Abstract
To further explore the molecular mechanism of triterpenoid biosynthesis and acquire high-value strain of Sanghuangporus baumii, the Agrobacterium tumefaciens-mediated transformation (ATMT) system was studied. The key triterpenoid biosynthesis-associated gene isopentenyl diphosphate isomerase (IDI) was transformed into S. baumii by ATMT system. Then, the qRT-PCR technique was used to analyze gene transcript level, and the widely targeted metabolomics was used to investigate individual triterpenoid content. Total triterpenoid content and anti-oxidant activity were determined by spectrophotometer. In this study, we for the first time established an efficient ATMT system and transferred the IDI gene into S. baumii. Relative to the wild-type (WT) strain, the IDI-transformant (IT) strain showed significantly higher transcript levels of IDI and total triterpenoid content. We then investigated individual triterpenoids in S. baumii, which led to the identification of 10 distinct triterpenoids. The contents of individual triterpenoids produced by the IT2 strain were 1.76–10.03 times higher than those produced by the WT strain. The triterpenoid production showed a significant positive correlation with the IDI gene expression. Besides, IT2 strain showed better anti-oxidant activity. The findings provide valuable information about the biosynthetic pathway of triterpenoids and provide a strategy for cultivating high-value S. baumii strains.
1. Introduction
Various medicinal fungi have been shown to have important pharmacological effects. Sanghuangporus baumii (Pilát) L.W. Zhou & Y.C. Dai is a widely used traditional Chinese medicinal fungus with antitumor, anticancer, anti-inflammatory, antioxidant, immunomodulatory, and antigout properties [Citation1–4]. Many medicinal bioactive ingredients have been discovered in S. baumii, including triterpenoids, flavonoids, and polysaccharides [Citation5,Citation6]. It is worth mentioning that the triterpenoids from S. baumii and other medicinal fungi have been found to possess medicinal activities. For example, triterpenoids of S. baumii evidently display immune-enhancing and anticancer properties; furthermore, triterpenoid 4 exhibits potent antitumor promoting activity [Citation5]. The triterpenoid ganoderic acid (GA) was reported to inhibit the growth and metastasis of lung cancer and induce the apoptosis of cervical carcinoma HeLa cells [Citation7]. Anti-inflammatory, antioxidant, and anticancer activities have also been found from triterpenoids [Citation2,Citation8]. As triterpenoids are secondary metabolites, their yield in natural organisms is relatively low. Although numerous induction strategies have been applied to increase triterpenoid production, the yield of triterpenoids still remains insufficient for widespread medicinal use [Citation9–11].
Exploring triterpenoids using transgenic methods seems promising [Citation12]. Triterpenoids are synthesized via the mevalonate (MVA) pathway [Citation13,Citation14]. The initial substrate acetyl-CoA is catalyzed by key enzymes such as mevalonate pyrophosphate decarboxylase (MVD) and isopentenyl diphosphate isomerase (IDI) to generate dimethylallyl diphosphate (DMAPP), the common precursor for triterpenoid synthesis [Citation15]. DMAPP is then converted into various types of triterpenoids under the catalysis of key downstream enzymes such as squalene epoxidase (SE) and lanosterol synthase (LS; ). The content of DMAPP can determine the yield of downstream triterpenoid products. Thus, increasing the DMAPP content is important for ensuring high triterpenoid yield. DMAPP is synthesized from isopentenyl diphosphate (IPP) under the catalysis of IDI. Thus, the IDI gene is considered to be a key regulator in triterpenoid biosynthesis [Citation16]. At present, it is unclear if the overexpression of IDI gene can increase triterpenoid yield in S. baumii.
The emergence of genetic engineering has made it possible to determine the effect of IDI gene overexpression on triterpenoid yield. Gene overexpression technology can be used to regulate the metabolite biosynthetic pathway of key genes, making it feasible to improve triterpenoid yield. Further, Agrobacterium tumefaciens-mediated transformation (ATMT) has been applied to increase triterpenoid production in filamentous fungi [Citation14,Citation17]. When ATMT was used to overexpress squalene synthase (SQS) and LS genes in Ganoderma lucidum, the maximum triterpenoid contents of GA-MK and GA-O were 2.86 and 6.1 times higher than those of the wild strain, respectively [Citation13,Citation17]. Similarly, on transforming triterpenoid synthesis gene into Antrodia cinnamomea, the productions of the triterpenoids dehydrosulphurenic acid and dehydroeburicoic acid were found to increase by more than three times compared to the wild strain [Citation14]. Advances in ATMT have resulted in the development of increasingly powerful approaches for metabolic engineering that can be used to improve the triterpenoid yield in filamentous fungi. However, to our knowledge, ATMT has not been successfully applied in S. baumii.
