Abstract
Termitomyces sp. that grow in symbiosis with fungus-farming Termites have medicinal properties. However, they are rare in nature, and their artificial culture is challenging. The expression of AXL receptor tyrosine kinase and immune checkpoint molecules favor the growth of cancer cells. The study evaluated the optimal conditions for the artificial culture of Termitomyces and their inhibitory activity on AXL and immune checkpoint molecules in lung adenocarcinoma and melanoma cell lines. The culture of 45 strains of Termitomyces was compared. Five strains with marked growth rates were selected. Four of the selected strains form a single cluster by sequence analysis. The mycelium of 4 selected strains produces more fungal mass in potato dextrose broth than in a mixed media. The bark was the most appropriate solid substrate for Termitomyces mycelia culture. The mycelium of all five selected strains showed a higher growth rate under normal CO2 conditions. The culture broth, methanol, and ethyl acetate of one selected strain (T-120) inhibited the mRNA relative expression of AXL receptor tyrosine kinase and immune checkpoint molecules in cancer cell lines. Overall, these results suggest the potential usefulness of Termitomyces extracts as a co-adjuvant therapy in malignant diseases.
1. Introduction
Termitomyces sp. or termite mushrooms belong to the kingdom Fungi, Basidiomycota phylum, Lyophyllaceae family, and the order Agaricales [Citation1]. They have a unique symbiotic association with Macrotermitinae Termites [Citation2,Citation3]. They only live in a medium called the fungus comb inside the Termite underground nest made by termite workers from incompletely digested plant materials [Citation3]. The fungus forms a mass of white mycelium called a nodule that helps Termites digest and consume cellulosic materials [Citation3,Citation4]. Approximately 30 species of Termitomyces differing in basidiocarp and basidiospore morphology have been isolated [Citation5]. Termitomyces are not only superior in taste but also more highly rich in nutrients, including proteins (27–36%), ascorbic acid (10–18 mg/g), and ergosterol compared to other edible mushrooms [Citation6–8]. They are geographically distributed throughout Africa and Southeast Asia’s tropical and subtropical areas [Citation1]. However, they generally remain unrecognizable in Japan due to their restricted distribution (Yaeyama and Okinawa Islands) and limited harvest period [Citation2,Citation9,Citation10].
The medicinal properties of Termitomyces have recently been the focus of many investigations [Citation11]. Reports have shown favorable effects of the mushroom constituents in rheumatism, high blood pressure, obesity, Kwashiorkor, and diarrhea [Citation12]. In addition, the phenolic compounds and polysaccharides from the fruiting body of Termitomyces possess antioxidant, anti-inflammatory, antibacterial, and anticancer activities [Citation12–19]. Due to its scarcity, artificial cultivation would be the ideal approach to collect a sufficient amount of this mushroom for medicinal and commercial applications. Unfortunately, Termitomyces typically generate no fruiting bodies in artificial cultivation. However, the successful growth of Termitomyces mycelia in agar media has recently been reported [Citation20]. Therefore, optimizing the mycelium growth condition could make the artificial cultivation of these fungi possible. In addition, because the growth of the mushroom fruiting body is difficult, solid-state cultivation requires a long period and is generally non-reproducible. Cultivating mycelia by submerged fermentation in a defined medium appears to be a promising substitute to improve reproducibility and obtain identical fungal biomass with consistent quality [Citation21].
Several studies have demonstrated the anticancer properties of mushrooms [Citation22,Citation23]. The extracts and derivatives of mushrooms have been reported to suppress the proliferation of malignant cells in vitro and improve cancer response to cytotoxic therapy [Citation24,Citation25]. Anticancer activity of Termitomyces sp. has also been reported. Anticancer activity of Termitomyces sp. derivatives has been reported against several cancer cell lines from the liver, lungs, melanoma, colon, ovary, prostate, kidneys, central nervous system, breast, and leukemia [Citation11,Citation18,Citation26–28]. However, the mechanism of the anticancer activity of mushroom-derived compounds is unclear. Recent studies suggest that the beneficial effects of mushrooms may depend on their inhibitory activity on the expression of the AXL receptor, programmed death-ligand 1 (PD-L1), and PD-L2 [Citation26,Citation29–32]. AXL is a cell-surface tyrosine kinase receptor, and PD-L1 and PD-L2 are immune checkpoint molecules [Citation33,Citation34]. AXL receptor, PD-L1, and PD-L2 suppress the host immune response [Citation33,Citation34]. Cancer cells evade the host immune response by expressing high levels of AXL, PD-L1, and PD-L2 [Citation33,Citation34].
The objective of the present study was to determine the optimal conditions to culture Termitomyces sp. and to evaluate whether the extracts of Termitomyces sp. can exert anticancer activity by inhibiting the expression of AXL receptor, PD-L1, and PD-L2.
2. Materials and methods
2.1. Collection of mushrooms
The mycelium from 45 strains of Termitomyces sp. were collected from Iriomote, Naha, and Ishigaki (Okinawa Islands, Japan) (). In addition, the fruiting body of Termitomyces sp. was collected from Ishigaki, Okinawa, Japan, to prepare methanol and hot water extracts ().
Table 1. Sequence and geographic origin of each selected Termitomyces strain.
2.2. Evaluation of the growth rate of Termitomyces sp. mycelium in culture and strain selection
The Termitomyces sp. mycelia were separately inoculated into a newly prepared agar medium (glucose 2 g, malt extract 2 g, yeast extract 1 g, potato dextrose broth 1 g, and agar 5 g, 300 mL of distilled water) in triplicates (three plates per each strain) and incubated at 25 °C for 30 days. The growth rate was monitored, and the size in diameter was measured. Five of the 45 strains were selected for subsequent experiments based on the growth rate and mycelium characteristics (smooth mycelium surface, radial and uniform growth of mycelium). The presence of a clamp connection was checked in all selected strains to confirm the dikaryon stage of the mycelium.
