Abstract
The diversity of A mating type in wild strains of Lentinula edodes was extensively analyzed to characterize and utilize them for developing new cultivars. One hundred twenty-three A mating type alleles, including 67 newly discovered alleles, were identified from 106 wild strains collected for the past four decades in Korea. Based on previous studies and current findings, a total of 130 A mating type alleles have been found, 124 of which were discovered from wild strains, indicating the hyper-variability of A mating type alleles of L. edodes. About half of the A mating type alleles in wild strains were found in more than two strains, whereas the other half of the alleles were found in only one strain. About 90% of A mating type combinations in dikaryotic wild strains showed a single occurrence. Geographically, diverse A mating type alleles were intensively located in the central region of the Korean peninsula, whereas only allele A17 was observed throughout Korea. We also found the conservation of the TCCCAC motif in addition to the previously reported motifs, including ATTGT, ACAAT, and GCGGAG, in the intergenic regions of A mating loci. Sequence comparison among some alleles indicated that accumulated mutation and recombination would contribute to the diversification of A mating type alleles in L. edodes. Our data support the rapid evolution of A mating locus in L. edodes, and would help to understand the characteristics of A mating loci of wild strains in Korea and help to utilize them for developing new cultivars.
1. Introduction
Mating compatibility in heterothallic Basidiomycete fungi is determined by mating type gene(s) located in mating type locus/loci. In the tetrapolar (bifactorial) system, two genetically independent mating type loci, A and B, regulate the viability and development of heterokaryon: only homokaryons carrying different alleles at both loci can successfully complete the sexual development [Citation1–3]. The A mating type locus contains two divergently transcribed homeodomain (HD) transcription factors that govern viability and post-mating growth after cell fusion [Citation1–3]. The B mating type locus harbors tightly linked pheromone and pheromone receptor genes, which are involved in molecular recognition and cell fusion [Citation1–3].
Lentinula edodes, also known as shiitake or pyogo, is an edible mushroom-forming fungus that belongs to the Agaricales order of the Agaricomycetes class in the Basidiomycota phylum. It contributes to about 22% of the total mushroom supply worldwide [Citation4]. It is largely consumed as a food, seasoning, and health-promoting resource because of its unique taste and nutritional value [Citation5–7]. The cultivation of L. edodes began more than 800 years ago [Citation8], and it is primarily cultivated in East Asian countries, such as Korea, China, Japan, and Taiwan using oak tree or sawdust. As present, the diversification of consumers’ preferences and global climate changes have increased the demand for the development of new L. edodes cultivars, as other crops [Citation9,Citation10]. Wild strains have been selected under competitive and variable conditions for a long time; thus, they may serve as genetic resources for introducing novel traits into new cultivars [Citation9,Citation10]. Information on mating types of wild strains of L. edodes is important because it is directly related to mating efficiency.
The B mating type locus of L. edodes consists of two sub-loci, namely Bα and Bβ. Each sub-locus harbors specific pheromone(s) and pheromone receptor genes [Citation11,Citation12]. A previous investigation of 111 dikaryotic strains, including 83 cultivated and 28 wild strains, showed 15 B mating types resulting from recombination between five alleles of Bα sub-locus and three alleles of Bβ sub-locus [Citation12].
The A mating type locus of L. edodes was identified after the completion of genome sequencing [Citation13,Citation14]. The locus contains a pair of HD transcription factor genes, namely HD1 and HD2, in the head-to-head orientation [Citation13]. Remarkably, the region encoding the N-termini of HD1 and HD2 and the intergenic region between HD1 and HD2 were highly variable as reported in other Basidiomycetes [Citation15–17], and these variable regions were proven to be useful as an effective marker to discriminate different A mating type alleles in L. edodes [Citation13,Citation14]. Sequence analysis of this region revealed 130 A mating type alleles in 127 dikaryotic strains, including 96 cultivated and 31 wild strains [Citation14]. Previous research also demonstrated the preferred selection of specific A mating type alleles in strains cultivated in East Asian countries, such as Korea, China, and Japan [Citation14]. However, analysis of the mating type alleles of L. edodes was primarily focused on the cultivated strains at present.
