Abstract
Two fungal strains belonging to Ascomycota were discovered in Gyeonggi-do, Korea, during this investigation of soil microfungi and microbiota of insects. The strain KNUF-20-047 produced milky white on the back and a milky creamy center to white toward the margin on the front side of colonies. Conversely, the closest Xenoacremonium falcatus displayed a pale luteous to luteous center, white toward margins on the front side, and pale luteous or luteous pigment on the back side, whereas X. recifei produced white colonies. The conidiophores of KNUF-20-047 were slightly larger than those of X. falcatus, and the conidia were distinct from X. recifei. Strain KNUF-20-NI-005 produced light brown to subhyaline conidiophores up to 56.0 μm tall, whereas Rhinocladiella anceps displayed golden to dark brown conidiophores up to 350 μm. Strain KNUF-20-NI-005 also produced larger conidia than R. anceps but smaller than R. coryli and R. fasciculata. Moreover, the molecular phylogeny strongly supports the detailed description and illustration of each proposed species to be designated as Xenoacremonium minutisporum sp. nov. and Rhinocladiella terrigenum sp. nov. in Korea.
1. Introduction
Among the estimated 2–11 million fungal species, only approximately 150,600 have been adequately characterized [Citation1–3]. The highest estimates of fungal species came from metabarcoding data, with estimates of 11.7–13.2 million species [Citation4]. Fungi are among the most significant microorganisms in the world due to their impact on humans and their activities, as well as the critical roles they play in ecosystem functioning [Citation5]. Despite significant efforts to enhance agricultural productivity, fungi have significantly reduced the postharvest yield of vegetables and fruits globally [Citation6]. The Nectriaceae family is distinguished by the presence of yellow, orange-red, purple, or white ascomata. The MycoBank database (https://www.mycobank.org/) currently lists four members of the genus Xenoacremonium under Nectriaceae. Some members of this genus are facultatively fungicolous or insectivorous, whereas others are soil-borne saprophytes or weak to virulent, facultative, or obligate plant pathogens [Citation7–10]. Additionally, certain species have been identified as critical opportunistic pathogens causing human infections [Citation11], whereas others create mycotoxins of significant scientific value [Citation12].
Chaetothyriales is a fungal order divided into Chaetothyriaceae and Herpotrichiellaceae families, which comprise non-lichenized ascomycetes [Citation13]. The well-defined ascomycete family, Herpotrichiellaceae, includes 16 genera and over 270 species [Citation14,Citation15]. A black yeast-like fungus belonging to the mitosporic Herpotrichiellaceae family was named the Rhinocladiella genus by Swedish botanist John Axel Nannfeldt in 1934. Furthermore, Rhinocladiella atrovirens has been designated as the type species for this fungus [Citation16,Citation17]. The Rhinocladiella genus includes members that cause illnesses in humans and animals, as well as plant pathogens. This widely distributed fungal genus is present in rotting wood, soil, herbaceous substrates, and other environments. Several Rhinocladiella spp. have been reported in Korea. In 2017, a news report stated that Rhinocladiella similis had been newly isolated from agricultural field soils [Citation18].
We aimed to identify and categorize new fungal species native to Korea. Molecular phylogenetic analyses were performed to identify novel species and describe their cultural and morphological characteristics. The isolated fungi were the focus of the current study, in which they were characterized and presented as novel fungal species.
2. Materials and methods
2.1. Sample collection and isolation of fungal strains
In 2018, the fungal isolates used in this investigation were identified in soil samples and beetles collected from Gyeonggi-do (37°28′03.7′′N 127°32′30.3′′E), Korea. The samples were taken from the field at a depth of 10–15 cm, air-dried, and stored at 4 °C in a plastic bag. The fungi were isolated using the traditional dilution plating method [Citation19]. The beetle sample was washed once with 70% ethanol, twice with sterile distilled water, and then ground using a hand grinder. Subsequently, the samples were suspended in 1.0 mL of sterile distilled water, vortexed gently, and diluted serially, followed by the spreading of 100 µL of each sample onto PDA (Difco, Detroit, MI, USA) plates and incubation at 25 °C for 2–3 days [Citation20]. Single colonies were transferred to potato dextrose agar plates and incubated for 4–5 days at 25 °C. The KNUF-20-047 and KNUF-20-NI-005 strains were selected for additional molecular analyses based on various culture parameters. For additional research, the fungal strains were kept at −80 °C in 20% glycerol. The National Institute of Biological Resources (NIBR) received fungal strains KNUF-20-047 (NIBRFGC000507844) and KNUF-20-NI-005 (NIBRFGC000507840).
