Abstract
Eurotiales is a relatively large order of Ascomycetes, well-known for their ability to produce secondary metabolites with potential beneficial applications. To understand their diversity and distribution, different environmental sources including soil, freshwater, insect, and indoor air were investigated. Eight strains of Eurotiales were isolated and identified based on their morphological characters and a multi-gene phylogenetic analysis of the ITS, BenA, CaM, and RPB2 regions. We identified eight taxa that were previously not reported from Korea: Aspergillus baeticus, A. griseoaurantiacus, A. spinulosporus, Penicillium anthracinoglaciei, P. labradorum, P. nalgiovense, Talaromyces atroroseus, and T. georgiensis. Detailed descriptions, illustrations, and phylogenetic tree for the eight new records species are presented, and information regarding the records is also discussed.
1. Introduction
Eurotiales is a relatively large order of Ascomycetes, which has both positive and negative impacts on human activities. The positive aspects include their utilization in food fermentation and biotechnology for the production of enzymes, organic acids, and medications [Citation1–4]. The negative aspects include opportunistic infections, indoor growth, food spoilage, and mycotoxin production [Citation1,Citation5,Citation6].
Eurotiales comprises 28 genera; 15 of them are classified under the Aspergillaceae family (Aspergillago, Aspergillus, Evansstolkia, Hamigera, Leiothecium, Monascus, Penicilliopsis, Penicillium, Phialomyces, Pseudohamigera, Pseudopenicillium, Sclerocleista, Warcupiella, Xerochrysium, and Xeromyces), two under Elaphomycetaceae (Elaphomyces and Pseudotulostoma), eight under Trichocomaceae (Acidotalaromyces, Ascospirella, Dendrosphaera, Rasamsonia, Sagenomella, Talaromyces, Thermomyces, Trichocoma), two under Thermoascaceae (Paecilomyces, Thermoascus), and one under Penicillaginaceae (Penicillago) [Citation1].
The genera Aspergillus, Penicillium, and Talaromyces (Eurotiomycetes, Eurotiales) are considered to be among the most chemically inventive fungi, producing a wide array of secondary metabolites [Citation1,Citation2,Citation7–9]. They are known for their ability to produce enzymes, organic acids, and antibacterial, anticancer, antifungal, antioxidative, and antiproliferative compounds [Citation1,Citation7,Citation8,Citation10–15]. In addition, they are effective biocontrol agents [Citation16].
The genus Aspergillus was first described by Micheli [Citation17]. Members of this genus are usually found in soil, water, decaying vegetation, seeds, grains, and indoor air environment [Citation18–20]. Currently, the genus Aspergillus is divided into six subgenera, 27 sections, 75 series, and 446 species [Citation1].
The genus Penicillium was erected by Link [Citation21]. Members of the genus are isolated from diverse substrates, including soil, water, air, indoor environments, and food products [Citation22–24]. Currently, this genus is divided into two subgenera, 32 sections, 89 series, and 483 accepted species [Citation1].
The genus Talaromyces was initially described by Benjamin as a sexual state of the genus Penicillium [Citation25]. Talaromyces species are distributed worldwide and are commonly isolated from soil, water, indoor environment, and human [Citation8,Citation26–29]. Currently, the genus Talaromyces is divided into eight sections and 171 accepted species [Citation1,Citation8,Citation27].
Although Aspergillus, Penicillium, and Talaromyces species are of economic importance, the current number of the three genera found in Korea is still limited [Citation23,Citation28–31]. These genera are relevant and significant in human life, thus it is important to isolate and identify them.
This work presents eight new records of Aspergillus baeticus, A. griseoaurantiacus, A. spinulosporus, Penicillium anthracinoglaciei, P. labradorum, P. nalgiovense, Talaromyces atroroseus, and T. georgiensis in Korea based on both morphological characteristics and phylogenetic analyses.
2. Materials and methods
2.1. Sample collection, and isolation
Soil samples were collected from Kunryang-ri, Cheongyang, Chungnam Province, Samgak-dong, Buk-gu, Gwangju, and Sangju, Gyeongsang Province in South Korea. Freshwater and insects samples were collected from Hanbat Arboretum located in Daejeon, and Kunryang-ri, Cheongyang, Chungnam Province. The samples were kept at 4 °C until further use. Fungal isolations from soil, freshwater, and insect were carried out as previously detailed [Citation28, Citation32]. Strains were isolated using potato dextrose agar (PDA; Becton, Dickinson and Co., Sparks, MD) and malt extract agar (MEA; Becton, Dickinson and Co., Sparks, MD) amended with 50 ppm of the antibiotic neomycin. For indoor air, colonies were picked from the contaminated plates kept at 25 °C. Individual colonies with various morphologies were picked and transferred to new PDA plates. All the pure isolates were maintained in PDA slant tubes and 20% glycerol at −80 °C at the Environmental Microbiology Laboratory Fungarium, Chonnam National University, Gwangju, South Korea. The strains were also deposited at the Culture Collection of the Nakdonggang National Institute of Biological Resources (NNIBR), Sangju, South Korea under the numbers NNIBRFG46707 and NNIBRFG46718, and the Collection of the National Institute of Biological Resources (NIBR), Incheon, Korea under the numbers NIBRFGC000508631, NIBRFG0000509598, NIBRFG0000509600, EKFTFGC000000138, and EKFTFGC000000142, and YYMAFGC000000144.