To introduce the wild strains and thereby increase genetic diversity in future breeding programs, we have collected a wide variety of wild L. edodes strains and examined their cultural characteristics [Citation18]. In this study, we extensively analyzed the sequences of the variable region in the A mating type locus of these wild strains to characterize and utilize them for developing new cultivars.
2. Materials and methods
2.1. Strains and culture conditions
All fungal isolates used in this study were obtained from National Institute of Forest Science (NIFoS) and National Academy of Agricultural Science (NAAS). The obtained isolates were grown on potato dextrose agar (PDA; BD Difco, MD, USA) medium for 2 weeks at 25 °C under dark condition.
2.2. Determination of A mating type sequences
Genomic DNAs were isolated from mycelia grown on PDA using the DNeay Plant mini kit (QIAGEN, Hilden, Germany). Amplification of the variable regions of the A mating type loci was conducted as reported previously [Citation14]. The PCR products were purified using the AccuPrep PCR/Gel DNA Purification kit (Bioneer, Daejeon, Korea) and then cloned into a vector using the TOPcloner Blunt kit (Enzynomics, Daejeon, Korea) following the manufacturers’ instructions. The obtained clones were sequenced by Macrogen Inc. (Seoul, Korea) using an ABI 3730xl DNA Analyzer (Applied Biosystems, CA, USA).
2.3. Generation of sequence logo
Conserved motifs previously found in the intergenic region of A mating alleles were parsed out manually in the newly identified A mating alleles. Intergenic regions of each A mating allele containing consensus motifs were aligned using MUSCLE in MEGA 11 software and the sequence logo was generated using WebLogo 3 (https://weblogo.threeplusone.com) [Citation19,Citation20].
2.4. Sequence comparison of A mating type alleles
MUSCLE in MEGA 11 software was used to align different A mating type alleles or clones with default parameters. Identical bases were indicated with black shading using Color Align Conservation program (https://www.bioinformatics.org/sms2/color_align_cons.html) [Citation21].
3. Results
3.1. Hyper-diversity of a mating type in wild strains
We analyzed sequences of the region between homeodomains of HD1 and HD2 genes in the A mating loci of 106 wild strains of L. edodes collected from 1983 to 2020 in Korea (). Using the primer set targeting consensus sequences in the homeodomains of HD1 and HD2 genes, we amplified the variable region, spanning 5′ region of HD1 gene, intergenic region, and 5′ region of HD2 gene, that could represent specific A mating type [Citation13,Citation14]. Because each dikaryotic strain harbor two nuclei carrying different A mating type alleles, it is expected to obtain 212 clones of A mating type alleles from 106 dikaryotic strains. One hundred ninety-five clones of A mating type alleles were obtained, and clones for the remaining 17 alleles could not be acquired probably because of the variation in the homeodomain region targeted by the primers we used.
Table 1. Alleles of A mating type discovered in Korean wild strains of Lentinula edodes.
After sequence analysis of the 195 clones obtained, we newly identified 67 alleles designated as A64 to A130 (). Except for A69 (1511 bp) and A129 (746 bp), the size of the newly found A mating type alleles ranged from 1000 to 1133 bp, with an average and standard deviation of 1083 ± 24 bp, in accordance with the previous observations (Supplementary Figure S1) [Citation14].
Figure 1. Venn diagram showing unique and common A mating type alleles in wild and cultivated strains of Lentinula edodes. The number and percentage of unique and common alleles are indicated in the circles. All alleles identified in this study and a previous study [Citation14] were included in the analysis.