Numerous studies have revealed the important pharmacological effects of triterpenoids. Some investigations have focused on the efficacy of various triterpenoids, which triterpenoids are mixtures of individual triterpenoids [Citation18–20]. In this case, it is difficult to determine which individual triterpenoids are responsible for the pharmacological effects, let al.one determine the biosynthetic pathways through which they are synthesized. Other studies have evaluated the functions of individual triterpenoids and their biosynthetic pathways. For example, triterpenoids including GA-S, GA-Mk, GA-Me, and GA-T were identified from G. lucidum, and their pharmacological activities were evaluated [Citation7,Citation21]. All studies reported on the pharmacological effects of triterpenoids in S. baumii have focused on total triterpenoids [Citation22,Citation23]. Considering that no studies have as yet explored individual triterpenoids, thus, it is important to investigate them in S. baumii.
In this study, we successfully constructed a genetic transformation system for S. baumii and transferred IDI gene into S. baumii. Transformants containing IDI gene were selected by PCR and Southern blot. Subsequently, the effects of IDI gene overexpression on the transcript levels of upstream and downstream genes and on total and individual triterpenoid production were investigated. We found that IDI gene overexpression significantly upregulated the transcript levels of downstream genes and increased the contents of individual as well as total triterpenoids. This study enhances our understanding of how the biosynthesis of individual triterpenoids is regulated and lays the foundation for developing more efficient S. baumii strains for triterpenoid production.
2. Materials and methods
2.1. Strain, vector, and culture conditions
S. baumii strain DL101 was stored in the China Center for Type Culture Collection (CCTCC No. M2011137). The ITS sequence was submitted to the NCBI GenBank (No. KP974834). Escherichia coli strain Top 10 and the A. tumefaciens strains were purchased from Tiangen Biology Company (Beijing, China). The vector pCAMBIA1301-gpd was preserved in the forest protection laboratory of Northeast Forestry University [Citation5]. It was modified by pCAMBIA1301, replacing the two 35S promoters in pCAMBIA1301 with the gpd promoters from S. baumii, and finally named pCAMBIA1301-gpd. The full sequence of the vector was provided in Supplementary Data. Pure cultures of S. baumii mycelia were cultivated on potato dextrose broth media or potato dextrose agar (PDA). Luria–Bertani (LB) broth or solid media were used for E. coli Top10 and A. tumefaciens cultures. The virulence of A. tumefaciens was preinduced using induction medium (IM). A. tumefaciens and S. baumii were cocultivated on cocultivation medium (Co-IM). Selection medium (SM) was used to screen positive transformants.
2.2. Construction of the IDI gene expression vector and transformation into S. baumii
IDI gene was amplified from S. baumii cDNA using the primers IDI-F1 and IDI-R1 (Supplementary Table S1). The amplified fragment was ligated into the vector pCAMBIA1301-gpd (linearized with Nco I) to create the pCAMBIA1301-gpd-IDI plasmid by using an In-Fusion HD Kit (Takara, Dalian, China). The pCAMBIA1301-gpd-IDI plasmid was transformed into A. tumefaciens EHA105 and pre-induced for 10 min using IM medium. A suspension of S. baumii mycelia was treated twice by dounce homogenizer, 0.2 g S. baumii broken mycelia were mixed with 1 mL preinduced A. tumefaciens solution for 20 min. A 200 µL portion of the mixture was poured onto 15 mL of Co-IM medium containing 0.2 mM acetosyringone (AS). After incubation at 25 °C, 5 mL of SM medium was covered on the mixture for 12 h. After 15 days of incubation at 25 °C, the visible S. baumii single colony was selected and transferred to fresh PDA medium containing 4 µg/mL hygromycin (Hyg). The colonies were subcultured five times to obtain stable S. baumii transformants. All media formulations and culture details are provided in the Supplementary Data.