2.3. Evaluation of molecular phylogeny based on the internal transcribed spacers regions (ITS) of the 5.8S ribosomal RNA (rRNA) gene
The five selected strains (T-14, T-26, T-76, T-108, T-120) were used for sequence-based molecular identification using the internal transcribed spacer regions of the 5.8S rRNA gene (ITS-5.8S rRNA gene). The sequences were identified by BLAST search. A phylogenetic tree was prepared to show the phylogenetic relationship among the strains. The phylogenetic tree was constructed using the MEGAX software with the maximum likelihood method and the Kimura 2-parameter model.
2.4. Comparative evaluation of mycelium growth in culture using two different liquid media
The growth of the mushroom’s mycelium in PDB (potato dextrose broth; Difco Laboratories, Detroit, MI) and mixed-media (glucose, malt extract, yeast extract, potato dextrose broth) was compared in triplicates (three cultures per strain) after incubation at 25 °C for 30 days. The cultured mycelia were then freeze-dried, and the dry weight was measured.
2.5. Evaluation of the growth rate of mycelia in different solid substrates
Three types of commercially available solid media for mushroom cultivation were used to evaluate the growth of Termitomyces mycelia of each strain. The cultures were performed at 25 °C in a dark environment for 45 days. The solid media were the following:
Sawdust media: sawdust 26.25 g (Queras acutissima supplied by a mushroom cultivator from Miyazaki Prefecture, Japan), rice bran 8.75 g, water 65 mL, pH 5.0.
Bark media: bark 26.25 g, rice bran 8.75 g, water 65 mL, pH 5.7
Peat moss media: peat moss 26.25 g, rice bran 8.75 g, water 65 mL (peat moss was mixed with CaCO3 in a ratio of 70:2 g), pH 4.0.
The substrates were directly used without size determination. The glass test tubes (diameter 28 mm, length 200 mm) were filled with 50 g of each solid substrate and then sterilized at 121 °C for 30 min. The strain mycelium taken from the PDA agar media was then inoculated into the solid substrate in the center. The final pH of each substrate was determined using a HORIBA F-51 pH meter (HORIBA Ltd., Kyoto, Japan) after 50 g of the solid substrate was agitated with distilled water. Finally, the mycelial growth length was measured using an electronic caliper. The results were expressed as the means of three replicates.
2.6. Evaluation of mycelia growth under high CO2 concentration
The five selected strains of Termitomyces mycelium were cultured under normal air conditions or at high (5%) CO2 levels at 25 °C for 30 days. The mycelial growth was then compared between the two conditions.
2.7. Preparation of mushroom extracts
The following materials were prepared from Termitomyces sp. for evaluating their inhibitory effect on the expression of AXL, PD-L1, and PD-L2 in cancer cells: freeze-dried culture broth from the mycelium of the five selected strains (T-14, T-26, T-76, T-108, T-120), methanol extracts from the mycelium of strains T-108, T-120, and the fruiting body of a Termitomyces sp., ethyl acetate extract from strains T-108, and T-120, and hot water extract from the fruiting body of a Termitomyces sp.
For methanol extraction, five strains of Termitomyces sp. mycelium from the liquid culture, including the culture broth and a piece of the termite nest, were freeze-dried in 50 mL test tubes. Then the dried products were crushed into fine pieces using a glass rod before homogenizing overnight with 40 mL methanol, followed by centrifugation. After repeating the extraction process three times, the supernatant was evaporated using a rotary evaporator, and the extracted powder was further dried by blowing nitrogen gas and freeze-dried. The fruiting body of 59 g was freeze-dried to a dry weight of 9.4 g and pulverized before homogenizing overnight with 200 mL methanol, followed by centrifugation and filtration. After three times of extraction, the supernatant was dried using a rotary evaporator, blowing nitrogen gas, and then freeze-dried.
For ethyl acetate extraction, the methanol extract from the Termitomyces sp. mycelium and the culture broth was extracted by directly adding ethyl acetate into the methanol extract powder, followed by shaking and centrifugation. After repeating three times the same procedure, the supernatant was collected and dried using a rotary evaporator. The extracted powder was further dried by blowing nitrogen gas.
For hot water extraction, the fruiting body of 59 g was freeze-dried to a dry weight of 9.4 g. The dried fruiting body was then pulverized, homogenized in 200 mL of water, and then extracted by autoclave (100 °C, 30 min). The extract was then centrifuged, and the supernatant was filtered and freeze-dried to get the final extract powder.
2.8. Culture of cell lines and treatment
The human A549 lung adenocarcinoma cell line and the mouse melanoma cell line B16F10 were from the American Type Culture Collection (Manassas, VA, USA). Dulbecco’s Modified Eagle Medium (DMEM) was purchased from Sigma–Aldrich (Saint Louis, MO, USA), and the fetal bovine serum (FBS) from Bio Whittaker (Walkersville, MD, USA). L-glutamine, penicillin, and streptomycin were from Invitrogen (Carlsbad, CA, USA). A549 cells and B16.F10 cells were maintained in RPMI-1640 medium (Nacalai Tesque, Kyoto, Japan) and DMEM (low glucose), respectively, supplemented with 10% heat-inactivated fetal bovine serum (Biowest, Nuaillé, France), penicillin (100 IU/mL), and streptomycin (100 mg/mL). Cell culture was performed at 37 °C in a humidified incubator containing 5%CO2. For the treatment with mushroom extracts, cells were seeded onto a 12-well plate at a density of 1 × 105 cells/well, cultured until becoming semi-confluent, and serum-starved overnight in RPMI-1640 with 1% fetal bovine serum. Mushroom extracts were added to a final concentration of 20 μg/mL mushroom extracts and cultured for 24 h. The methanol and ethyl acetate extracts from PDB and sterile physiological saline were used as negative controls, and the fruiting body alkaline extract from Agaricus blazei Murrill was used as a positive control in the experiments [Citation32].