![Figure 1. Venn diagram showing unique and common A mating type alleles in wild and cultivated strains of Lentinula edodes. The number and percentage of unique and common alleles are indicated in the circles. All alleles identified in this study and a previous study [Citation14] were included in the analysis.](/cms/asset/71fed3e9-19f5-49dd-aae1-83e219ff25de/tmyb_a_2161157_f0001_c.jpg)
A previous study on A mating type alleles of cultivated and wild strains of L. edodes collected from East Asia revealed that several A mating type alleles were prevalent in cultivated strains (15 unique and a total of 27 alleles in 96 strains), whereas diverse A mating type alleles were possessed by wild strains (36 unique and a total of 48 alleles in 31 strains) [Citation14]. In this study, a total of 123 A mating type alleles were found from 106 wild strains, and more than 80% of which (102 alleles) were found only in wild strains. Taking together previous studies and current findings, only six A mating type alleles (A3, A20, A23, A25, A26, and A29) were unique to cultivated strains, whereas 103 A mating type alleles were unique to wild strains (). In addition, 124 A mating type alleles have been found in 137 wild strains, thereby supporting the hyper-diversity of A mating type alleles in wild strains of L. edodes.
3.2. Frequency of the occurrence of A mating type alleles in wild strains
We next investigated the occurrence of each A mating allele in wild strains. About half of the alleles discovered in wild strains showed a single occurrence (). In a previous study, most alleles (41 out of 48 alleles) in wild strains were observed only in one strain [Citation14], but we found that about half of the alleles in wild strains were found in multiple strains. Among recurrently observed alleles, only 20% (A1, A2, A4, A5, A7, A8, A10, A11, A17, A27, A28, and A30) were reported in cultivated strains. Consistent with the previous study [Citation14], allele A1 was most frequently found in wild strains of the Korean peninsula. Alleles A37 and A75 were the second most frequent alleles, and they have not yet been observed in cultivated strains. Nine alleles (A2, A5, A6, A8, A15, A21, A22, A28, A31), previously reported only in cultivated strains, were also observed in wild strains. Moreover, allele A6, observed only in Chinese strains, and alleles A21 and A22, observed only in Japanese strains, were found in Korean wild strains.
Table 2. Occurrence of A mating alleles in Korean wild strains of Lentinula edodes.
The occurrence of allele combinations in wild strains was also examined. About 90% of combinations were detected in only one strain (). The most frequent combination was A59A72, but it only appeared three times. In addition to A1A4 and A1A7, previously reported as the combination found both in cultivated and wild strains, A1A5, A1A10, and A11A28 were also found in wild and cultivated strains. Among them, A1A4, A1A5, and A1A7 pairs were preferentially found in cultivated strains [Citation14].
Table 3. Occurrence of A mating allele combinations in dikaryotic Korean wild strains of Lentinula edodes.
3.3. Regional distribution of A mating type alleles in wild strains
The Republic of Korea is located in the central and southern parts of the Korean peninsula. Our and previous collection sites of wild strains were distributed from Jeju Island through the southern region to the central region of the Korean peninsula (). Diverse A mating type alleles were intensively discovered in the central region where most of wild strains were collected ( and ). A total of 111 alleles and 89 unique alleles were found in this area. Thus, this mountainous area may be the major repository of diverse genetic resources of L. edodes in Korea. In the southern region, 12 alleles were exclusively found, and a total of 31 alleles were observed. Nineteen alleles were commonly found in the central and southern regions, including three alleles, A1, A4, and A5, which were prevalent in cultivated strains [Citation14]. Allele A7, the third frequent allele in cultivated strains [Citation14], was exclusively found in the southern region. Previously, Ha et al. suggested that cultivated strains might have been exposed to the wild in this area, based on the presence of cultivar-preferred alleles (A1, A4, and A7) and the fact that log-media-based cultivation has been conducted in this area [Citation14]. However, such alleles showed low frequency in our strains collected in this area (). In addition, A1A5, the allele combination of the widely used strain Mori290 for log cultivation, was found only once (). Therefore, we suppose that the exposure of cultivated strains to the wild might not be prevalent in Korea. Further research is needed to address this point.