2.3. Optimization of the ATMT system and detection
To obtain a highly efficient transformation system of S. baumii using the ATMT method, we tested different conditions for optimization. Different strains of A. tumefaciens (EHA105, AGL1, and LBA4404) were cocultured with S. baumii broken mycelia, and the most suitable strain (EHA105) was used. The mixtures were evenly spread on Co-IM medium containing various concentrations of AS (0, 0.05, 0.2, and 1 mM) and incubated at 25 °C for varying lengths of coculture duration (12, 24, 36, and 48 h). The rest of the transgenic methods and procedures were the same as those stated under 2.2. IDI gene and Hyg resistance cassette were verified by performing whole-genome PCR with the primers gpd-F2/Gus-R2 and Hyg-F2/Hyg-R2 (Supplementary Table S1). Southern blot was conducted to demonstrate foreign gene integration into S. baumii chromosome using the primers SNB-GusF2 and SNB-GusR2 (Table S1). Further details are provided in Supplementary Data.
2.4. Measurement of transcript levels by qRT-PCR
S. baumii mycelia were harvested and frozen at −80 °C. The total RNA of S. baumii was extracted using an RNAprep pure kit (Tiangen). Total RNA (1 µg) was reverse-transcribed using a PrimeScript RT reagent Kit with gDNA Eraser (Takara); synthesized cDNA was then used for quantitative real-time PCR (qRT-PCR). The transcript level of MVD, IDI, SE, and LS genes were evaluated by qRT-PCR, α-tubulin gene was used as the internal control gene. The primers for the amplification of five genes were provided in Supplementary Table S1. qRT-PCR was performed on a CFX 96 real-time PCR detection system (Bio-Rad, CA). The transcript level was normalized to the level of the internal control gene α-tubulin. The 2–ΔΔCt computing method was used to calculate the transcript levels of other genes [Citation24].
2.5. Quantification and purification of total triterpenoids
Mycelia were dried to constant weight at 45 °C, then 0.1 g dry cell weight (DW) of mycelia were used to extract total triterpenoids. Total triterpenoid content was calculated and sample purification according to the method reported by Liu et al. [Citation25].
2.6. Individual triterpenoid identification and quantification
Ultrahigh-performance liquid chromatography electrospray ionization tandem mass spectrometry (UHPLC-MS/MS) was used to determine the types and relative contents of individual triterpenoids in the IDI-transformed (IT) and the wild-type (WT) strains. The freeze-dried samples were crushed and extracted following the method of Maamoun et al. [Citation26]. The treated samples were stored at −80 °C until needed for UHPLC-MS/MS analysis.
UHPLC separation was carried out using an EXIONLC System (Sciex, Framingham, MA, USA) by Guangzhou Magigene Biotechnology Company (Guangzhou, China). Mobile phase A was 0.1% formic acid in water, while mobile phase B was acetonitrile. The column temperature was set at 40 °C. The autosampler temperature was 4 °C, and the injection volume was 2 µL. Sciex 6500 Q-TRAP LC/MS/MS system equipped with an Ion Drive Turbo V ESI ion source (Sciex Technologies) was applied for assay development. Quantitative analyses of metabolites were accomplished by multiple reaction monitoring. The typical ion source parameters were as follows: ion spray voltage = +5500/−4500 V; curtain gas pressure = 35 psi; temperature = 400 °C; ion source gas pressure = 1:60 psi; ion source gas pressure= 2:60 psi; and debunching voltage (DP) = ±100 V. Individual triterpenoids with variable importance in the projection > 1 and p < 0.05 were considered as significantly changed metabolites.
2.7. Analysis of antioxidant activity
The three classical antioxidant assays, ferric reducing antioxidant power (FRAP), O2– scavenging capacity assays, and DPPH radical scavenging capacity were carried out according to previous described [Citation27]. Total triterpenoid sample (0.1 g) was set as the test group, and 0.1 g BHT (butylated hydroxy toluene) was set as a positive control.
2.8. Statistical analysis
Data including qRT-PCR data, total and individual triterpenoid contents, antioxidant activity and biomass data were derived from three independent biological replicates. Error bars show standard deviation. Statistical significance and correlation were calculated with SPSS 17.0 using Duncan’s test and Spearman’s method, respectively. Differences were considered significant at p < 0.05.