2.9. RT-PCR
The cells were treated for 24 h, harvested, and then total RNA was extracted using Sepasol-RNA I SuperG (guanidinium thiocyanate-phenol-chloroform mixture; Nacalai tesque) reagent followed by chloroform-isopropanol extraction and ethanol precipitation. Subsequently, 1 μg of extracted RNA was reverse transcribed into cDNA using ReverTraAce reverse transcriptase (Toyobo Life Science, Osaka, Japan). describes the primers used to carry out RT-PCR. PCR was performed under the following conditions: cycles between 26 and 35, denaturation at 94 °C for 15 s, annealing at 65 °C for 30 s, elongation at 72 °C for 1 min, followed by a further extension at 72 °C for 5 min. The products of PCR were run on 1.5% agarose gel, stained with ethidium-bromide staining, and quantified by Image J software (National Institute of Health, Bethesda, ML, USA). The mRNA expression was normalized against the glyceraldehyde 3-phosphate dehydrogenase (GAPDH) mRNA expression.
Table 2. The primers used for RT-PCR.
2.10. Detection of apoptosis
The effects of mushroom extracts on the apoptosis of A549 cells were analyzed by flow cytometry. Twenty-four hours after treatment, cells were harvested and resuspended in annexin V-binding buffer containing 5 μg/mL FITC-labeled annexin V (BioLegend, San Diego, CA, USA), 100 μg/mL propidium iodide, and incubated for 20 min at room temperature [Citation35,Citation36]. Stained cells were analyzed using a FACScan flow cytometer (Becton Dickinson, San Jose, CA, USA) and CellQuest Pro software (Becton Dickinson).
2.11. Statistical analysis
Data are expressed as mean ± standard deviation (S.D.). Statistical difference between three or more variables was evaluated by one-way analysis of variance (ANOVA) with Dunnett’s test. The difference between the two variables was assessed by the Student t-test. For the statistical analysis, we used Graph-pad Prism version 9.0 (San Diego, CA, USA). A p < 0.05 was considered significant.
3. Results
3.1. Mycelia from 45 strains of Termitomyces sp. show differential growth rates in agar medium
The growth rates in agar media of mycelia from 45 strains of Termitomyces sp. were compared. There were marked differences in the growth rates of mycelia from the 45 strains. In particular, the growth rate of strains 14 (T-14), 26 (T-26), 76 (T-76), 108 (T-108), and 120 (T-120) was higher than other strains. In addition, the mycelia of T-14, T-26, T-76, T-108, and T-120 strains showed uniform radial growth and smooth and no bumpy surface. Therefore, these five strains were selected for subsequent experiments based on these mycelial characteristics and growth rates. Furthermore, the presence of a clamp connection under microscopic confirmed the dikaryon stage of the five selected strains ().
Figure 1. Mycelia of several strains of Termitomyces show differential growth in agar medium. (A) Mycelia of 45 strains of Termitomyces were collected from the Okinawa islands and cultured in agar medium under normal CO2 conditions at 25 °C for 30 days. The longest diameter was then measured and compared. N = 9 for each strain. Data are expressed as mean ± S.D. Red bars indicate selected strains; (B) Photographs of cultured mycelium from each selected strain. The scale bar indicates 3 cm; (C) The red circles in microphotographs indicate the clamp connection. Scale bars indicate 200 µm; (D) Photographs of the mycelium of each non-selected strain.
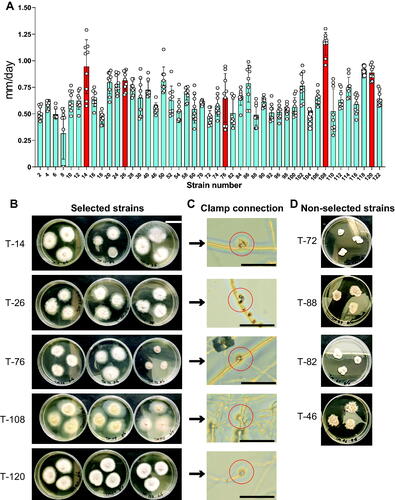
3.2. Strains with significant growth rates show high phylogenetic identity with Termitomyces sp.
The gene sequence of the strains was determined, and their identity was searched in the GenBank by BLAST. Termitomyces sp. showed the maximum identity with the sequence of the five selected strains. The sequence of strain T-26 (570 base pair) showed a maximum score with Termitomyces sp. YO198 in 18S rRNA, ITS1, 5.8S rRNA, ITS2, 28S rRNA, partial and complete sequence (AB968241.1) with an identity of 99.47%. The sequence of strains T-14, T-76, and T-120 showed 99.82–100% identity with Termitomyces sp. YO198 (AB968241.1). Strain T-108 (638 base pairs) hit the maximum score for Termitomyces sp. strain BD4 small subunit ribosomal RNA gene, partial sequence, ITS1, 5.8S rRNA gene, and ITS2, complete sequence; and large subunit ribosomal RNA gene, partial sequence (MF163149.1) with an identity of 99.22%. The sequence of strains T-14, T-26, T-76, T-120, and Termitomyces sp. YO198 (AB968241.1) belongs to a single cluster. However, strain T-108 was separately clustered with Termitomyces sp. strain BD4 (MF163149.1) (; ).
Figure 2. Phylogenetic analysis of Termitomyces sp. The sequence of each selected strain was determined, and then the sequence identity was searched in GenBank using BLAST. Twenty-nine internal transcribed spacer’s DNA was inferred based on bootstrap and maximum likelihood analysis. The red squares indicate clusters. Species in blue represent sequenced strains. The numbers on branches indicate the bootstrap values of the parsimony analysis from 1000 replicates. The MEGAX software by maximum likelihood method and the Kimura 2-parameter model was used. Agaricus abruptibulbus was used as an outgroup.
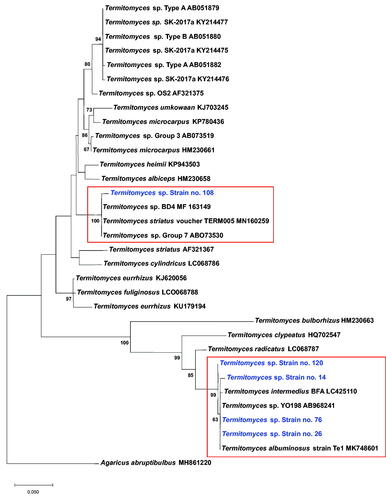
3.3. Significant stimulation of fungal growth by PDB media
The growth rate of mushrooms in two liquid media, PDB and mixed media, was compared. The mycelium cultured in PDB media produced more fungal mass than mycelium grown in mixed media in all strains except strain T-14. This strain T-14 showed a significantly lower mass in PBD than in mixed-media ().
