Abstract
This study examined the effects of myristate on an asymbiotic culture of Rhizoglomus intraradices, a species of arbuscular mycorrhizal fungi (AMF; Glomeromycota). Mycelial growth and sporulation in a modified medium containing myristate were observed. The findings demonstrated that myristate induced R. intraradices spore formation, with daughter spores having a smaller diameter than the parent spores. This observation is consistent with previous studies on other Rhizoglomus species. Further studies are needed to investigate the potential for continuous culture, mass production using daughter spores, and the application of AMF colonization techniques in plants.
1. Introduction
Arbuscular mycorrhizal fungi (AMF) have symbiotic relationships with terrestrial plants, where the fungi help host plants by increasing nutrient absorption, improving salt and drought resistance, and promoting growth through interactions with other microorganisms [Citation1]. AMF require the colonization of plant roots and the establishment of a symbiotic relationship during their life cycle. If hyphae do not encounter roots after the initial growth from spore germination, fungal growth ceases [Citation2]. As a result, AMF can be cultured either in pots with host plants or in Petri dishes with roots transformed by Rhizobium rhizogenes [Citation3,Citation4]. However, recent studies have revealed that AMF can be cultured alone without plants using a medium containing fatty acid [Citation5,Citation6]. Myristate induces sporulation during the asymbiotic growth of Rhizoglomus irregulare, and in the case of Rhizoglomus clarum, spore production increases when myristate and peptone are mixed. In this study, we investigated whether myristate induces sporulation in an asymbiotic culture of R. intraradices and examined the mycelial growth and sporulation processes.
2. Materials and methods
Soil collected from the fields was trap-cultured. A single spore was isolated and inoculated into sorghum (Sorghum bicolor (L.) Moench) to obtain a pure culture of R. intraradices (KNUE S1103) in pots. Pure-cultured soil from these pots was used in this study. After grinding 5 g of pure-cultured soil in a blender for 1 min, it was centrifuged at 2,000 rpm for 10 min with a 50% sugar solution. Spores in the supernatant were collected using a 45 µm sieve, and healthy spores were selected under a dissecting microscope.
DNA extracted from the crushed spores was amplified using nested PCR for the 18S ribosomal RNA (SSU) region. The first and second PCR used NS1 and NS4 primers and AML1 and AML2 primers, respectively [Citation7]. PCR products were sequenced by SolGent Co., Ltd. (Daejeon, Korea). Molecular identification was performed using BLAST, and phylogenetic trees were constructed with MEGA (version 7.0.26).
To surface-sterilize the spores, they were first treated with 2% chloramine T solution and gently vortexed for 15 min, followed by rinsing 2–3 times with sterile water. The spores were then treated with a 0.04% streptomycin solution and vortexed for 2 min, and rinsed again 2–3 times with sterile water. Ultimately, the sterilized spores were individually placed in a modified T medium [Citation6], in which potassium myristate was replaced with myristate (Samchun, Pyeongtaek, Korea). The spores were placed in the medium at 25 °C in the dark. Mycelial growth and spore formation were observed using an optical microscope (Axio Imager A1, Carl Zeiss, Oberkochen, Germany) and a dissecting microscope (Olympus SZX7, Tokyo, Japan). The runner hyphal length was measured using eXcope (version 3.7.7934) by the gridline intersection method [Citation8].
Ten weeks after cultivation, the mycelium and daughter spores were cut along with the medium block and subcultured under the same conditions. The parent and daughter spores were isolated from solid medium using 1 mM sodium hexametaphosphate [Citation9] and rinsed 2–3 times with sterile water. The spores were then stained with 100 μM SYTO 13 green fluorescent nucleic acid stain (Thermo Fisher Scientific, Massachusetts, USA) in water for 2 h, and observed by fluorescent microscopy (EVOS M5000, Thermo Fisher Scientific).
3. Results and discussion
The parent spores used in this study were identified as R. intraradices through morphological and molecular identification (). The small subunit sequence was 99.61% similar to R. intraradices FL208; additionally, in phylogenetic analysis based on the neighbor-joining method, the isolate KNUE S1103 was closely related to the same reported strain. The spores germinated within 3–6 days. From the germinated spores, a single transparent and thick hypha extended, and thin hyphae branched from it (). One to two hyphae branched minimally and continuously elongated, with a few reaching the end of the Petri dish. The hyphae around the parent spores branched more frequently than those extending farther away, with thin hyphae densely formed within a narrow area (). Daughter spores began to form around the parent spores 2–4 weeks later and were partially produced from the extended hyphae over time. The daughter spores were mostly spherical with elliptical shapes, with a diameter of (23.91–) 35.67 (–54.39) × (19.18–) 35.46 (–52.64) μm and exhibited a light-yellow color (). Daughter spore formation was observed only in the medium containing myristate. The diameters of the daughter spores were less than half those of the parent spores (). This finding was consistent with the characteristics of R. irregulare and R. clarum cultured in a medium containing myristic acid [Citation5,Citation6]. In the first cultivation and subculture, the daughter spores germinated, and the hyphae were thinner than the parent hyphae (). Following subculture, hyphae grew and branched more; in one of the subcultures, new daughter spores were observed (). However, the spores were produced on the parent hyphae, but not on the daughter hyphae.