2.2. Cultural and morphological characterization
The culture characteristics and morphological observations of the KNUF-20-047 and KNUF-20-NI-005 fungal strains were recorded using different media. Each fungal strain was cultured on potato dextrose agar (PDA), 2% malt extract agar (MEA; Difco), and oatmeal agar (OA; Difco) and incubated for 7–21 days at 25 °C [Citation17,Citation21]. Fungal growth and colony characteristics such as color, shape, and size were monitored. Morphological characteristics were examined using a BX-50 light microscope (Olympus, Tokyo, Japan).
2.3. Genomic DNA extraction, PCR amplification, and sequencing
Total genomic DNA from the KNUF-20-047 and KNUF-20-NI-005 strains were extracted from the fungal mycelia grown on the PDA plate using a HiGene Genomic DNA Prep Kit (BIOFACT, Daejeon, Korea) following the manufacturer’s protocol. Polymerase chain reactions (PCR) were performed using the internal transcribed spacer (ITS) regions, 28 s rDNA large subunit (LSU), partial RNA polymerase II subunit (RPB2), and translation elongation factor 1-alpha (TEF1α) genes as targets for molecular identification [Citation22]. The ITS1/ITS4 primer pair was used to amplify the ITS regions of the nuclear rRNA gene [Citation23], fRPB2-5F/fRPB2-7cR primers for the partial RPB2 [Citation24], LROR [Citation25], and LR5 [Citation26] primers for partial gene sequences of the 28S rDNA LSU; and EF1–526F/EF1–986R primers for TEF1α [Citation27]. The RPB2 region was not successfully amplified, resulting in the exclusion of this marker in further analyses of the KNUF-20-047 strain. The quality of the amplified PCR products was checked by 1.2% agarose gel electrophoresis stained with ethidium bromide. The electrophoresed product was purified using ExoSAP-IT (Thermo Fisher Scientific, Waltham, MA, USA) and then sent to SolGent for sequencing (Daejeon, Korea).
2.4. Molecular phylogenetic analyses
Phylogenetic analyses were performed using the sequences retrieved from the National Center for Biotechnology Information (NCBI) (). Ambiguous regions were deleted from the alignments, and evolutionary distance matrices for the neighbor-joining (NJ) algorithm were calculated using Kimura’s two-parameter model [Citation28]. The NJ method [Citation29] was used to infer tree topology using MEGA7.0 software, with bootstrap values based on 1000 replicates [Citation30].
Table 1. GenBank accession numbers used for the phylogenetic analyses in this study.
3. Results
3.1. Taxonomical analysis of Xenoacremonium minutisporum sp. Nov
3.1.1. Taxonomy
The KNUF-20-047 strain exhibited morphological characteristics distinct from those of allied species of Xenoacremonium and was therefore described as a new species.
Xenoacremonium minutisporum S.Y. Lee and H.Y. Jung, sp. nov. ()
Figure 1. Cultural and morphological characteristics of KNUF-20-047T. The colony growth on potato dextrose agar (A), oatmeal agar (B), and malt extract agar (C) after 14 days of incubation at 25 °C. Lateral phialidic pegs (D, E); conidiophores arising laterally from somatic hyphae (F, G); conidia (H, I). Scale bars: D–I = 10 μm.
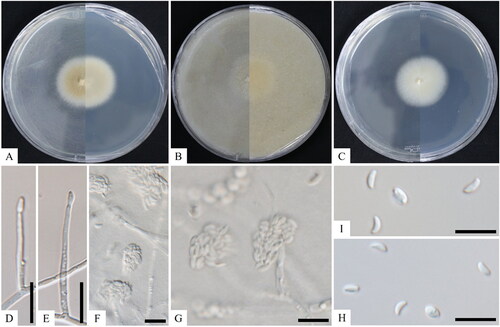
MycoBank: MB8457434
Etymology: The specific epithet “minutisporum” refers to the small size of conidia.
Typus: Gyeonggi-do (37°28′03.7′′N 127°32′30.3′′E), isolated from the female beetle, Dorcus titanus castanicolor. The stock culture (NIBRFGC000507844) was deposited in the National Institute of Biological Resources (NIBR) as a metabolically inactive culture.