Figure 3. Significant stimulation of fungal growth in potato dextrose broth. (A) The mycelia were cultured in potato dextrose broth (PDB) mixed-media (glucose, malt extract, yeast extract, and potato dextrose broth) at 25 °C for 30 days; (B) The mycelia were then collected, lyophilized, and their dry weight was measured. n = 3 for each strain. Data are expressed as mean ± S.D. Statistical analysis by Student t-test. *p < 0.05; **p < 0.01.
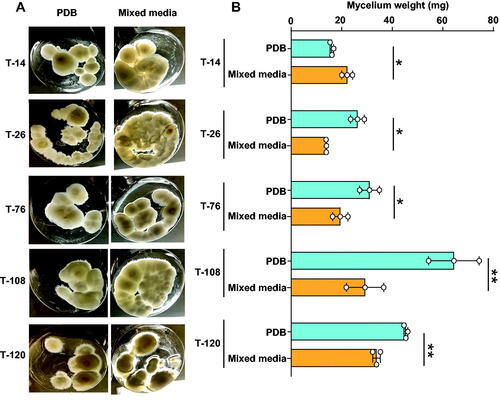
3.4. The bark is the most optimal solid substrate for the artificial culture of Termitomyces sp.’s mycelium
The growth of mycelium in media containing sawdust, peat moss, or bark was compared. The mycelia of strains T-14, T-26, and T-120 showed no growth in sawdust media. On the other hand, the mycelium of strain T-14 showed a fast growth (0.54 mm/day) in peat moss-containing media compared to other strains (0.31–0.37 mm/day). The mycelium from all strains grew at an equal level in solid media containing bark with a growth rate between 0.35 and 0.45 mm/day ().
Figure 4. Comparative evaluation of culture conditions for Termitomyces mycelium. (A) Photographs of the mycelium of strain T-108 cultured in a medium containing, from left to right, sawdust, peat moss, or bark; (B) The growth was defined as how deep the mycelium can vertically grow toward the solid media containing each substrate. Strains T-14, T-26, and T-120 showed no growth in sawdust; (C) The mycelium was cultured under normal (0.03–0.04%) or high (5%) CO2 conditions, and the fungal growth was compared. Data are expressed as mean ± S.D. Statistical analysis by Student t-test. *p < 0.05; **p < 0.01.
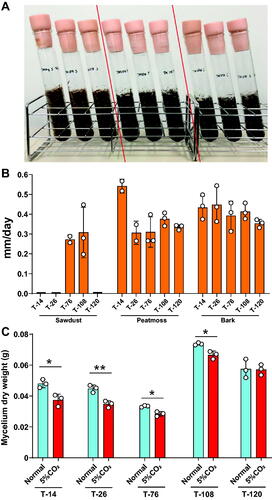
3.5. Mycelium grows better under normal CO2 conditions
The mycelium was cultured under normal (0.03–0.04%) or high (5%) CO2 conditions, and the fungal growth was compared. Strain T-14, T-26, T-76, and T-108 showed significant growth improvement under normal CO2 conditions compared to growth under high CO2 conditions. Only strain 120 showed no significant difference between normal and high CO2 conditions ().
3.6. The freeze-dried culture broth supernatant of strain T-120 inhibits the expression of AXL and immune checkpoint molecules
The freeze-dried culture broth supernatant of strain T-120 significantly inhibited the mRNA relative expression of AXL and PD-L2 in lung A549 adenocarcinoma cell lines compared to saline control. The inhibitory activity of the freeze-dried culture broth supernatant from T-120 on AXL mRNA expression was significantly higher than the positive control. The PD-L1 mRNA expression was also inhibited by the freeze-dried culture broth supernatant of strain T-120 in A549 cells compared to the control, but the inhibition was not statistically significant. The freeze-dried culture broth supernatant from strains T-14, T-26, and T-108 induced no significant inhibition of AXL, PD-L1, or PD-L2 compared to saline control ().
Figure 5. The freeze-dried culture broth supernatant of strain T-120 inhibits the expression of AXL and immune checkpoint molecules. A549 cells were cultured in the presence of the freeze-dried culture broth supernatant from strains T-14, T-26, T-76, T-108, and T-120. Cells treated with an alkaline extract from Agaricus blazei Murril’s fruiting body were used as positive controls, and cells treated with physiological saline (SAL) alone were used as negative controls. The total RNA was extracted from each treatment group, and the relative expression of AXL, PD-L1, and PD-L2 was evaluated by RT-PCR. Data are the mean ± S.D. Statistical analysis by ANOVA with Dunnett’s test. *p < 0.05 vs. saline; †p < 0.05 vs. Agaricus blazei fruiting (fr.) body extract.
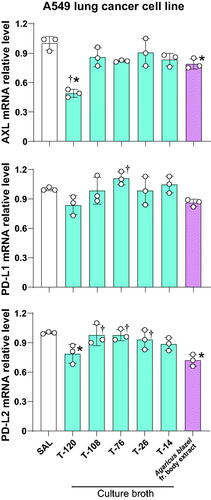
3.7. The methanol extracts of the mycelium and culture broth supernatant from strain T-120 inhibit the expression of AXL and immune checkpoint molecules
The methanol extracts of the mycelium and culture broth supernatant from strains T-108 and T-120 were prepared and used to treat A549 lung cancer and B16.F10 melanoma cell lines. Saline and the methanol extract of PDB were used as negative controls. The methanol extracts from strain T-120 mycelium and its culture broth supernatant significantly inhibited the mRNA expression of AXL, PD-L1, and PD-L2 compared to saline control in A549 cells. The methanol extracts of the mycelium, but not that of the culture broth from strain T-108, significantly inhibited the AXL mRNA expression in A549 cells. However, the methanol extracts from strain T-108 did not significantly affect PDL-1 or PDL-2 expression in A549 cells ().