Figure 2. Regional distribution of A mating type alleles in wild strains of Lentinula edodes of Korea. (A) Map of collection sites. Closed triangles indicate the mountains where wild strains were collected in this study and a previous study [Citation14]. (B) Venn diagram showing distinct and common A mating type alleles in different regions. The number and percentage of unique and common alleles are indicated in circles. All alleles identified in wild strains by this study and a previous study [Citation14] were included in the analysis.
![Figure 2. Regional distribution of A mating type alleles in wild strains of Lentinula edodes of Korea. (A) Map of collection sites. Closed triangles indicate the mountains where wild strains were collected in this study and a previous study [Citation14]. (B) Venn diagram showing distinct and common A mating type alleles in different regions. The number and percentage of unique and common alleles are indicated in circles. All alleles identified in wild strains by this study and a previous study [Citation14] were included in the analysis.](/cms/asset/3c9a295e-360e-4f9f-accb-622b17a3b112/tmyb_a_2161157_f0002_c.jpg)
Table 4. Regional distribution of A mating type alleles in Korean wild strains of Lentinula edodes.
Allele A119 was found to be unique in Jeju Island, isolated from the mainland. Alleles A10, A17, A27, and A63 were commonly found in Jeju Island and the central region. Although four alleles found in Jeju Island were also identified in the central region, only one allele found in Jeju Island was identified in the southern region, even though the southern region is closer to Jeju Island than the central region (). This finding might be due to the limited number of collections from Jeju Island. Notably, only allele A17 was observed throughout Korea. The hyper-diversity of A mating type alleles in wild strains is supported by the observation that only one allele was found throughout Korea.
3.4. Conserved sequences in the intergenic regions of A mating loci
In Basidiomycetes, A mating locus consists of a pair of divergently transcribed HD transcription factor genes and the intergenic region that functions as a bidirectional promoter [Citation13–17]. Three conserved motifs, ATTGT (ACAAT in reverse), ACAAT, and GCGGAGT, were reported in the intergenic region of A mating locus of L. edodes [Citation13,Citation14]. In verifying the presence of conserved motifs reported previously in the newly identified A mating type alleles and the presence of other conserved sequences, the intergenic region was aligned from ATTGT to ACAAT in all A mating type alleles except for three alleles (A38, A69, and A124), which highly vary in lengths. The sequence logo derived from 127 A mating type alleles demonstrated the conservation of the TCCCAC motif in addition to ATTGT, ACAAT, and GCGGAG motifs ().
Figure 3. Sequence logo for conserved motifs in the intergenic regions of A mating type loci of Lentinula edodes strains. The sequence logo was created by using the sequences of the region from ATTGT to GCGGAGT in the intergenic regions of 127 A mating type alleles. The position of bases and the information content measured in bits are indicated on the x- and y-axes, respectively.
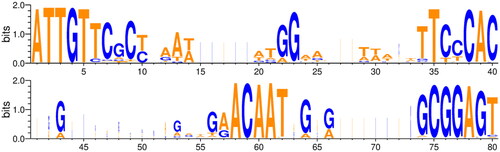
3.5. Sequence diversification of A mating type alleles
To obtain some clues on the mechanism for the diversification of A mating type alleles, we compared the sequences of 130 A mating type alleles. However, discovering any specific mechanism underlying the diversification of A mating type at a glance was difficult because of the high variability among alleles (Supplementary Figure S1) [Citation14]. Instead, we decided to compare similar sequences by aligning the sequences of different clones for the same allele. Allele A1 was selected for further investigation because it was the allele for that we acquired the most diverse clones. Although we expected that variations would primarily occur in the intergenic region, the comparison among the sequences of allele A1 showed the presence of single base transitions and in-frame insertion (or deletion) in the coding regions (). We next compared the sequences of alleles A67, A98, and A122, which have the most similar sequences to one another. Variations were concentrated in the promoter region, and single base transitions and in-frame insertions (or deletions) in the coding regions were observed (). One interesting feature was the observation of small blocks where the same sequences were shared between two alleles, implying recombination. Collectively, accumulated base transition, in-frame insertion (or deletion), and recombination would contribute to the diversification of A mating type alleles in L. edodes.