Ecology and distribution: Members of this genus are considered pathogens of plants and humans and are widely dispersed in tropical and temperate ecosystems worldwide. Some species have been isolated from various plants, such as macadamia, Persea sp. (avocado), and pomegranate. Some countries, such as China, New Zealand, Brazil, and the USA, have reported Xenoacremonium spp. mainly from the soil, leaves, and stem/bark of these plants. The proposed novel species, X. minutisporum, was isolated from a female beetle, D. titanus castanicolor, in Korea.
Cultural characteristics: The KNUF-20-047 strain was cultured on MEA, OA, and PDA at 25 °C for 7–14 days to observe its morphological structure with a normal growth rate. Colonies grew moderately, attaining 55–60 mm in diameter on MEA and PDA and 45–55 mm on OA at 25 °C for 10 days of incubation (). Colonies consisted of semi-immersed aerial mycelium. The surface had a pale luteous to luteous center becoming white toward the margins, and the reverse side of the plate was pale luteous with a pale luteous or luteous pigment throughout the medium. Colonies on OA became buff, planar, and portioned with regular margins (). These colonies were initially milky white on the front side of the plate, and over time, they became light yellow-white to dull yellow-white from the center outward. Colonies on the back side of the plate were creamy pale-yellow white on PDA and clear white on MEA and OA from the center outward. The milky white droplets were smooth ().
Morphological characteristics: The hyphae of strain KNUF-20-047 were a light white color, septate, branched, and were commonly 1.0–4.0 µm wide. Conidiophores that arose laterally from somatic hyphae were either lateral phialidic pegs, 2.0–4.0 × 1.0–2.0 µm, or erect, subcylindrical, unbranched or rarely branched, 0- to 1-septate, up to 80 µm tall, 2.0–4.0 µm in diameter, hyaline, smooth, and terminating in one or two conidiogenous cells (). Conidia were straight or slightly curved, hyaline, smooth, formed in heads at the apex of conidiogenous cells, subcylindrical, tapering toward the apex with periclinal thinning and inconspicuous collarette, slightly to strongly curved, 2.4–9.6 × 1.0–3.0 µm in size (). Chlamydospores were not observed.
Note: The KNUF-20-047 strain produced milky white colonies on the back side of the media surfaces and a milky, creamy center turning yellow to white toward the margin on the front side of the colonies. Conversely, Xenoacremonium falcatus displayed a pale luteous to luteous center, turning white toward the margins on the front side of the plate, with the back side displaying a pale luteous or luteous pigment throughout the medium. Furthermore, Xenoacremonium recifei produced white colonies (). The conidiophores (2.0 × 7.0 µm) of the KNUF-20-047 strain were slightly larger than those of X. falcatus (2.0–4.0 × 1.0–2.0 µm). The conidia of strain KNUF-20-047 (2.4–9.6 × 1.0–3.0 µm) were shorter in length but almost similar in width to those of X. falcatus (4.0–8.0(–10.0) × 1.0–2.0 µm) but were also longer than those of X. recifei (4.0–6.0(–7.5) × 1.0–2.0 µm) (). Thus, the proposed strain KNUF-20-047 differed from X. falcatus and X. recifei in their sizes of conidiophores, conidia, and cultural characteristics, especially the presence of a milky smooth surface.
Table 2. Morphological comparison between Xenoacremonium minutisporum (KNUF-20-047T) and the closest species of Xenoacremonium.
3.1.2. Molecular phylogeny of strain KNUF-20-047
The ITS regions (535 bp) demonstrated 99.43, 99.23, and 98.84% sequence similarities to the reported species, X. falcatus isolate 1609, X. falcatus CBS 400.85T, and X. recifei CBS 541.89, respectively. The closest taxonomic relatives of the isolated strain KNUF-20-047 were identified using the LSU (729 bp) gene sequence, which revealed a high sequence similarity of 99.72–99.86% to the isolates, X. falcatus CBS400.85T and X. recifei CBS 596.74. TEF1-α (416 bp) displayed 95.00 and 93.87% sequence similarities with X. falcatus CBS400.85T and X. recifei CBS 541.89, respectively. Phylogenetic analyses using TEF1-α gene sequences were performed for accurate differentiation between isolates. The strain KNUF-20-047 was closely clustered with X. falcatus CBS 400.85T in the NJ phylogenetic tree using the TEF1-α gene sequences indicated by the node in the neighbor-joining phylogenetic tree along with the filled nodes in the maximum likelihood and maximum parsimony trees. Open circles show nodes created using maximum likelihood or maximum parsimony (). The precise taxonomic position of the strain was ascertained by a phylogenetic analysis based on a combination of sequences with maximum parsimony (tree length = 168, consistency index = 0.90, retention index = 0.92, and composite index = 0.87). Therefore, KNUF-20-047 represented a single, novel, and phylogenetically distinct species. Based on the phylogenetic analysis and morphological observations, strain KNUF-20-047 was distinct from the previously identified Xenoacremonium species (). Thus, it should be placed in the genus as a novel species, for which we proposed the name X. minutisporum sp. nov.