Figure 6. The methanol extracts of the mycelium and culture broth supernatant from strain T-120 inhibit the expression of AXL and immune checkpoint molecules. (A,B) B16.F10, and A549 cells were cultured in 12-well plates for 24 h in the presence of methanol extracts from mycelia and culture broth supernatant from strains T-108 and T-120, or the methanol extract from the potato dextrose broth. The expression of Axl and checkpoint molecules was evaluated by RT-PCR. Cells cultured in the presence of saline and potato dextrose broth were the negative controls, and cells cultured in the presence of extract from Agaricus blazei Murril (20 µg/ml) were the positive controls. Data are expressed as the mean ± S.D. n = 3 in each treatment group. Statistical analysis by ANOVA with Dunnett’s test. PD-L1: programmed death-ligand 1; PD-L2: programmed death-ligand 2. *p < 0.05 vs. saline. †p < 0.05 vs. Agaricus blazei fruiting (fr.) body extract.
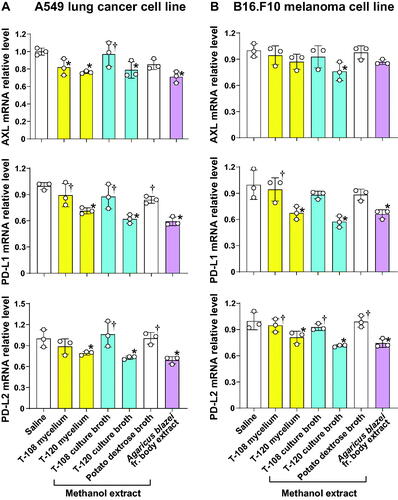
The methanol extracts from strain T-120 mycelium, and its culture broth supernatant significantly inhibited the mRNA expression of PD-L1 and PD-L2 compared to saline control in B16.F10 melanoma cell lines. The AXL mRNA expression was significantly inhibited by the methanol extract of strain T-120’s culture broth supernatant in melanoma cell lines. The methanol extract of T-120 mycelium inhibited AXL mRNA expression, but the inhibition was not statistically significant in melanoma cells. The methanol extract of strain T-108’s culture broth supernatant significantly inhibited PD-L1 mRNA expression but did not inhibit the mRNA expression of AXL or PD-L2 in melanoma cell lines. The methanol extract of T-108 mycelium showed no significant inhibitory activity ().
3.8. The ethyl acetate extract of strain T-120’s culture broth supernatant and the methanol extract of Termitomyces sp.’s fruiting body inhibit the expression of AXL, PD-L1, and PD-L2
A549 cells were cultured in the presence of the ethyl acetate extract of the culture broth supernatant, mycelium from strain T-120, and the culture broth supernatant from strain T-108. The mRNA expression of AXL, PD-L1, and PD-L2 was evaluated. The ethyl acetate extract of strain T-120’s culture broth supernatant and the methanol extract of Termitomyces sp.’s fruiting body significantly inhibited the mRNA expression of AXL, PD-L1, and PD-L2 compared to saline control. The hot water extract of Termitomyces sp.’s fruiting body and the ethyl acetate extract of strain T-120 mycelium significantly inhibited the mRNA expression of PD-L1 but not that of AXL or PD-L2 compared to saline control. The ethyl acetate extract of strain T-108 showed no inhibitory activity ().
Figure 7. The ethyl acetate extract of strain T-120’s culture broth supernatant and the methanol extract of Termitomyces fruiting body inhibit the expression of AXL and immune checkpoint molecules. A549 cells were cultured in 12-well plates for 24 h in the presence of the ethyl acetate extract of the culture broth supernatant and mycelium from strain T-120 and the ethyl acetate extract of the culture broth supernatant from strain T-108. A549 cells were also stimulated with the methanol and hot water extracts from the Termitomyces fruiting body. Cells cultured in the presence of saline and potato dextrose broth were the negative controls, and cells cultured in the presence of extract from Agaricus blazei Murril were the positive controls. The mRNA expression of AXL, PD-L1 and PD-L2 was evaluated by RT-PCR. Data are expressed as the mean ± S.D. n = 3 in each treatment group. Statistical analysis by ANOVA with Dunnett’s test. PD-L1: programmed death-ligand 1; PD-L2: programmed death-ligand 2. *p < 0.05 vs. saline. †p < 0.05 vs. Agaricus blazei fruiting (fr.) body extract.
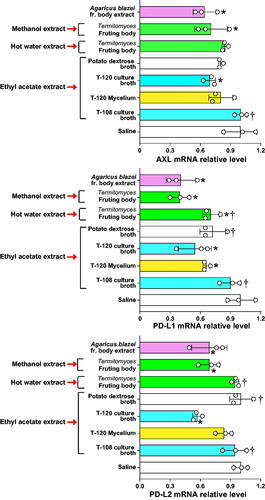
3.9. Induction of apoptosis
The freeze-dried culture broth extract of strain 120 increased apoptosis of A549 lung cancer cell lines compared to saline control, but the inhibition was not statistically significant. The freeze-dried of the culture broth from T-108, T-76, T-26, and T-14 had no direct effect on the apoptosis of A549 cells (). The methanol extract, but not the hot water extract, of the fruiting body from Termitomyces significantly induced apoptosis of A549 cells compared to saline control. The ethyl extract of the T-120 mycelium and the ethyl acetate extract of the culture broth from T120 and T-108 had no direct effect on the apoptosis of A549 cells ().
Figure 8. Induction of apoptosis by the freeze-dried culture broth of strain T-120 and methanol extract of Termitomyces. A549 cells were cultured for 24 h in the presence of freeze-dried culture broth from each selected mushroom strain (A,B) or in the presence of ethyl acetate extract of the culture broth from T-108 or T-120, ethyl acetate extract of T-120 mycelium, the hot water or methanol extract (B,C) of Termitomyces. Cells cultured in the presence of saline were the negative controls, and cells cultured in the presence of extract from Agaricus blazei Murril were the positive controls. Apoptosis was evaluated by flow cytometry. Data are expressed as the mean ± S.D. n = 3 in each treatment group. Statistical analysis was performed by ANOVA with Dunnett’s test. PD-L1: programmed death-ligand 1; PD-L2: programmed death-ligand 2. *p < 0.05 vs. saline. †p < 0.05 vs. Agaricus blazei fruiting (fr.) body extract.