3. Results
3.1. Construction of the S. baumii IT strain with overexpressed IDI gene
The IDI gene was constructed between the gpd promoter and Gus gene via homologous recombination to obtain the new plasmid pCAMBIA1301-gpd-IDI (). The primers gpd-F2/Gus-R2 and Hyg-F2/Hyg-R2 were used for PCR validation. The results showed amplicon bands that were consistent with theoretical results (supplemental Figure S1). Subsequently, IDI and Hyg genes were transferred into S. baumii mycelia using the ATMT system. Positive IT strains mediated by EHA105 still showed growth on SM after five generations of subculture, while the WT and IT strains mediated by AGL1 and LBA4404 did not grow on SM medium; subsequent transformation experiments were thus performed with EHA105.
Figure 2. Construction of overexpression vector and PCR validation of the transformants. (A) pCAMBIA1301-gpd-IDI vector. Gray arrows represent the terminators; the green square pattern represents Hyg gene; blue arrows represent the gpd promoters; purple square pattern represents IDI gene; orange square pattern represent Gus gene. Growth morphologies of the (B) IT1-3; (C) IT4-6; (D) IT7-8; (E) IT9-10; and WT strains cultured on SM medium (supplemented with 4 μg/mL Hyg); (F) Results of PCR amplification using the primers gpd-F2 and Gus-R2; (G) and Hyg-F2 and Hyg-R2. Lanes 1–10 correspond to IT1-10 strains; 11 to the WT strain; 12 to the negative control (H2O); 13 to the positive control (plasmid); and M to DNA marker 2000.
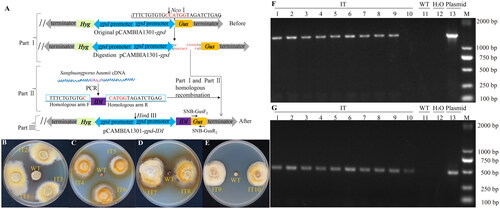
The length of coculture and concentration of AS also had a certain effect on the efficiency of ATMT. When AS concentration was 0.2 mM, three IT strains that continued to stably grow for 12 h and 24 h of cocultivation were encoded as IT1-3 () and IT4-6 (). Similarly, two IT strains that continued to stably grow for 36 h of cocultivation were encoded as IT7-8 (). However, no IT strains showed growth on prolonged cocultivation (>48 h). To ensure successful completion of the ATMT protocol, 12 h was chosen as the coculture duration for subsequent assays, whereas the optimal AS concentration was selected to be 0.2 mM (). Two IT strains that continued to stably grow in the presence of 0.05 mM AS were encoded as IT9-10 (), but no positive colonies were obtained in cocultures with either 0 mM or 1 mM AS. Based on these findings, cocultivation of EHA105 at 25 °C for 12 h in Co-IM containing 0.2 mM AS was determined to be the optimal conditions for high efficiency transformation in S. baumii.
Whole-genome PCR was then performed to detect the integrated IDI and Hyg genes of the transformants. The presence of integrated IDI and Hyg genes in the IT strain and positive control were confirmed using the primers gpd-F2/Gus-R2 () and Hyg-F2/Hyg-R2 (); no corresponding bands were found for the WT strain, false-positive strain (IT10), and negative control (). We obtained a total of 10 transgenic strains, of which IT10 was a false positive. We believe that IT10 contained only Hyg. To be precise, only nine stable transformants were obtained.
3.2. Effects of IDI gene overexpression on the transcript levels of upstream and downstream genes
The effects of IDI gene overexpression on the transcript levels of upstream and downstream genes in S. baumii were evaluated by qRT-PCR. The IDI transcript level was higher in the IT1-9 strains compared to that in the WT strain; the maximum transcript level was 9.29 times higher in the IT2 strain on day 9 (). The transcript level of the upstream MVD gene was not very significantly different between the IT and WT strains on day 9 and 12 (). However, the transcript levels of downstream genes were significantly higher in the IT1-9 strain than in the WT strain. The trends in the SE and LS genes over time were nearly the same, with the highest transcript levels observed on day 12, being 7.64 and 7.60 times higher than those in the WT strain, respectively (). These findings also proved that IT10 showed no exogenous IDI gene integration.