Figure 2. Neighbor-joining phylogenetic tree of KNUF-20-047T based on the TEF1α sequences indicating the relationship between Xenoacremonium minutisporum and the closest species. The tree was rooted using Stachybotrys chartarum CBS 129.13 as the outgroup. The neighbor-joining phylogenetic tree, maximum likelihood, and maximum parsimony trees indicated with filled nodes, whereas open circles showed maximum-likelihood or parsimony. The strain isolated in this study is in bold, and the bootstrap values (>50%) are based on 1000 replicates. Bar = 0.1 substitutions per nucleotide position.
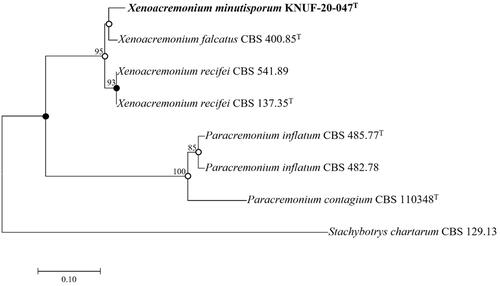
3.2. Taxonomical analysis of Rhinocladiella terrigenum sp. nov
3.2.1. Taxonomy
Strain KNUF-20-NI-005 exhibited morphological characteristics different from those of other Rhinocladiella spp. Therefore, it was proposed as a new species.
Rhinocladiella terrigenum H.Y. Jung and S.Y. Lee, sp. nov. ()
Figure 3. Cultural and morphological characteristics of KNUF-20-NI-005T. The colony growth on potato dextrose agar (A), oatmeal agar (B), and malt extract agar (C) for 14 days of incubation at 25 °C. Conidial structure at different stages of development, resulting in semi-micronematous conidiophores and sympodially proliferating conidiogenous cells (D–F); conidiogenous cells (G, H); conidia (I). Scale bars: D–I = 10 μm. Arrows indicate conidial structure.
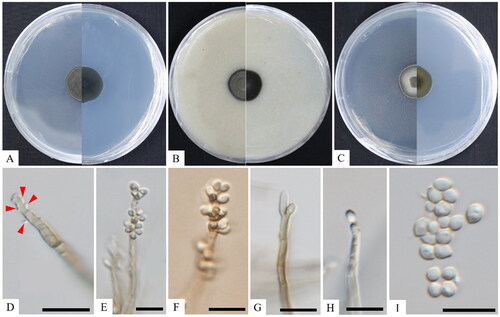
MycoBank: MB845744
Etymology: This refers to the soil, the substrate from which the type strain was isolated.
Typus: Gyeonggi-do (37°28′03.7′′N 127°32′30.3′′E), isolated from the rhizosphere soil of Robinia pseudoacacia. The stock culture (NIBRFGC000507840) was deposited in the NIBR as a metabolically inactive culture.
Ecology and distribution: Members of this genus are primarily considered human pathogens (for example, they cause chromoblastomycosis) and are present globally, including in soil, herbaceous substrates, and rotting wood. Chromoblastomycosis is common in tropical, subtropical, and temperate climates, with high prevalence rates in Madagascar, South Africa, Brazil, Costa Rica, Australia, and other Asian countries such as India, China, Japan, South Korea, and Malaysia. The proposed novel species, R. terrigenum, was collected from soil in Korea.
Cultural characteristics: After 2 weeks of dark incubation at 25 °C, the colonies of strain KNUF-20-NI-005 developed slowly, with a moist texture, irregular form, and curled margin, with diameters ranging from 19.4–20.7 mm on PDA, 18.8–19.4 mm on MEA, and 18.4–18.9 mm on OA (). However, this species did not grow at 35 °C. Colonies on PDA were dark olivaceous green on both the front and reverse sides of the plate, with smooth, aerial mycelium with a dark-grey-green circle (). The colonies on OA created a dark color on both the front and back sides of the plate, as well as dark-dust grey, smooth aerial mycelium (). Additionally, colonies on MEA were dark olivaceous on the front side of the plate and orange-green on the reverse side, with pale yellow aerial hyphae in the inner circle and dark-grey green aerial hyphae in the outer circle ().