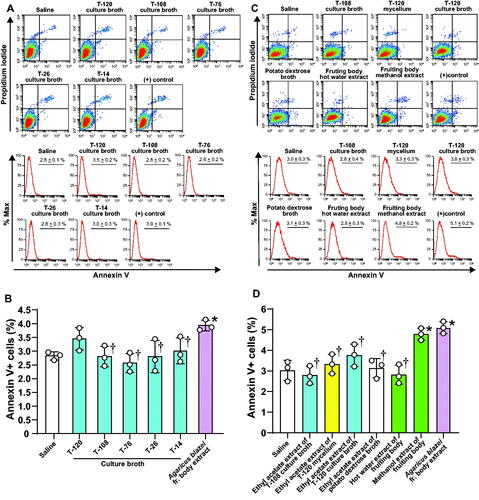
4. Discussion
Termitomyces sp. are rare mushrooms distributed only in specific geographical areas of Japan [Citation2,Citation9]. They grow in symbiosis with fungus-farming Termites [Citation3]. Recently, Termitomyces have been the focus of many studies for the medicinal properties of their structural components [Citation11]. However, the mycelia of Termitomyces sp. grew very slowly, making it difficult to collect a sufficient amount for commercial or therapeutic purposes [Citation37]. In the present study, we screened the mycelial growth of 45 strains of Termitomyces sp. and selected five showing marked mycelial growth in an artificial culture system. Sequence analysis demonstrated that the five selected strains correspond to species of Termitomyces. The sequence of 4 out of 5 selected strains (T-14, T-26, T-76, T-120) was highly identical to Termitomyces sp. YO198, with which they form a single cluster. The remaining strain, T-108, forms a cluster with the Termitomyces sp. strain BD4. Importantly, like most members of the Basidiomycota phylum, the five selected strains of Termitomyces showed the characteristic findings of dikaryon formation and clamp connection, indicating their capability to produce fruiting bodies. These observations suggest that the collected mushrooms were correctly identified as Termitomyces sp.
A novel piece of information the current study provides is the optimal conditions for the artificial cultivation of Termitomyces sp. Although solid media have generally been used for growing fruiting bodies of mushrooms under artificial culture conditions, the growth of fruiting bodies of Termitomyces in canonical solid media has been unsuccessful [Citation38]. Therefore, recent studies have focused on culturing the mycelium rather than the fruiting bodies of Termitomyces [Citation20,Citation39]. Successful cultivation of Termitomyces sp.’s mycelium on agar media has been documented [Citation20]. However, mycelial growth on agar media requires a long cultivation period and is poorly reproducible [Citation20]. To find out the most optimal culture condition, in the current study, we compared the growth rate of five selected strains of Termitomyces sp. between two liquid media (potato dextrose broth, mixed media) and between three solid substrates (sawdust, bark, peat moss). We found that four of the five selected strains developed more significant mycelial biomasses in potato dextrose broth than in mixed media and that media containing bark more consistently stimulated the mycelial growth of all selected strains than media containing sawdust or peat moss. These findings suggest that the artificial growth of Termitomyces may be substantially improved by cultivating in potato dextrose broth and bark.
Under natural conditions, Termitomyces have an obligated mutualistic relationship with Termites [Citation26]. In this symbiotic association, Termites provide a nest that the mushroom uses as a substrate to grow (fungus comb), and Termitomyces provide a lignocellulosic enzyme that digests the substrate consumed by Termites [Citation26]. Growth of Termitomyces in the nest of Termites is associated with high CO2 production [Citation40]. Therefore, fungus cultivation by Termites inside the fungus-comb chambers may lead to increased CO2 levels in the nest. The current study addressed the hypothesis that the CO2 level affects mushroom mycelial growth. The results of the experiments, however, showed that Termitomyces mycelium grows better under a normal atmospheric concentration of CO2. To explain this finding, we speculate that the architectural design and physical properties of the Termite nest probably maintain a stable and normal level of CO2 in the fungus-comb chambers [Citation41,Citation42]. Indeed, previous studies have shown that the fungus comb is effectively ventilated and that CO2 is dissipated to the surrounding atmosphere [Citation42]. Dissipation of the high CO2 level to the external atmosphere appears to occur by gas exchange through external openings and small pores in the nest’s outer wall [Citation26,Citation43–46]. Reports have shown that Termites can open and close these openings in response to changes in CO2 levels [Citation47]. Other reported mechanisms of Termite nest ventilation include temperature-driven convection current ventilation, external wind flow, and passive diffusion [Citation44–48]. Overall, these findings may explain the improved growth of Termitomyces under normal CO2 levels.
Clinical trials in humans, in vitro experiments, and pre-clinical studies in experimental animals have shown the beneficial effects of mushroom components in malignant tumors [Citation22,Citation23,Citation49]. The extracts of many mushrooms have been demonstrated to inhibit the proliferation and growth of malignant cells from the lungs, kidneys, liver, prostate, stomach, breast, and brain [Citation24,Citation25]. Dietary supplementation of the fungus Agaricus blazei was found to block tumor growth, and high mushroom consumption has been associated with a lower risk of cancer [Citation50,Citation51]. The concomitant administration of imidazole-4-carboxamide, a derivative from fairy-forming mushrooms, improved melanoma’s therapeutic response to an anticancer drug in an experimental animal model [Citation29]. The beneficial effects of products derived from Termitomyces for several benign and malignant diseases have been also well-documented [Citation11–19]. Recent studies suggested that inhibition of the expression of AXL receptor tyrosine kinase and the immune checkpoint molecules PD-L1 and PD-L2 by mushroom extracts may be a potential mechanism [Citation34,Citation52]. Cell activation by AXL, PD-L1, and PD-L2 inhibits the immune response [Citation34,Citation52]. Therefore, the increased expression of AXL, PD-L1, and PD-L2 is harnessed by cancer cells to evade the host immune response [Citation34,Citation52]. Inhibitors of AXL, PD-L1, and PD-L2 are also very effective drugs for treating patients with malignant tumors in clinical practice [Citation34]. In the present study, we evaluated whether extracts of Termitomyces sp. inhibit the expression of these molecules. This study showed that the culture broth supernatant and the mycelium’s methanol extract of the Termitomyces sp. strain T-120 significantly inhibit the expression of AXL, PD-L1, and PD-L2 in lung cancer and melanoma cell lines. In addition, the ethyl acetate extract of the strain T-120’s culture broth supernatant and the methanol extract of the fruiting body from Temitomyces sp. also showed inhibitory activity on the expression of AXL, PD-L1, and PD-L2. Overall, these observations suggest the potential usefulness of bioactive components of Temitomyces sp. as a co-adjuvant therapy in patients with cancer.