3.3. Overexpression of the IDI gene increased total triterpenoid production
To detect the effects of IDI gene overexpression on total triterpenoid contents and biomass production in S. baumii, samples were collected and analyzed after day 9 and 12 of cultivation. A spectrophotometer was used to measure absorption at 551 nm. In case of both strains, total triterpenoid content increased from day 9 to day 12 (), being significantly higher in the IT1-9 strain than in the WT strain on both days. Besides, total triterpenoid content of the IT1-9 strain on day 9 was even higher than that of the WT strain on day 12 (18.31 ± 0.36 mg/g). This further confirmed that IT10 had no exogenous IDI gene expression. The mycelia DW were not extremely significantly different between the IT and WT strains on the 9th and 12th days. The maximum DW for the IT5 and WT strains were 3.76 and 3.97 g/L, respectively ().
3.4. Effects of IDI gene overexpression on individual triterpenoid contents
The above results suggest that IT2 strain on day 12 shows high total triterpenoid productivity, 11.42 mg/g higher than WT strains, and IT2 strain has also been proved to have two copies of foreign gene (Supplementary Figure S2). However, individual triterpenoids that responded to IT strain are still unclear. Thus, the IT2 and WT strains on day 12 were selected to further investigate changes in the yields of individual triterpenoids. UHPLC-MS/MS analysis identified a total of 10 individual triterpenoids (Supplementary Table S2). Compared to the WT strain, the contents of all individual triterpenoids were higher in the IT2 strain. The contents of 11-keto-β-boswellic acid, dammarenediol II, friedelin, ganoderal A, germanicol, glycyrrhetic acid 3-O-mono-β-d-glucuronide, jangomolide, limonexic acid, rutaevin, and simiarenol in the IT2 strain were 6.00, 10.03, 5.98, 3.70, 5.60, 2.00, 1.82, 1.76, 3.14, and 3.73 times higher than those in the WT strain, respectively (). In addition, individual triterpenoids showed a significant positive correlation with the transcript levels of the IDI gene (), indicating that IDI gene plays a key role in triterpenoid biosynthesis.
Figure 5. Analysis of the relative contents of 10 individual triterpenoids in the WT and IT strains of Sanghuangporus baumii and their correlation with the IDI gene. (The mean difference being significant at the 0.05 level, p < 0.05). (A) 11-Keto-β-boswellic acid; (B) Dammarenediol II; (C) Friedelin; (D) Ganoderal A; (E) Germanicol; (F) Glycyrrhetic acid 3-O-mono-β-d-glucuronide; (G) Jangomolide; (H) Limonexic acid; (I) Rutaevin; (J) Simiarenol; (K) Spearman’s correlations analysis.
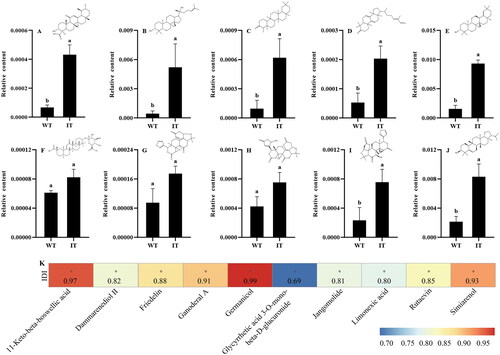
3.5. Analysis of the antioxidant capacities of triterpenoids between WT and IT strains
Oxidative damage is a negative effect produced by free radicals in vivo and is considered an important factor leading to aging and disease [Citation27]. To evaluate the antioxidant capacities of WT and IT strains, three classic antioxidant assays (FRAP, O2–, and DPPH) were carried out. Overall, all samples showed antioxidant capacity, and the antioxidant capacity of FRAP (), O2– (), and DPPH () of IT strain was significantly higher than that of WT strain, and even close to the antioxidant BHT, indicating that the triterpenoids of S. baumii have the potential to become natural antioxidants.
4. Discussion
ATMT has been widely used in the genetic transformation of various plant species because of its efficiency and technical simplicity [Citation28]. In later studies, ATMT was also found to be suitable for the genetic transformation of filamentous fungi [Citation14,Citation17,Citation29–31]. In this study, we successfully established an efficient transformation system for S. baumii using ATMT and obtained nine positive transgenic strains, demonstrating that ATMT is applicable to S. baumii. Herein, we found that stable genetic transformants were obtained only by EHA105-mediated transformation, and not by AGL1- or LBA4404-mediated transformation, indicating that the transformation efficiency of A. tumefaciens is affected by certain differences and preferences [Citation32,Citation33]. In addition to the influence of A. tumefaciens on genetic transformation efficiency, variations in cocultivation time and AS concentrations also led to differences in transformation efficiency [Citation34,Citation35]. These data suggest that appropriate conditions are pivotal for achieving good transformation efficiency and also for obtaining positive transformants.