Figure 4. Sequence alignment of the A1 allele from different strains of Lentinula edodes. The sequences of allele A1 obtained from wild strains were aligned with the GenBank sequence JN129271.1 obtained from the Chinese strain L54A using MEGA 11. Putative start codons for HD1 and HD2 genes are indicated in green. Conserved motifs are indicated in red.
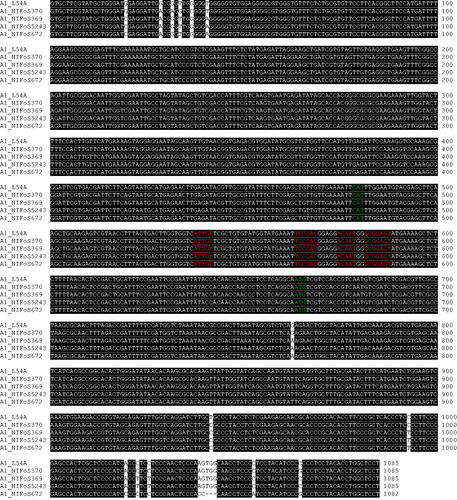
Figure 5. Sequence alignment of A67, A98, and A122 alleles of Lentinula edodes. The sequences of A67, A98, and A122 alleles were aligned using MEGA 11. Putative start codons for HD1 and HD2 genes are indicated in green. Conserved motifs are indicated in red. The regions that harboring sequences identical between two alleles with a length of more than 20 bp are highlighted in red boxes.
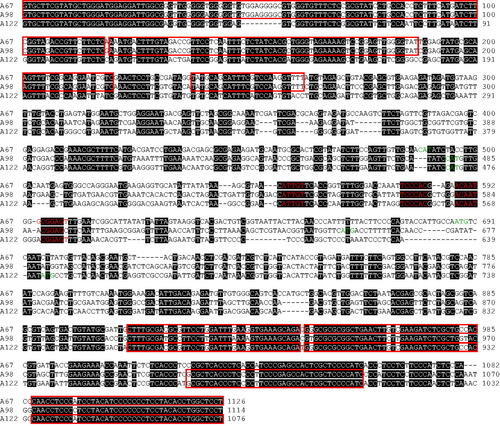
4. Discussion
4.1. Diversity of A mating type alleles in Korean wild strains
We extensively examined the diversity of A mating type alleles in Korean wild strains of L. edodes by sequence analysis on the variable region, representing a specific A mating type [Citation13,Citation14]. Two different A mating type alleles were determined in every 89 strains among 106 dikaryotic wild strains (). Most of these strains (76 out of 89 strains) had unique allele combinations (), indicating that the wild strains analyzed in this study are not recurrently collected but genetically diverse. Only a single allele was identified in the remaining 17 strains among 106 strains (). Based on the current findings and previous studies, a single allele could not be determined in 13.9% of wild dikaryotic strains (19 out of 137 strains), probably because of amplification failure caused by variations in the regions targeted by the primers used. Therefore, it is expected that more diverse A mating type alleles are present in the wild, and the development of new primer sets or genome sequencing of wild strains may be necessary to reveal such alleles.
