidden diversity of novel Daphnia parasites from European Lakes. Appl Environ Microbiol. 2009;75(22):7051–7059.
- de Bary HA. Species der Saprolegnieen. Bot Zeitung. 1888;46:597–653.
- Coker WC. Leptolegnia from North Carolina. Mycologia. 1909;1(6):262–264.
- Höhnk W, Vallin S. Epidemisches Absterben von Eurytemora im Bottnischen Meerbusen, verursacht durch Leptolegnia baltica nov. spec. Veröffentlichungen des Institutes für Meeresforschung in Bremerhaven. 1953;2:215–223.
- Czeczuga B, Muszynska E. Aquatic fungi growing on the eggs of the percids fish (Percidae) in Poland. Pol J Environ Stud. 1999;8:31–34.
- Montalva C, dos Santos K, Collier K, et al. First report of Leptolegnia chapmanii (Peronosporomycetes: Saprolegniales) affecting mosquitoes in Central Brazil. J Invertebr Pathol. 2016;136:109–116.
- Karling JS. Zoosporic fungi of Oceania. II. Two saprophytic species of Aphanomycopsis. Mycologia. 1968;60(2):271–284.
- Harvey JV. A study of the water molds and Pythiums occurring in the soils of Chapel Hill. J Elisha Mitchell Sci Soc. 1925;41:151–164.
- Steciow MM, Lara E, Pillonel A, et al. Incipient loss of flagella in the genus Geolegnia: the emergence of a new clade within Leptolegnia? IMA Fungus. 2013;4(2):169–175.
- Höhnk W. Nachtrag zu: Die in Nordwestdeutschland gefundenen ufer- und bodenbewohnenden Saprolegniaceae. Veröffentlichungen des Instituts für Meeresforschung in Bremerhaven. 1952;1:126–128.
- Thines M, Choi Y-J. Evolution, diversity, and taxonomy of the Peronosporaceae, with focus on the genus Peronospora. Phytopathology. 2016;106(1):6–18.
- Choi Y-J, Thines M. Host jumps and radiation, not co-divergence drives diversification of obligate pathogens. A case study in downy mildews and Asteraceae. PLOS One. 2015;10(7):e0133655.
- Choi Y-J, Klosterman SJ, Kummer V, et al. Multi-locus tree and species tree approaches toward resolving a complex clade of downy mildews (Straminipila, Oomycota), including pathogens of beet and spinach. Mol Phylogenet Evol. 2015;86:24–34.
- Hulvey J, Telle S, Nigrelli L, et al. Salisapiliaceae – a new family of oomycetes from marsh grass litter of southeastern North America. Persoonia. 2010;25:109–116.
- Nigrelli L, Thines M. Tropical oomycetes in the German Bight – climate warming or overlooked diversity? Fungal Ecol. 2013;6(2):152–160.
- Nam B, Lee D-J, Choi Y-J. High-temperature-tolerant fungus and Oomycetes in Korea, including Saksenaea longicolla sp. nov. Mycobiology. 2021;49(5):476–490.
- Nam B, Choi Y-J. Phytopythium and Pythium species (Oomycota) isolated from freshwater environments of Korea. Mycobiology. 2019;47(3):261–272.
- Masigol H, Khodaparast SA, Mostowfizadeh-Ghalamfarsa R, et al. Taxonomical and functional diversity of Saprolegniales in Anzali Lagoon, Iran. Aquat Ecol. 2020;54(1):323–336.
- White TJ, Bruns T, Lee S, et al. Amplification and direct sequencing of fungal ribosomal RNA genes for phylogenetics. In: Innis MA, Gelfand DH, Sninsky JJ, et al. editors. PCR protocols: a guide to methods and applications. New York: Academic Press, Inc.; 1990. p. 315–322.
- Robideau GP, De Cock AW, Coffey MD, et al. DNA barcoding of oomycetes with cytochrome c oxidase subunit I and internal transcribed spacer. Mol Ecol Resour. 2011;11(6):1002–1011.