In fungi, overexpression of the genes involved in triterpenoid biosynthesis can significantly increase the transcript levels of these genes themselves as well as of downstream genes and enhance triterpenoid production [Citation7,Citation13,Citation17]. Our results were consistent with those previously reported; overexpression of the IDI gene increased the transcript levels of not only IDI but also downstream genes, and also increased individual triterpenoid content and total triterpenoid production. The transcript level of the upstream MVD gene was not significantly different between the IT and WT strains on either day 9 or 12, which has been previously reported as well [Citation7,Citation13]. This suggests that the overexpression of downstream genes in the triterpenoid biosynthetic pathway does not affect the transcript levels of upstream genes. IDI gene overexpression increased the production of both total triterpenoid and individual triterpenoids, while it did not significantly affect cell growth. These findings concur with those of previous studies in which overexpression of the target gene had no extremely significantly affected on cell growth [Citation7,Citation36].
It is notable that the functions of the IDI gene have been mostly demonstrated in prokaryotes [Citation16,Citation37,Citation38], our results lay the foundation for IDI gene overexpression to increase triterpenoid production in eukaryotes. Seven individual triterpenoids were significantly increased by IDI gene overexpression in S. baumii, and these individual triterpenoids have obvious medicinal effects, including anti-inflammatory [Citation39], hypoglycemic [Citation40], anticancer [Citation41–43], antioxidant [Citation44], and cholesterol lowering [Citation45] effects, indicating that the strain producing more triterpenoids has higher medicinal value, this result has been confirmed in this study and previous reports [Citation2]. Further studies are warranted to explore how squalene 2,3-epoxide, a diverse substrate of triterpenoids, forms individual triterpenoids under the catalysis of oxidosqualene cyclases, which construct the basic triterpenoid skeletons; cytochrome P450 monooxygenases, which mediate oxidations; and uridine diphosphate-dependent glycosyltransferases, which catalyze glycosylations [Citation46].
Author contributions
Writing–original draft preparation, Zengcai Liu. Data curation, Ruipeng Liu. Writing–review and editing. Li Zou. All authors have read and agreed to the published version of the manuscript.
Supplemental Material
Download MS Word (244.1 KB)Disclosure statement
No potential conflict of interest was reported by the author(s).
Additional information
Funding
References
- Yang Y, He P, Li N. The antitumor potential of extract of the oak bracket medicinal mushroom Inonotus baumii in SMMC-7721 tumor cells. Evid Based Complement Alternat Med. 2019;2019:1242784.
- Cai CS, Ma JX, Han CR, et al. Extraction and antioxidant activity of total triterpenoids in the mycelium of a medicinal fungus, Sanghuangporus sanghuang. Sci Rep. 2019;9(1):7418.
- Liu MM, Zeng P, Li XT, et al. Antitumor and immunomodulation activities of polysaccharide from Phellinus baumii. Int J Biol Macromol. 2016;91:1199–1205.
- Yang K, Zhang S, Ying YM, et al. Cultivated fruit body of Phellinus baumii: a potentially sustainable antidiabetic resource. ACS Omega. 2020;5(15):8596–8604.
- Sun TT, Sun XY, Wang XT, et al. Construction of prokaryotic expression and overexpression vectors of squalene epoxidase gene from Sanghuangporus baumii. Chin Trad Herb Drugs. 2018;49:2632–2639.
- Li ZN, Li WJ, Wang JZ, et al. Defatted silkworm pupae hydrolysates as a nitrogen source to produce polysaccharides and flavonoids using Phellinus baumii. Biomass Conv. Bioref. 2021;11(2):527–537.
- Zhang DH, Jiang LX, Li N, et al. Overexpression of the squalene epoxidase gene alone and in combination with the 3-hydroxy-3-methylglutaryl coenzyme a gene increases ganoderic acid production in Ganoderma lingzhi. J Agric Food Chem. 2017;65(23):4683–4690.
- Lee CH, Hsu KH, Wang SY, et al. Cloning and characterization of the lanosterol 14alpha-demethylase gene from Antrodia cinnamomea. J Agric Food Chem. 2010;58(8):4800–4807.