Based on the present and previous study, 124 A mating type alleles were identified in 137 Korean wild strains ( and ). Including the cultivated strains, 130 A mating type alleles have been determined in L. edodes at present [Citation14]. The high degree of multiallelism of A mating type strongly supports its rapid evolution to increase the chance of finding a compatible mating partner [Citation3]. Compared with A mating type, a relatively small number of B mating types, 15 types, were identified in 111 strains of L. edodes [Citation12]. These findings consistent with the hypothesis that high selection pressure on A mating type locus, which is due to the importance of HD genes in the viability of fused cells [Citation3], has brought hyper-diversity of A mating type alleles of L. edodes in the wild as observed in other basidiomycetes [Citation22,Citation23]. The diversification of A mating type alleles would be achieved by accumulated base transition, in-frame insertion (or deletions), and recombination (). However, whether the variations are concentrated on A mating type locus and their underlying mechanism remain elusive. Genome-wide analysis on the mutation hot spot in L. edodes would provide comprehensive understanding of the molecular mechanism of A mating type allele diversification.
4.2. Prevalence of certain A mating type alleles in cultivated strains
Our collections revealed the presence of nine alleles (A2, A5, A6, A8, A15, A21, A22, A28, and A31), previously identified only in cultivated strains, in wild strains of Korea (). Considering that cultivars originated from wild strains, it seems reasonable that the more wild strains are analyzed, the more common alleles between wild and cultivated strains are revealed.
Only 21 of 124 alleles found in wild strains and 12 of 60 alleles found in more than two wild strains were selected in cultivated strains ( and ). Even the second most frequent alleles in the wild, A37 and A75, were not found in cultivated strains. These findings indicate the preferred selection of specific A mating types in cultivated strains, which was reported previously [Citation14]. One possible explanation for this is that specific A mating types are related to the characteristics suitable for cultivars, such as high-quality fruiting body or short cultivation period; therefore, they have been preferentially selected in cultivated strains. Reports on the presence of quantitative trait loci, related to mycelial growth rate and fruiting body-related traits such as pileus diameter and stipe length, in proximity to the A mating locus support this hypothesis [Citation24–26]. Notably, Ha et al. showed the dominant expression of HD1 encoded in A1, the most frequent allele in cultivated strains, in A1-containing dikaryotic strains [Citation14]. In addition, a recent RNA-seq-based linkage map construction study found that the expression of HD1 was significantly correlated with the pileus diameter [Citation26]. The high expression level of HD1 in A1 may have led to the preferential selection of A1 in cultivars to obtain large pileus.
However, it might not be the sole factor responsible for the prevalence of specific alleles in cultivars. Studies on wild and cultivated strains in Korea and China showed that the genetic diversity of cultivars is higher than that of wild strains in each country [Citation27,Citation28]. These data indicate that stable cultivars was developed by mating strains from different countries, and thus genetically distant, had been repeatably used for developing new varieties. Therefore, we think the limited number of strains used as a parent for developing cultivars has also contributed to the limited number of A mating type alleles in cultivated strains.
4.3. Conserved motifs in the promoter region of A mating locus
Our analysis newly identified the TCCCAC motif in the promoter region of A mating locus, in addition to the previously discovered ATTGT (ACAAT in reverse), ACAAT, and GCGGAG motifs () [Citation13,Citation14]. The ACAAT motif is part of the DNA binding site of yeast for MATα2, an HD transcription factor homologous to other sex-related genes, and for ROX1, a hypoxic gene-repressor containing a high-mobility-group DNA binding motif [Citation29,Citation30]. The TCCCAC motif is reported in animals as the binding site for two transcription factors, Runx2 and MNRR1, which are involved in osteogenesis and breast carcinogenesis, respectively [Citation31–33]. However, proteins recognizing TCCCAC or GCGGAG have not been documented in fungi yet.
The motifs TCCCAC and ACAAT were also reported in the A mating type locus of Pleurotus sp., including P. eryngii, P. ostreatus, and P. tuoliensis [Citation34]. On contrary, the GCGGAG motif was specific to L. edodes, and the GCCG motif was specific to Pleurotus. Collectively, there would be the conserved and divergent regulatory mechanism of HD genes in A mating locus in mushrooms.