Morphological characteristics: Strain KNUF-20-NI-005 comprised branched hyphae, which were brown to pale brown, 2.0–3.9 µm wide, and irregularly septate from 10.1–26.5 µm, and conidiophores consisting of conidiogenous cells that were connected with vegetative hyphae. Conidiophores were erect, straight, thick-walled, up to 56.0 µm tall, brown to pale brown, bearing small, pigmented denticles, on which conidia are generated in a sympodial order and are 1.6–3.8 µm wide (). Furthermore, conidiophores bore multiple conidia, terminal or lateral conidiogenous cells, often intercalary, cylindrical in an apical section with multiple conidia-connected cells, and rachis with scattered and pimple-shaped denticles (). Single conidia developed budding cells and were unicellular, hyaline, generally 1-septate, and 2.3–4.2 × 3.1–5.7 µm ().
Note: The KNUF-20-NI-005 strain produced light brown to subhyaline conidiophores, up to 56.0 µm tall, intercalary cells 4.0–27.0 µm long, whereas the strain, Rhinocladiella anceps, displayed golden to dark brown conidiophores, up to 350 µm tall, and intercalary cells 9.0–14.0 µm long. Lastly, the conidiophores of R. coryli were straight to slightly curved, grey-brown, smooth, subcylindrical with a slight apical taper, unbranched, 0–7-septate, and 10–70 × 2.5–3.0 µm (). KNUF-20-NI-005 produced larger conidia (2.3–4.2 × 3.1–5.7 µm) than those of R. anceps (2.5–4.0 × 2.0–2.5 µm) but different from those of R. coryli ((3)4–4.5(5) × 2(2.5) µm) and R. fasciculata ((2.5–)4.0–5.0(–6.0) × 2.0–3.0 µm) (). The proposed strain produced shorter conidiophores than those of R. anceps and different-sized conidia than those of the closest species. Thus, the morphology of strain KNUF-20-NI-005 was distinct from the previously identified species of Rhinocladiella.
Table 3. Morphological comparison between Rhinocladiella terrigenum (KNUF-20-NI-005T) and the closest species of Rhinocladiella.
3.2.2. Molecular phylogeny of strain KNUF-20-NI-005
Based on the sequencing analyses, the lengths of the sequences were 639 bp and 837 bp for the ITS regions and 28S rDNA of LSU, respectively. The NCBI BLAST tool was used to investigate the novel species in this genus, and gene markers for the ITS regions and 28S rDNA were used to analyze the sequences. Strain KNUF-20-NI-005 had 86.7% and 86.6% sequence similarity to R. anceps (CBS 538.69; CBS 157.54) for the ITS regions. For the 28S rDNA, the isolated strain demonstrated sequence similarities of 98.2% to R. atrovirens (CBS 317.33; CBS 264.49) and 97.8% to R. aquaspera (CBS 127261; CBS 127260; CBS 313.73), R. anceps (MUCL 7792), and R. aquaspera (CBS 122635). The phylogenetic analysis was built using a combination of ITS regions and partial 28S rDNA sequences, as indicated by the node in the neighbor-joining phylogenetic tree, as well as the filled nodes in the maximum likelihood and maximum parsimony trees. The maximum likelihood or maximum parsimony was also used to make the corresponding nodes, as shown by the open circles (). A phylogenetic analysis was also conducted based on a combination of sequences with maximum parsimony (tree length = 345, consistency index = 0.64, retention index = 0.61, and composite index = 0.45) to determine the exact taxonomic position of the strain. The phylogenetic tree revealed that KNUF-20-NI-005 was distinctly clustered in a different clade with respect to the previously identified strains of Rhinocladiella (). Thus, strain KNUF-20-NI-005 was phylogenetically distinct from other Rhinocladiella spp.
Figure 4. Neighbor-joining phylogenetic tree of KNUF-20-NI-005T based on ITS and LSU sequences, depicting the phylogenetic position among the related strains in the genus Rhinocladiella. Cladophialophora carrionii CBS 160.54T was selected as the outgroup. The neighbor-joining tree, maximum likelihood, and maximum parsimony trees indicated with filled nodes and open circles were made using maximum likelihood or parsimony. The strain isolated in this study is in bold, and the numbers above the branches represent the bootstrap values obtained for 1000 replicates. Bar = 0.01 substitutions per nucleotide position.