- Sun TT, Zou L, Zhang LF, et al. Methyl jasmonate induces triterpenoid biosynthesis in Inonotus baumii. Biotechnol. Biotechnol. Equip. 2017;31(2):312–317.
- Xu YN, Xia XX, Zhong JJ. Induction of ganoderic acid biosynthesis by Mn2+ in static liquid cultivation of Ganoderma lucidum. Biotechnol Bioeng. 2014;111(11):2358–2365.
- Xu YN, Xia XX, Zhong JJ. Induced effect of Na+ on ganoderic acid biosynthesis in static liquid culture of Ganoderma lucidum via calcineurin signal transduction. Biotechnol Bioeng. 2013;110(7):1913–1923.
- Lu JMY, Fan WL, Wang WF, et al. Genomic and transcriptomic analyses of the medicinal fungus Antrodia cinnamomea for its metabolite biosynthesis and sexual development. Proc Natl Acad Sci USA. 2014;111(44):E4743–E4752.
- Zhou JS, Ji SL, Ren MF, et al. Enhanced accumulation of individual ganoderic acids in a submerged culture of Ganoderma lucidum by the overexpression of squalene synthase gene. Biochem. Eng. J. 2014;90:178–183.
- Lin YL, Lee YR, Tsao NW, et al. Characterization of the 2,3-Oxidosqualene cyclase gene from Antrodia cinnamomea and enhancement of cytotoxic triterpenoid compound production. J Nat Prod. 2015;78(7):1556–1562.
- Ajikumar PK, Xiao WH, Tyo KEJ, et al. Isoprenoid pathway optimization for taxol precursor overproduction in Escherichia coli. Science. 2010;330(6000):70–74.
- Wu FL, Shi L, Yao J, et al. The cloning, characterization, and functional analysis of a gene encoding an isopentenyl diphosphate isomerase involved in triterpene biosynthesis in the lingzhi or reishi medicinal mushroom Ganoderma lucidum (higher basidiomycetes). Int J Med Mushrooms. 2013;15(3):223–232.
- Zhang DH, Li N, Yu X, et al. Overexpression of the homologous lanosterol synthase gene in ganoderic acid biosynthesis in Ganoderma lingzhi. Phytochemistry. 2017;134:46–53.
- Wang YH, Wang GQ, Yi XM, et al. Hepatoprotective and antioxidant effects of total triterpenoids from Poria cocos. EJMP. 2017;21(2):1–9.
- Wang JR, Li YY, Liu DN. Cloning and characterization of farnesyl diphosphate synthase gene involved in triterpenoids biosynthesis from Poria cocos. Int J Mol Sci. 2014;15(12):22188–22202.
- Wu XD, Xie JZ, Qiu L, et al. The anti-inflammatory and analgesic activities of the ethyl acetate extract of Viburnum taitoense Hayata. J Ethnopharmacol. 2021;269:113742.
- Yang CS, Li WC, Li C, et al. Metabolism of ganoderic acids by a Ganoderma lucidum cytochrome P450 and the 3-keto sterol reductase ERG27 from yeast. Phytochemistry. 2018;155:83–92.
- Wang XT, Wang SX, Xu XR, et al. Molecular cloning, characterization, and heterologous expression of an acetyl-CoA acetyl transferase gene from Sanghuangporus baumii. Protein Expr Purif. 2020;170:105592.
- Huang J, Wang K, Zuo S, et al. Unsaturated fatty acid promotes the production of triterpenoids in submerged fermentation of Sanghuangporus baumii. Food Biosci. 2020;37:100712.
- Livak KJ, Schmittgen TD. Analysis of relative gene expression data using real-time quantitative PCR and the 2−ΔΔCT method. Methods. 2001;25(4):402–408.
- Liu ZC, Sun TT, Wang SX, et al. Cloning, molecular properties and differential expression analysis of the isopentenyl diphosphate isomerase gene in Sanghuangporus baumii. Biotechnol Biotechnol Equip. 2020;34(1):623–630.
- Maamoun AA, El-Akkad RH, Farag MA. Mapping metabolome changes in Luffa aegyptiaca Mill fruits at different maturation stages via MS-based metabolomics and chemometrics. J Adv Res. 2021;29:179–189.
- Lv H, Zhang Y, Shi J, et al. Phytochemical profiles and antioxidant activities of Chinese dark teas obtained by different processing technologies. Food Res Int. 2017;100(Pt 3):486–493.