- Hudspeth DSS, Steven AN, Hudspeth MES. cox2 molecular phylogeny of the Peronosporomycetes. Mycologia. 2000;92(4):674–684.
- Choi Y-J, Beakes GW, Glockling S, et al. Towards a universal barcode of oomycetes – a comparison of the cox1 and cox2 loci. Mol Ecol Resour. 2015;15(6):1275–1288.
- Katoh K, Toh H. Improved accuracy of multiple ncRNA alignment by incorporating structural information into a MAFFT-based framework. BMC Bioinformatics. 2008;9:1–13.
- Katoh K, Standley DM. MAFFT multiple sequence alignment software version 7: improvements in performance and usability. Mol Biol Evol. 2013;30(4):772–780.
- Kumar S, Stecher G, Li M, et al. MEGA X: molecular evolutionary genetics analysis across computing platforms. Mol Biol Evol. 2018;35(6):1547–1549.
- Vaidya G, Lohman DJ, Meier R. SequenceMatrix: concatenation software for the fast assembly of multi-gene datasets with character set and codon information. Cladistics. 2011;27(2):171–180.
- Hammer Ø, Harper DA, Ryan PD. Past: Paleontological statistics software package for education and data analysis. Palaeontol Electron. 2001;4:1–9.
- Anderson M, Gorley R, Clarke KP. For PRIMER: guide to software and statistical methods. Plymouth, UK: Primer-e; 2008.
- Czeczuga B, Muszyńska E, Krzemińska A. Aquatic fungi growing on the spawn of certain amphibians. Amphib Reptilia. 1998;19(3):239–251.
- Peterson HE. An account of Danish freshwater Phycomycetes, with biological and systematical remarks. Ann Mycol. 1940;8:494–560.
- Bisht GS, Joshi C, Khulbe RD. Watermolds: potential biological control agents of malaria vector Anopheles culicifacies. Curr Sci. 1996;70:393–395.
- Scholte EJ, Knols BGJ, Samson RA, et al. Entomopathogenic fungi for mosquito control: a review. J Insect Sci. 2004;4:19.
- de Bary HA. Einige neue Saprolegnieen. Jahrb Wiss Bot. 1860;2:169–192.
- Cook WRI, Morgan E. Some observations on the Saprolegniaceae of the soils of Wales. J Bot Lond. 1934;72:345–349.
- Dick MW. Saprolegnia asterophora de Bary (1860). Trans Brit. 1960;43:597–602.
- Czeczuga B, Muszyńska E, Tryggvadottir SV. Aquatic fungi growing on the eggs on nine salmonid species on the genus Hucho, Salmo, and Salvelinus. Acta Ichthyol Piscat. 1996;26(2):113–124.
- Elliott RF. Morphological variation in New Zealand Saprolegniaceae. N Z J Bot. 1968;6(1):94–105.
- Edgerton BF, Evans LH, Stephens FJ, et al. Synopsis of freshwater crayfish diseases and commensal organisms. Aquaculture. 2002;206(1–2):57–135.
- Krugner-Higby L, Haak D, Johnson PTJ, et al. Ulcerative disease outbreak in crayfish Orconectes propinquus linked to Saprolegnia australis in Big Muskellunge Lake, Wisconsin. Dis Aquat Org. 2010;91(1):57–66.
- Cao H, Ou R, Li G, et al. Saprolegnia australis R. F. Elliott 1968 infection in Prussian carp Carassius gibelio (Bloch, 1782) eggs and its control with herb extracts. J Appl Ichthyol. 2014;30(1):145–150.
- Hatai K, Egusa S, Nomura T. Saprolegnia australis Elliot isolated from body surface lesions of rainbow trout fingerlings. Fish Pathol. 1977;11(4):201–206.
- Fregeneda-Grandes JM, Rodríguez-Cadenas F, Aller-Gancedo JM. Fungi isolated from cultured eggs, alevins and broodfish of brown trout in a hatchery affected by saprolegniosis. J Fish Biol. 2007;71(2):510–518.
- Coker WC. The Saprolegniaceae, with notes on other water molds. Chapel Hill (NC): University of North Carolina Press; 1923.