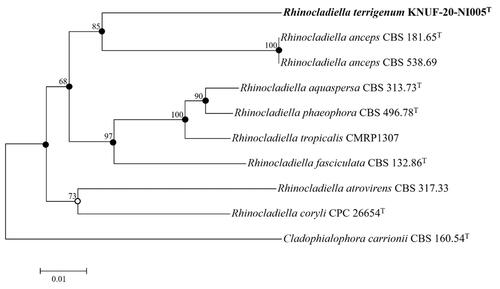
4. Discussion
Morphologically distinct strains, KNUF-20-047 and KNUF-20-NI-005, were isolated from soil and female beetles, D. titanus castanicolor, obtained from Gyeonggi-do, Korea. The strains were morphologically different from each of the identified closely related species ( and ).
According to Ko and Kunimoto [Citation33], Xenoacremonium spp. are widely distributed from temperate to tropical regions worldwide and cause infected trees to perish within 2–3 months of the onset of early foliar symptoms, such as fading color and yellowing. Although the exact hosts of the genus Xenoacremonium have not yet been determined, this species has been detected in several plants, including macadamia, Persea sp. (avocado), and pomegranate [Citation34]. Several nations, including China, New Zealand, Brazil, and the USA, have documented the presence of Xenoacremonium spp. in soil, plant leaves, stems, and bark. The first sexual species in this genus, X. brunneosporum, was identified in a marine habitat on Rhizophora mucronata in Thailand and is the most well-known species in the genus, along with X. recifei and X. falcatus (plant and human pathogens) [Citation35]. However, the genus Xenoacremonium was established with a group of fungi previously treated as Acremonium recifei [Citation36], including the ex-type of A. recifei (CBS 137.35) [Citation22]. Members of the genus Acremonium, such as A. zeylanicum, have been isolated from infected citrus cottony scale (Pulvinaria aurantia), which belongs to the family Coccidae in the order Hemiptera [Citation37]. The pathogenic effect of A. zeylanicum has previously been reported on the sugarcane wooly aphid (Ceratovacuna lanigera) in India [Citation38] and the two-spotted spider mite (Tetranychus urticae Koch) [Citation39]. Currently, four species of Xenoacremonium have been identified so far, including a sexual morph from different countries with diverse hosts and none of the species was not recorded in a freshwater environment. However, no sexual or asexual morph has been recorded under this genus in Korea until now. Although members of the genus Xenoacremonium occur in many habitats worldwide, the identified strain, KNUF-20-047, was isolated from the female beetle, D. titanus castanicolor, in Korea.
The Rhinocladiella genus comprises a black yeast-like fungus present in various environments, including soil, herbaceous substrates, and decaying wood [Citation17,Citation40]. The close relatives of black filamentous fungi R. similis were isolated from both groundwater and surface water [Citation41]. Chromoblastomycosis is a disease that can affect humans and is caused by the genera of melanized fungi. Chromoblastomycosis and/or phaeohyphomycosis are caused by the genera Exophialia, Cladophialophora, Fonsecaea, and Phialophora [Citation42,Citation43]. Chromoblastomycosis is most prevalent in tropical, subtropical, and temperate regions, with significant incidence rates in Madagascar, South Africa, Brazil, Costa Rica, and other Asian countries. Additionally, this infection has been observed on the limbs of agricultural farmers and laborers because the causative agents are primarily located in the soil and decaying vegetation [Citation44–46]. The Rhinocladiella genus contains several clinically relevant species such as R. mackenziei, R. basitonum, R. fasciculatum, R. anceps, R. atrovirens, R. phaeophora, R. aquaspersa, and R. similis. Some of these species are also responsible for human infection. R. mackenziei is one of the most well-known causes of cerebral phaeohyphomycosis, with cases frequently appearing in healthy individuals. R. aquaspersa is also frequently linked to chromoblastomycosis lesions, and R. basitona and R. similis are two of the most common skin pathogens [Citation11,Citation17,Citation47,Citation48].
In conclusion, each species analyzed in this study had a variety of hosts, including both plants and humans, and caused various diseases. Based on cultural, morphological, and phylogenetic analyses, the strains were identified as X. minutisporum and R. terrigenum. These analyses suggested that the strains notably differed from the previously described strains of the genera. Further research is required to explore the etiology of these species as well as their pathogenicity, along with their ecological importance based on Korean soils, environmental conditions, and relationships in agricultural production, thereby considering all the aspects of these two new species.
Disclosure statement
No potential conflict of interest was reported by the author(s).
Additional information
Funding
References
- Hawksworth DL, Lücking R. Fungal diversity revisited: 2.2 to 3.8 million species. Microbiol Spectr. 2017;5(4):79–95.