Figure 1. Neighbor-joining phylogenetic tree of 18S small subunit regions of Rhizoglomus intraradices KNUE S1103. Glomus macrocarpum was used as an outgroup. Numbers of branches indicate bootstrap values (2000 replicates). The fungal isolate used in this study is shown in bold.
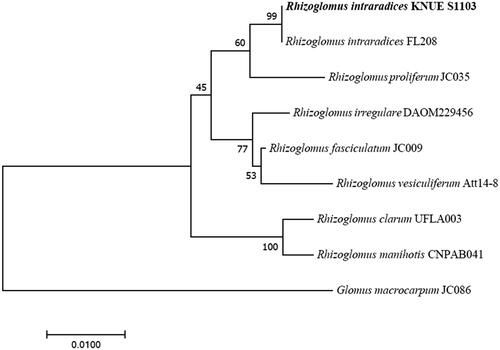
Figure 2. Asymbiotic culture of Rhizoglomus intraradices. (A) Parent spore stained with SYTO 13 green fluorescent nucleic acid stain and (B) daughter spore; (C,D) Germination of spores 3 days after culturing in myristate-containing medium. (E) Branching pattern of hyphae around the parent spore. (F) Branching pattern of hyphae extending far from the parent spore. (G) Appearance after 10 weeks of culturing in myristate-free medium. (H–J) Appearance after 10 weeks of culturing in myristate-containing medium (H–J) (scale bars: A,B: 75 µm, C–J: 100 µm; white arrow: parent spore, black arrow: daughter spore).
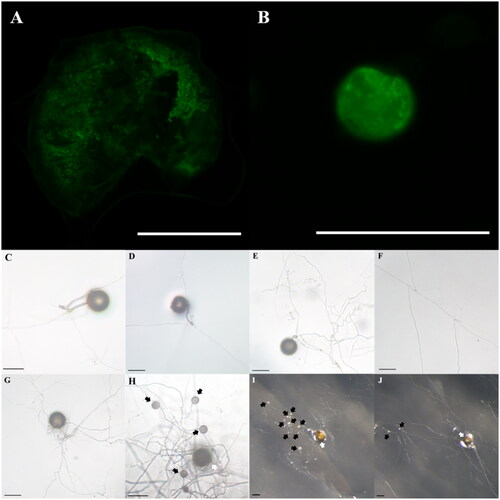
Figure 4. Daughter spore germination and new spore production at the subculture of Rhizoglomus intraradices. The germinated daughter spore (A,B); R. intraradices 10 weeks after cultivation (C) and 4 weeks after subculture (D) (scale bars: 100 µm; black arrow: hyphae from daughter spores, white arrow: daughter spores produced on first cultivation, red arrow: daughter spores produced on subculture).
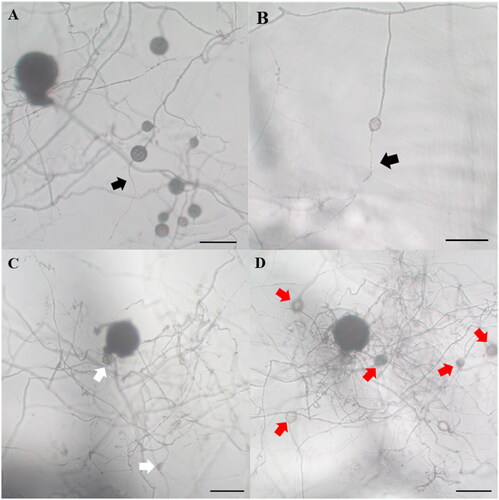
R. intraradices was reported to produce spores without plant roots when cocultured with the bacterium Paenibacillus validus [Citation10]. This study demonstrated that myristic acid is a factor in the asymbiotic spore production of R. intraradices. Based on the results of previous studies showing that the production of spores of R. irregulare and R. clarum can be induced using myristate [Citation5,Citation6], it is clear that spore production of Rhizoglomus species is induced by myristate. However, the finding that daughter spores have a significantly smaller diameter than the parent spores is consistent with that of a previous study. Considering palmitoleic acid accounts for the largest proportion of fatty acids in R. intraradices spore [Citation11], myristate is likely elongated and unsaturated after absorption. Given that, mitosis occurs during spore formation [Citation12], many fatty acids will be needed in the process. These factors may contribute to the formation of small daughter spores.