In summary, we discovered 67 unidentified A mating type alleles from 106 dikaryotic wild strains collected from Korea. Our study revealed the hyper-diversity of A mating type alleles in wild strains of L. edodes in Korea. Further research on the sequences of each allele obtained from the present and previous studies could provide insight into the evolutionary mechanism of multiallelism of A mating type in tetrapolar basidiomycetes and the regulatory process of HD genes residing in A mating locus. Moreover, marker development based on the sequences of A mating type alleles will facilitate the breeding of new cultivars using diverse wild strains.
Supplemental Material
Download PDF (1.2 MB)Acknowledgements
The authors sincerely appreciate Prof. Hyeon-Su Ro and Dr. Sinil Kim at Gyeongsang National University for providing critical advice on the sequence analysis. The authors also thank Sang-Woo Lee for assisting with PCR amplifications and Sujung Yoo for helping to map the collection sites using QGIS.
Disclosure statement
No potential conflict of interest was reported by the authors.
Additional information
Funding
References
- Casselton LA. Mate recognition in fungi. Heredity (Edinb). 2002;88(2):142–147.
- Ni M, Feretzaki M, Sun S, et al. Sex in fungi. Annu Rev Genet. 2011;45:405–430.
- Nieuwenhuis BPS, Billiard S, Vuilleumier S, et al. Evolution of uni- and bifactorial sexual compatibility systems in fungi. Heredity (Edinb). 2013;111(6):445–455.
- Royse DJ, Baars J, Tan Q. Current overview of mushroom production in the world. In: Zied DC, Pardo-Giménez A, editors. Edible and medicinal mushrooms: technology and applications. Hoboken: John Wiley & Sons Ltd; 2017. p. 5–13.
- Bak WC, Park JH, Park YA, et al. Determination of glucan contents in the fruiting bodies and mycelia of Lentinula edodes cultivars. Mycobiology. 2014;42(3):301–304.
- Gaitán-Hernández R, López-Peña D, Esqueda M, et al. Review of bioactive molecules production, biomass, and basidiomata of shiitake culinary-medicinal mushrooms, Lentinula edodes (Agaricomycetes). Int J Med Mushrooms. 2019;21(9):841–850.
- Shah SK, Walker PA, Moore-Olufemi SD, et al. An evidence-based review of a Lentinula edodes mushroom extract as complementary therapy in the surgical oncology patient. JPEN J Parenter Enteral Nutr. 2011;35(4):449–458. Jul
- Chang S, Miles P. Historical record of the early cultivation of Lentinula in China. Mushroom J Trop. 1987;7:31–37.
- Bohra A, Kilian B, Sivasankar S, et al. Reap the crop wild relatives for breeding future crops. Trends Biotechnol. 2022;40(4):412–431.
- Renzi JP, Coyne CJ, Berger J, et al. How could the use of crop wild relatives in breeding increase the adaptation of crops to marginal environments? Front Plant Sci. 2022;13:886162.
- Wu L, van Peer A, Song W, et al. Cloning of the Lentinula edodes B mating-type locus and identification of the genetic structure controlling B mating. Gene. 2013;531(2):270–278.
- Ha BS, Moon YJ, Song Y, et al. Molecular analysis of B mating type diversity in Lentinula edodes. Sci Hortic. 2019;243:55–63.
- Au CH, Wong MC, Bao D, et al. The genetic structure of the A mating-type locus of Lentinula edodes. Gene. 2014;535(2):184–190.
- Ha B, Kim S, Kim M, et al. Diversity of A mating type in Lentinula edodes and mating type preference in the cultivated strains. J Microbiol. 2018;56(6):416–425.
- Banham AH, Asante-Owusu RN, Gottgens B, et al. An N-terminal dimerization domain permits homeodomain proteins to choose compatible partners and initiate sexual development in the mushroom Coprinus cinereus. Plant Cell. 1995;7(6):773–783.