5. Conclusions
This study showed that PDB, bark media, and a normal CO2 level are the best conditions to obtain remarkable production of fungal mass for the artificial culture of Termitomyces sp., and suggest the potential of bioactive components of Temitomyces sp. as a co-adjuvant therapy in patients with cancer.
Author contributions
I.K.: conceptualization. E.C.G.: methodology. S.T., M.T., and T.K.: formal analysis. S.T., M.T., C.N.D-G., and T.Y.: resources. E.C.G., T.K., T.T., and I.K.: writing—original draft preparation. S.T., T.Y., and E.C.G.: writing—review and editing. E.C.G. and I.K.: funding acquisition. All authors have read and agreed to the published version of the manuscript.
Acknowledgments
The authors want to thank all people who cooperated in the mushroom collection.
Disclosure statement
E.C.G. and I.K. have a pending patent on the discovery reported in this article. Other authors declared no conflict of interest regarding this work.
Data availability statement
All data are available from the corresponding author under reasonable request.
Additional information
Funding
References
- Liu J, Wang Y, Wu J, et al. Isolation, structural properties, and bioactivities of polysaccharides from mushrooms Termitomyces: a review. J Agric Food Chem. 2022;70(1):21–33.
- Aanen DK, Eggleton P, Rouland-Lefevre C, et al. The evolution of fungus-growing termites and their mutualistic fungal symbionts. Proc Natl Acad Sci USA. 2002;99(23):14887–14892.
- Chouvenc T, Sobotnik J, Engel MS, et al. Termite evolution: mutualistic associations, key innovations, and the rise of termitidae. Cell Mol Life Sci. 2021;78(6):2749–2769.
- da Costa RR, Hu H, Li H, et al. Symbiotic plant biomass decomposition in fungus-growing termites. Insects. 2019;10(4):87.
- Froslev TG, Aanen DK, Laessoe T, et al. Phylogenetic relationships of Termitomyces and related taxa. Mycol Res. 2003;107(Pt 11):1277–1286.
- Ferreira IC, Barros L, Abreu RM. Antioxidants in wild mushrooms. Curr Med Chem. 2009;16(12):1543–1560.
- Ferreira IC, Vaz JA, Vasconcelos MH, et al. Compounds from wild mushrooms with antitumor potential. Anticancer Agents Med Chem. 2010;10(5):424–436.
- Nakalembe I, Kabasa JD, Olila D. Comparative nutrient composition of selected wild edible mushrooms from two agro-ecological zones, Uganda. Springerplus. 2015;4:433.
- Johjima T, Taprab Y, Noparatnaraporn N, et al. Large-scale identification of transcripts expressed in a symbiotic fungus (Termitomyces) during plant biomass degradation. Appl Microbiol Biotechnol. 2006;73(1):195–203.
- Taprab Y, Ohkuma M, Johjima T, et al. Molecular phylogeny of symbiotic basidiomycetes of fungus-growing termites in Thailand and their relationship with the host. Biosci Biotechnol Biochem. 2002;66(5):1159–1163.
- Hsieh HM, Ju YM. Medicinal components in Termitomyces mushrooms. Appl Microbiol Biotechnol. 2018;102(12):4987–4994.
- Woldegiorgis AZ, Abate D, Haki GD, et al. Antioxidant property of edible mushrooms collected from Ethiopia. Food Chem. 2014;157:30–36.
- Lu YY, Ao ZH, Lu ZM, et al. Analgesic and anti-inflammatory effects of the dry matter of culture broth of Termitomyces albuminosus and its extracts. J Ethnopharmacol. 2008;120(3):432–436.
- Mahamat O, Christopher T, Andre-Ledoux N, et al. Screening of the immunomodulatory and antibacterial activity of Termitomyces letestui (Pat.) Heim (Lyophyllaceae), an edible mushroom from Cameroon. J Basic Clin Physiol Pharmacol. 2018;29(6):645–650.
- Manna DK, Nandi AK, Pattanayak M, et al. A water soluble beta-glucan of an edible mushroom Termitomyces heimii: structural and biological investigation. Carbohydr Polym. 2015;134:375–384.
- Mau JL, Chao GR, Wu KT. Antioxidant properties of methanolic extracts from several ear mushrooms. J Agric Food Chem. 2001;49(11):5461–5467.
- Mau JL, Lin HC, Chen CC. Antioxidant properties of several medicinal mushrooms. J Agric Food Chem. 2002;50(21):6072–6077.
- Mondal A, Banerjee D, Majumder R, et al. Evaluation of in vitro antioxidant, anticancer and in vivo antitumour activity of Termitomyces clypeatus MTCC 5091. Pharm Biol. 2016;54(11):2536–2546.
- Nowakowski P, Markiewicz-Zukowska R, Bielecka J, et al. Treasures from the forest: evaluation of mushroom extracts as anti-cancer agents. Biomed Pharmacother. 2021;143:112106.
- Hsieh HM, Chung MC, Chen PY, et al. A termite symbiotic mushroom maximizing sexual activity at growing tips of vegetative hyphae. Bot Stud. 2017;58(1):39.
- Lu ZM, Tao WY, Zou XL, et al. Protective effects of mycelia of Antrodia camphorata and Armillariella tabescens in submerged culture against ethanol-induced hepatic toxicity in rats. J Ethnopharmacol. 2007;110(1):160–164.
- Anusiya G, Gowthama Prabu U, Yamini NV, et al. A review of the therapeutic and biological effects of edible and wild mushrooms. Bioengineered. 2021;12(2):11239–11268.