- Vain P. Thirty years of plant transformation technology development. Plant Biotechnol J. 2007;5(2):221–229.
- Chen X, Stone M, Schlagnhaufer C, et al. A fruiting body tissue method for efficient Agrobacterium-mediated transformation of Agaricus bisporus. Appl Environ Microbiol. 2000;66(10):4510–4513.
- Michielse CB, Hooykaas PJJ, Hondel C, et al. Agrobacterium-mediated transformation of the filamentous fungus Aspergillus awamori. Nat Protoc. 2008;3(10):1671–1678.
- Shi L, Fang X, Li MJ, et al. Development of a simple and efficient transformation system for the basidiomycetous medicinal fungus Ganoderma lucidum. World J Microbiol Biotechnol. 2012;28(1):283–291.
- Wang S, Chen H, Wang Y, et al. Effects of Agrobacterium tumefaciens strain types on the Agrobacterium-mediated transformation efficiency of filamentous fungus Mortierella alpina. Lett Appl Microbiol. 2020;70(5):388–393.
- Shi L, Qin L, Xu YJ, et al. Molecular cloning, characterization, and function analysis of a mevalonate pyrophosphate decarboxylase gene from Ganoderma lucidum. Mol Biol Rep. 2012;39(5):6149–6159.
- Liu RD, Kim WY, Paguirigan JA, et al. Establishment of Agrobacterium tumefaciens-mediated transformation of Cladonia macilenta, a model lichen-forming fungus. JOF. 2021;7(4):252.
- Liu N, Chen GQ, Ning GA, et al. Agrobacterium tumefaciens-mediated transformation: an efficient tool for insertional mutagenesis and targeted gene disruption in Harpophora oryzae. Microbiol Res. 2016;182:40–48.
- Cardoza RE, Vizcaíno JA, Hermosa MR, et al. Cloning and characterization of the erg1 gene of Trichoderma harzianum: effect of the erg1 silencing on ergosterol biosynthesis and resistance to terbinafine. Fungal Genet Biol. 2006;43(3):164–178.
- Sun J, Zhang YY, Liu H, et al. A novel cytoplasmic isopentenyl diphosphate isomerase gene from tomato (Solanum lycopersicum): cloning, expression, and color complementation. Plant Mol Biol Rep. 2010;28(3):473–480.
- Wang YC, Qiu CX, Zhang F, et al. Molecular cloning, expression profiling and functional analyses of a cDNA encoding isopentenyl diphosphate isomerase from Gossypium barbadense. Biosci Rep. 2009;29(2):111–119.
- Miscioscia E, Shmalberg J, Scott KC. Measurement of 3-acetyl-11-keto-beta-boswellic acid and 11-keto-beta-boswellic acid in Boswellia serrata supplements administered to dogs. BMC Vet Res. 2019;15(1):1–7.
- Kim SH, Jung SH, Lee YJ, et al. Dammarenediol-II prevents VEGF-mediated microvascular permeability in diabetic mice. Phytother Res. 2015;29(12):1910–1916.
- Lu B, Liu L, Zhen X, et al. Anti tumor activity of triterpenoid rich extract from bamboo shavings Caulis bamfusae in Taeniam. Afr J Biotechnol. 2010;9:6430–6436.
- Supanimit T, Sasinun S, Chadarat A, et al. Pharmacokinetics of ganoderic acids a and f after oral administration of Ling Zhi preparation in healthy male volunteers. J. Evidence-Based Integr. Med. 2012;2012:780892.
- Jayaprakasha GK, Jadegoud Y, Nagana GGA, et al. Bioactive compounds from sour orange inhibit Colon cancer cell proliferation and induce cell cycle arrest. J Agric Food Chem. 2010;58(1):180–186.
- Poulose SM, Harris ED, Patil BS. Citrus limonoids induce apoptosis in human neuroblastoma cells and have radical scavenging activity. J Nutr. 2005;135(4):870–877.
- Hajjaj H, Mace C, Roberts M, et al. Effect of 26-oxygenosterolsfrom Ganoderma lucidum and their activity as cholesterol synthesis inhibitors. Appl Environ Microbiol. 2005;71(7):3653–3658.
- Sawai S, Saito K. Triterpenoid biosynthesis and engineering in plants. Front Plant Sci. 2011;2:25.