The possibility of continuous culture and mass production using daughter spores requires further investigation. Although daughter spores germinated, they formed thinner hyphae. Parent hyphae in subculture produced new daughter spores; however, daughter spores did not continue to the next generation. The maturity and diameter of spores affect their viability and germination [Citation12], and small daughter spores induced by myristate demonstrated low germination and growth rates. Considering that R. clarum can be continuously cultured in media containing myristate, peptone, strigolactone, and jasmonic acid [Citation6], it is thought that continuous cultivation of R. intraradices will be possible through the addition of phytohormones.
The findings imply that root organ and asymbiotic cultures rely on the formation of daughter spores; the potential use of AMF colonization techniques in plants is also worth exploring. Given that the success rate of inoculation and culture using single spores is low [Citation13,Citation14], using AMF grown for a certain period after germination in a medium containing myristate without host plants could potentially increase the success rate of colonization. By inoculating these AMF into plants along with sterilized soil, it may be possible to establish the colonization of a single species more effectively. This approach may also provide better control over the inoculation process and allow for easier monitoring of the progress of AMF colonization in the host plants. Further studies will be required to fully understand the advantages and limitations of this technique and to optimize the process for practical applications.
Disclosure statement
No potential competing interest was reported by the author(s).
References
- Anand K, Pandey GK, Kaur T, et al. Arbuscular mycorrhizal fungi as a potential biofertilizers for agricultural sustainability. J App Biol Biotech. 2022;10(1):90–107.
- Willis A, Rodrigues BF, Harris PJ. The ecology of arbuscular mycorrhizal fungi. Crit Rev Plant Sci. 2013;32(1):1–20.
- Mugnier J, Mosse B. Vesicular-arbuscular mycorrhizal infection in transformed root-inducing T-DNA roots grown axenically. Phytopathology. 1987;77(6):1045–1050.
- Declerck S, Strullu DG, Plenchette C. In vitro mass-production of the arbuscular mycorrhizal fungus, Glomus versiforme, associated with Ri T-DNA transformed carrot roots. Mycol Res. 1996;100(10):1237–1242.
- Sugiura Y, Akiyama R, Tanaka S, et al. Myristate can be used as a carbon and energy source for the asymbiotic growth of arbuscular mycorrhizal fungi. Proc Natl Acad Sci USA. 2020;117(41):25779–25788.
- Tanaka S, Hashimoto K, Kobayashi Y, et al. Asymbiotic mass production of the arbuscular mycorrhizal fungus Rhizophagus clarus. Commun Biol. 2022;5(1):1–9.
- Lee J, Lee S, Young JPW. Improved PCR primers for the detection and identification of arbuscular mycorrhizal fungi. FEMS Microbiol Ecol. 2008;65(2):339–349.
- Shen Q, Kirschbaum MU, Hedley MJ, et al. Testing an alternative method for estimating the length of fungal hyphae using photomicrography and image processing. PLoS One. 2016;11(6):e0157017.
- Doner LW, Bécard G. Solubilization of gellan gels by chelation of cations. Biotechnol Tech. 1991;5(1):25–28.
- Hildebrandt U, Ouziad F, Marner FJ, et al. The bacterium Paenibacillus validus stimulates growth of the arbuscular mycorrhizal fungus Glomus intraradices up to the formation of fertile spores. FEMS Microbiol Lett. 2006;254(2):258–267.
- Jabaji-Hare S. Lipid and fatty acid profiles of some vesicular-arbuscular mycorrhizal fungi: contribution to taxonomy. Mycologia. 1988;80(5):622–629.
- Marleau J, Dalpé Y, St-Arnaud M, et al. Spore development and nuclear inheritance in arbuscular mycorrhizal fungi. BMC Evol Biol. 2011;11(1):1–11.
- Selvakumar G, Krishnamoorthy R, Kim K, et al. Propagation technique of arbuscular mycorrhizal fungi isolated from coastal reclamation land. Eur J Soil Biol. 2016;74:39–44.
- Selvakumar G, Shagol CC, Kang Y, et al. Arbuscular mycorrhizal fungi spore propagation using single spore as starter inoculum and a plant host. J Appl Microbiol. 2018;124(6):1556–1565.