- Lücking R, Aime MC, Robbertse B, et al. Fungal taxonomy and sequence-based nomenclature. Nat Microbiol. 2021;6(5):540–548.
- Baldrian P, Větrovský T, Lepinay C, et al. High-throughput sequencing view on the magnitude of global fungal diversity. Fungal Divers. 2021;19:1–9.
- Wu B, Hussain M, Zhang W, et al. Current insights into fungal species diversity and perspective on naming the environmental DNA sequences of fungi. Mycology. 2019;10(3):127–140.
- Mueller GM, Foster M, Bills GF. Biodiversity of fungi inventory and monitoring methods. San Diego (CA): Elsevier Academic Press; 2004.
- Pérez-García A, Romero D, de Vicente A. Plant protection and growth stimulation by microorganisms: biotechnological applications of bacilli in agriculture. Curr Opin Biotechnol. 2011;22(2):187–193.
- Rossman AY, Samuels GJ, Rogerson CT, et al. Genera of Bionectriaceae, Hypocreaceae and Nectriaceae (Hypocreales, Ascomycetes). Stud Mycol. 1999;42:1–248.
- Rossman AY. Towards monophyletic genera in the holomorphic hypocreales. Stud Mycol. 2000;45:27–34.
- Chaverri P, Salgado C, Hirooka Y, et al. Delimitation of Neonectria and Cylindrocarpon (Nectriaceae, Hypocreales, Ascomycota) and related genera with Cylindrocarpon-like anamorphs. Stud Mycol. 2011;68:57–78.
- Gräfenhan T, Schroers HJ, Nirenberg HI, et al. An overview of the taxonomy, phylogeny, and typification of nectriaceous fungi in Cosmospora, Acremonium, Fusarium, Stilbella, and Volutella. Stud Mycol. 2011;68:79–113.
- Chang DC, Grant GB, O’Donnell K, et al. Multistate outbreak of Fusarium keratitis associated with use of a contact lens solution. JAMA. 2006;296(8):953–963.
- Rossman AY. Morphological and molecular perspectives on systematics of the Hypocreales. Mycologia. 1996;88(1):1–19.
- Geiser DM, Gueidan C, Miadlikowska J, et al. Eurotiomycetes: Eurotiomycetidae and Chaetothyriomycetidae. Mycologia. 2006;98(6):1053–1064.
- Wijayawardene NN, Hyde KD, Al-Ani LKT, et al. Outline of fungi and fungus-like taxa. Mycosphere. 2020;11(1):1060–1456.
- Munk A. The system of the Pyrenomycetes. Dansk Botanisk Arkiv. 1953;15:1–163.
- Liu D. Molecular detection of human fungal pathogens. London (UK): Taylor & Francis Group CRC Press; 2011.
- Arzanlou M, Groenewald JZ, Gams W, et al. Phylogenetic and morphotaxonomic revision of Ramichloridium and allied genera. Stud Mycol. 2007;58:57–93.
- Adhikari M, Kim HS, Gurung SK, et al. Two new records of Ascomycetes from crop field soils in Korea. Kor J Mycol. 2017;45:167–174.
- Park S, Ten L, Lee SY, et al. New recorded species in three genera of the Sordariomycetes in Korea. Mycobiology. 2017;45(2):64–72.
- Das K, Kim JH, Choi KS, et al. A new report of Biscogniauxia petrensis isolated from mosquitoes in Korea. Kor J Mycol. 2020;48(2):87–93.
- Crous PW, Verkley GJM, Groenewald JZ, et al. Fungal biodiversity. CBS laboratory manual series no. 1. Utrecht (The Netherlands): CBS-KNAW Fungal Biodiversity Centre; 2009.
- Lombard L, van der Merwe NA, Groenewald JZ, et al. Generic concepts in Nectriaceae. Stud Mycol. 2015;80:189–245.
- White TJ, Bruns T, Lee S, et al. Amplification and direct sequencing of fungal ribosomal RNA genes for phylogenetics. In: Innis MA, Gelfand DH, Sninsky JJ, White TJ, editors. PCR protocols: a guide to methods and applications. New York (NY): Academic Press, Inc.; 1990. p. 315–322.
- Liu YJ, Whelen S, Hall BD. Phylogenetic relationships among Ascomycetes: evidence from an RNA polymerse II subunit. Mol Biol Evol. 1999;16(12):1799–1808.
- Rehner SA, Samuels GJ. Taxonomy and phylogeny of Gliocladium analysed from nuclear large subunit ribosomal DNA sequences. Mycol Res. 1994;98(6):625–634.