- Patel S, Goyal A. Recent developments in mushrooms as anti-cancer therapeutics: a review. 3 Biotech. 2012;2(1):1–15.
- Konno S, Chu K, Feuer N, et al. Potent anticancer effects of bioactive mushroom extracts (Phellinus linteus) on a variety of human cancer cells. J Clin Med Res. 2015;7(2):76–82.
- Shomali N, Onar O, Karaca B, et al. Antioxidant, anticancer, antimicrobial, and antibiofilm properties of the culinary-medicinal fairy ring mushroom, Marasmius oreades (Agaricomycetes). Int J Med Mushrooms. 2019;21(6):571–582.
- Aanen DK, Eggleton P. Fungus-growing termites originated in African rain forest. Curr Biol. 2005;15(9):851–855.
- Majumder R, Banik SP, Khowala S. AkP from mushroom Termitomyces clypeatus is a proteoglycan specific protease with apoptotic effect on HepG2. Int J Biol Macromol. 2016;91:198–207.
- Njue AW, Omolo JO, Cheplogoi PK, et al. Cytotoxic ergostane derivatives from the edible mushroom Termitomyces microcarpus (Lyophyllaceae). Biochem Syst Ecol. 2018;76:12–14.
- Inoue C, Yasuma T, D'Alessandro-Gabazza CN, et al. The fairy chemical imidazole-4-carboxamide inhibits the expression of Axl, PD-L1, and PD-L2 and improves response to cisplatin in melanoma. Cells. 2022;11(3):374.
- Malya IY, Wu J, Harada E, et al. Plant growth regulators and Axl and immune checkpoint inhibitors from the edible mushroom Leucopaxillus giganteus. Biosci Biotechnol Biochem. 2020;84(7):1332–1338.
- Ridwan AY, Wu J, Harada E, et al. Axl and immune checkpoints inhibitors from fruiting bodies of Pleurocybella porrigens. J Antibiot. 2020;73(10):733–736.
- Yasuma T, Toda M, Kobori H, et al. Subcritical water extracts from Agaricus blazei Murrill’s mycelium inhibit the expression of immune checkpoint molecules and Axl receptor. J Fungi. 2021;7:590.
- Hafizi S, Dahlback B. Gas6 and protein S. Vitamin K-dependent ligands for the Axl receptor tyrosine kinase subfamily. FEBS J. 2006;273(23):5231–5244.
- Waldman AD, Fritz JM, Lenardo MJ. A guide to cancer immunotherapy: from T cell basic science to clinical practice. Nat Rev Immunol. 2020;20(11):651–668.
- D'Alessandro-Gabazza CN, Kobayashi T, Yasuma T, et al. A Staphylococcus pro-apoptotic peptide induces acute exacerbation of pulmonary fibrosis. Nat Commun. 2020;11(1):1539.
- D'Alessandro-Gabazza CN, Yasuma T, Kobayashi T, et al. Inhibition of lung microbiota-derived proapoptotic peptides ameliorates acute exacerbation of pulmonary fibrosis. Nat Commun. 2022;13(1):1558.
- Wisselink M, Aanen DK, van ’t Padje A. The longevity of colonies of fungus-growing termites and the stability of the symbiosis. Insects. 2020;11(8):527.
- Abd Malek SN, Kanagasabapathy G, Sabaratnam V, et al. Lipid components of a Malaysian edible mushroom, Termitomyces heimii Natarajan. Int J Food Properties. 2012;15(4):809–814.
- Yang G, Ahmad F, Liang S, et al. Termitomyces heimii associated with fungus-growing termite produces volatile organic compounds (VOCs) and lignocellulose-degrading enzymes. Appl Biochem Biotechnol. 2020;192(4):1270–1283.
- Konate S, Le Roux X, Verdier B, et al. Effect of underground fungus-growing termites on carbon dioxide emission at the point- and landscape-scales in an African savanna. Insects. 2003;17:305–314.
- Kusumawardhani D, Nandika D, Karlinasari L, et al. Architectural and physical properties of fungus comb from subterranean termite Macrotermes gilvus (Isoptera: Termitidae) mound. Biodiversitas. 2021;22(4):1627–1634.
- Singh K, Muljadi BP, Raeini AQ, et al. The architectural design of smart ventilation and drainage systems in termite nests. Sci Adv. 2019;5(3):eaat8520.
- Cosarinsky MI. The nest growth of the neotropical mound-building termite, Cornitermes cumulans: a micromorphological analysis. J Insect Sci. 2011;11:122.
- Turner JS. Termites as models of swarm cognition. Swarm Intell. 2011;5(1):19–43.
- Korb J, Linsenmair KE. Ventilation of termite mounds: new results require a new model. Behav Ecol. 2000;11(5):486–494.
- Ocko SA, King H, Andreen D, et al. Solar-powered ventilation of African termite mounds. J Exp Biol. 2017;220(Pt 18):3260–3269.
- Turner JS. On the mound of Macrotermes michaelseni as an organ of respiratory gas exchange. Physiol Biochem Zool. 2001;74(6):798–822.
- King H, Ocko S, Mahadevan L. Termite mounds harness diurnal temperature oscillations for ventilation. Proc Natl Acad Sci USA. 2015;112(37):11589–11593.
- Gargano ML, van Griensven LJLD, Isikhuemhen OS, et al. Medicinal mushrooms: valuable biological resources of high exploitation potential. Plant Biosyst. 2017;151(3):548–565.
- Ba DM, Ssentongo P, Beelman RB, et al. Higher mushroom consumption is associated with lower risk of cancer: a systematic review and meta-analysis of observational studies. Adv Nutr. 2021;12(5):1691–1704.
- Delmanto RD, de Lima PL, Sugui MM, et al. Antimutagenic effect of Agaricus blazei Murrill mushroom on the genotoxicity induced by cyclophosphamide. Mutat Res. 2001;496(1–2):15–21.
- Davra V, Kimani SG, Calianese D, et al. Ligand activation of TAM family receptors-implications for tumor biology and therapeutic response. Cancers. 2016;8:107.