- James TY, Liou SR, Vilgalys R. The genetic structure and diversity of the a and B mating-type genes from the tropical oyster mushroom, Pleurotus djamor. Fungal Genet Biol. 2004;41(8):813–825.
- Wang W, Lian L, Xu P, et al. Advances in understanding mating type gene organization in the mushroom-forming fungus Flammulina velutipes. G3 (Bethesda). 2016;6(11):3635–3645.
- Park YA, Seo S, Ka KH. Cultural characteristics and morphological comparison of the wild mushroom Lentinula edodes cultivated on sawdust substrate. Kor J Mycol. 2018;46(2):177–185.
- Schneider TD, Stephens RM. Sequence logos: a new way to display consensus sequences. Nucleic Acids Res. 1990;18(20):6097–6100.
- Crooks GE, Hon G, Chandonia JM, et al. WebLogo: a sequence logo generator. Genome Res. 2004;14(6):1188–1190.
- Stothard P. The sequence manipulation suite: javaScript programs for analyzing and formatting protein and DNA sequences. Biotechniques. 2000;28(6):1102–1104.
- Pardo EH, O'Shea SF, Casselton LA. Multiple versions of the A mating type locus of Coprinus cinereus are generated by three paralogous pairs of multiallelic homeobox genes. Genetics. 1996;144(1):87–94.
- Schirawski J, Heinze B, Wagenknecht M, et al. Mating type loci of Sporisorium reilianum: novel pattern with three A and multiple B specificities. Eukaryot Cell. 2005;4(8):1317–1327.
- Gong WB, Liu W, Lu YY, et al. Constructing a new integrated genetic linkage map and mapping quantitative trait loci for vegetative mycelium growth rate in Lentinula edodes. Fungal Biol. 2014;118(3):295–308.
- Gong WB, Li L, Zhou Y, et al. Genetic dissection of fruiting body-related traits using quantitative trait loci mapping in Lentinula edodes. Appl Microbiol Biotechnol. 2016;100(12):5437–5452.
- Zhang L, Gong W, Li C, et al. RNA-Seq-based high-resolution linkage map reveals the genetic architecture of fruiting body development in shiitake mushroom, Lentinula edodes. Comput Struct Biotechnol J. 2021;19:1641–1653.
- Xiang X, Li C, Li L, et al. Genetic diversity and population structure of Chinese Lentinula edodes revealed by InDel and SSR markers. Mycol Prog. 2016;15:37.
- Lee HY, Moon S, Ro HS, et al. Analysis of genetic diversity and population structure of wild strains and cultivars using genomic SSR markers in Lentinula edodes. Mycobiology. 2020;48(2):115–121.
- Kurischko C, Schilhabel MB, Kunze I, et al. The MATA locus of the dimorphic yeast Yarrowia lipolytica consists of two divergently oriented genes. Mol Gen Genet. 1999;262(1):180–188.
- Balasubramanian B, Lowry CV, Zitomer RS. The Rox1 repressor of the Saccharomyces cerevisiae hypoxic genes is a specific DNA-binding protein with a high-mobility-group motif. Mol Cell Biol. 1993;13(10):6071–6078.
- Gaur T, Lengner CJ, Hovhannisyan H, et al. Canonical WNT signaling promotes osteogenesis by directly stimulating Runx2 gene expression. J Biol Chem. 2005;280(39):33132–33140.
- Choi H, Kim TH, Yang S, et al. A reciprocal interaction between β-Catenin and osterix in cementogenesis. Sci Rep. 2017;7(1):8160.
- Aras S, Maroun MC, Song Y, et al. Mitochondrial autoimmunity and MNRR1 in breast carcinogenesis. BMC Cancer. 2019;19(1):411.
- Lee SH, Ali A, Ha B, et al. Development of a molecular marker linked to the A4 locus and the structure of HD genes in Pleurotus eryngii. Mycobiology. 2019;47(2):200–206.