- Vilgalys R, Hester M. Rapid genetic identification, and mapping of enzymatically amplified ribosomal DNA from several Cryptococcus species. J Bacteriol. 1990;172(8):4238–4246.
- Carbone I, Kohn LM. A method for designing primer sets for speciation studies in filamentous Ascomycetes. Mycologia. 1999;91(3):553–556.
- Kimura M. A simple method for estimating evolutionary rates of base substitutions through comparative studies of nucleotide sequences. J Mol Evol. 1980;16(2):111–120.
- Saitou N, Nei M. The neighbor-joining method: a new method for reconstructing phylogenetic trees. Mol Biol Evol. 1987;4(4):406–425.
- Kumar S, Stecher G, Tamura K. MEGA7: molecular evolutionary genetics analysis version 7.0 for bigger datasets. Mol Biol Evol. 2016;33(7):1870–1874.
- Hughes SJ. Revisiones hyphomycetum aliquot cum appendice de nominibus rejiciendis. Can J Bot. 1958;36(6):727–836.
- Hernández-Restrepo M, Schumacher RK, Wingfield MJ, et al. Fungal systematics and evolution: FUSE 2. Sydowia. 2016;68:193–230.
- Ko WH, Kunimoto RK. Quick decline of macadamia trees: association with Xylaria arbuscula. Plant Pathol. 1991;40(4):643–644.
- Patel JN, Parmar FA, Upasani VN. Isolation and characterization of pathogens causing disease in pomegranate (Punica granatum L.), India. Int J Innov Res Sci Eng Technol. 2021;10:4403–4408.
- Dayarathne MC, Jones EBG, Maharachchikumbura SSN, et al. Morpho-molecular characterization of microfungi associated with marine based habitats. Mycosphere. 2020;11(1):1–188.
- Gams W. Cephalosporium-artige schimmelpilze (Hyphomycetes). Int Syst Agric Sci Technol. 1971;48:25–26.
- Mousavi K, Rajabpour A, Parizipour MHG, et al. Biological and molecular characterization of Cladosporium sp. and Acremonium zeylanicum as biocontrol agents of Aphis fabae in a tri-trophic system. Entomologia Exp Applicata. 2022;170(10):877–886.
- Patil NB, Mallapur CP, Sujay YH. Efficacy of Acremonium zeylanicum on sugarcane woolly aphid under laboratory conditions. Int J Plant Prot. 2011;4:279–283.
- Krishna RA, Haseena B, Beena S. First report of Acremonium zeylanicum, Conidiobolus sp. and Neozygites floridana as mycopathogens of two spotted spider mite Tetranychus urticae koch on brinjal in polyhouse. Indian J Fundam Appl Life Sci. 2014;4:230–232.
- de Hoog GS, Vicente V, Caligiorne RB, et al. Species diversity and polymorphism in the Exophiala spinifera clade containing opportunistic black yeast-like fungi. J Clin Microbiol. 2003;41(10):4767–4778.
- Babič MN, Zalar P, Ženko B, et al. Yeasts and yeast-like fungi in tap water and groundwater, and their transmission to household appliances. Fungal Ecol. 2016;20:30–39.
- Queiroz-Telles F, de Hoog S, Santos DW, et al. Chromoblastomycosis. Clin Microbiol Rev. 2017;30(1):233–276.
- Gomes RR, Vicente VA, Azevedo CM, et al. Molecular epidemiology of agents of human chromblastomycosis in Brazil with the description of two novel species. PLoS Negl Trop Dis. 2016;10(11):e0005102.
- Queiroz-Telles F, Esterre P, Perez-Blanco M, et al. Chromoblastomycosis: an overview of clinical manifestations, diagnosis, and treatment. Med Mycol. 2009;47(1):3–15.
- Jun JB, Park JY, Kim DW, et al. Chromoblastomycosis caused by Rhinocladiella aquaspersa. Korean J Med Mycol. 2004;9:117–122.
- Attapattu MC. Chromoblastomycosis a clinical and mycological study of 71 cases from Sri Lanka. Mycopathologia. 1997;137(3):145–151.
- González GM, Rojas OC, González JG, et al. Chromoblastomycosis caused by Rhinocladiella aquaspersa. Med Mycol Case Rep. 2013;2:148–151.
- Kanj SS, Amr SS, Roberts GD. Ramichloridium mackenziei brain abscess: report of two cases and review of the literature. Med Mycol. 2001;39(1):97–102.