- Dzyuba EV, Kondratov IG, Maikova OO, et al. Water molds of the order Saprolegniales (Oomycota) in association with fish and sponge species from Lake Baikal. Biol Bull Russ Acad Sci. 2020;47(5):514–521.
- Humphrey JE. The Saprolegniaceae of the United States, with notes on other species. Trans Am Philos Soc. 1893;17(3):63–148.
- Kützing FT. Phycologia generalis oder, Anatomie, physiologie und systemkunde der tange. Vol. 35. Leipzig, Germany: F. A. Brockhaus; 1843.
- Cao H, Zheng W, Xu J, et al. Identification of an isolate of Saprolegnia ferax as the causal agent of saprolegniosis of yellow catfish (Pelteobagrus fulvidraco) eggs. Vet Res Commun. 2012;36(4):239–244.
- Thuret G. Recherches zur les Zoospores des Alguez et les Antheridies des Cryptogams. Ann Sci Nat Bot. 1850;3:214.
- Liberman K. Pathobiology of water molds in fish: an insight into Saprolegniasis. Orono (ME): University of Maine; 2017.
- de Bary HA. Zu Pringsheims Beobachtungen über den Befruchtungsact der Gattungen Achlya und Saprolegnia. Bot Zeitung. 1883;41:38–60.
- Sarowar MN, Cusack R, Duston J. Saprolegnia molecular phylogeny among farmed teleosts in Nova Scotia, Canada. J Fish Dis. 2019;42(12):1745–1760.
- Ault KK, Johnson JE, Pinkart HC, et al. Genetic comparison of water molds from embryos of amphibians Rana cascadae, Bufo boreas and Pseudacris regilla. Dis Aquat Organ. 2012;99(2):127–137.
- Krauss GJ, Solé M, Krauss G, et al. Fungi in freshwaters: ecology, physiology and biochemical potential. FEMS Microbiol Rev. 2011;35(4):620–651.
- Gessner M, Gulis V, Kuehn K, et al. 17 Fungal decomposers of plant litter in aquatic ecosystems. Environ Microbiol. 2007;4:301–324.
- Czeczuga B, Kiziewicz B, Mazalska B. Further studies on aquatic fungi in the river Biebrza within Biebrza National Park. Pol J Environ Stud. 2003;12:531–543.
- Paliwal PC, Sati SC. Distribution of aquatic fungi in relation to physicochemical factors of Kosi River in Kumaun Himalaya. Nat Sci. 2009;7:70–74.
- Bly J, Lawson L, Dale D, et al. Winter saprolegniosis in channel catfish. Dis Aquat Org. 1992;13:155–164.
- Liu S, Song P, Ou R, et al. Sequence analysis and typing of Saprolegnia strains isolated from freshwater fish from Southern Chinese regions. Aquac Fish. 2017;2(5):227–233.
- Tandel RS, Dash P, Bhat RAH, et al. Morphological and molecular characterization of Saprolegnia spp. from Himalayan snow trout, Schizothorax richardsonii: a case study report. Aquaculture. 2021;531:735824.
- Couch JN. The development of the sexual organs in Leptolegnia caudata. Am J Bot. 1932;19(7):584–599.
- Czeczuga B, Kozłowska M, Godlewska A. Zoosporic aquatic fungi growing on dead specimens of 29 freshwater crustacean species. Limnologica. 2002;32(2):180–193.
- Eissa AE, Abdelsalam M, Tharwat N, et al. Detection of Saprolegnia parasitica in eggs of angelfish Pterophyllum scalare (Cuvier–Valenciennes) with a history of decreased hatchability. Int J Vet Sci. 2013;1(1):7–14.
- Kiesecker JM, Blaustein AR, Belden LK. Complex causes of amphibian population declines. Nature. 2001;410(6829):681–684.
- Carey C, Alexander MA. Climate change and amphibian declines: is there a link? Divers Distrib. 2003;9(2):111–121.
- Pounds JA. Climate and amphibian declines. Nature. 2001;410(6829):639–640.