2.2. DNA extraction, PCR, and sequencing
Genomic DNA extractions were performed on seven-day-old colonies grown on PDA using the Solg™ Genomic DNA Prep Kit (Solgent Co. Ltd., Daejeon, South Korea). The internal transcribed spacer (ITS) rDNA, β-tubulin (BenA), calmodulin (CaM), and RNA polymerase II second largest subunit (RPB2) gene regions were amplified by PCR using the primer pairs ITS5/ITS4 [33] and V9G/ITS4 [Citation33,Citation34], Tub2Fd/Tub4Rd [Citation35] and T1/T22 [Citation36], CF1L/CF4 [Citation36], Cmd5/Cmd6 [Citation37], and RPB2-5F/RPB2-7cR [Citation38], respectively. PCR was done in 20 μL volumes with AccuPower PCR PreMix (BioneerCorp., Daejeon, South Korea), 2 μL genomic DNA, 1.5 μL of each primer (5 pmol/μL), and 14 μL deionized water. The PCR thermal cycle programs for ITS, BenA, CaM, and RPB2 amplification were as follows: initial denaturing step of 95 °C for 5 min, followed by 35 cycles of denaturation at 95 °C for 30 s, annealing at 52 °C (ITS) or 55 °C (BenA, CaM, RPB2) for 30 s, elongation at 72 °C for 45 s, and final extension at 72 °C for 7 min. The PCR products were then purified using an Accuprep PCR Purification Kit (BioneerCorp., Daejeon, South Korea). The purified PCR products were sequenced in both directions with the same primers by Macrogen (Daejeon, South Korea).
2.3. Molecular analyses
The taxa used in the phylogenetic analysis were obtained from previous studies [Citation1, Citation28, Citation39,Citation40], and downloaded from GenBank (www.ncbi.nlm.nih.gov/genbank/). SeqMan v. 7.0.0 (DNAstar, Madison, WI) was used to assemble the consensus sequences. The sequences were aligned using the MAFFT v.7 online program (http://mafft.cbrc.jp/alignment/server/) [Citation41] and manually optimized using MEGA v.7 [Citation42]. Maximum-likelihood (ML) analysis was performed using the RAxML-HPC2 on XSEDE (v.8.2.12) in the CIPRES Science Gateway (https://www.phylo.org/portal2) using a GTRGAMMA model with rapid bootstrap analysis followed by 1000 bootstrap replicates. The consensus trees were viewed in FigTree v. 1.3.1 [Citation43]. The newly generated sequences in this study were deposited in GenBank ().
Table 1. Fungal species and sequences used in phylogenetic analyses.
2.4. Morphological studies
Methods used for morphological observations followed previous study [Citation8, Citation44–46]. Colony characteristics were recorded after seven days of incubation. Micro-morphological characters were observed under a light microscope using a differential interference contrast microscope (Olympus BX53, Tokyo, Japan) and Olympus DP74 digital camera.
3. Results
3.1. Phylogenetic analyses
The phylogenetic relationship of isolates CNUFC SF23, CNUFC CY2264, and CNUFC GRS15 with accepted Aspergillus species was determined by analysis of concatenated sequence datasets of four loci (ITS, BenA, CaM, and RPB2). The concatenated alignment consisted of 2748 nucleotides, including inserted gaps (ITS: 675 bp, BenA: 459 bp, CaM: 603 bp, and RPB2: 1011 bp).
The phylogenetic relationship of isolates CNUFC CY2234, CNUFC MOP1, and CNUFC CY224 with accepted Penicillium species was determined by analysis of concatenated sequence datasets of four loci (ITS, BenA, CaM, and RPB2). The concatenated alignment consisted of 2619 nucleotides, including inserted gaps (ITS: 584 bp, BenA: 499 bp, CaM: 581 bp, and RPB2: 955 bp).
The phylogenetic relationship of isolates CNUFC SJ322 and CNUFC DW211 with accepted Talaromyces species was determined by analysis of concatenated sequence datasets of four loci (ITS, BenA, CaM, and RPB2). The concatenated alignment consisted of 2806 nucleotides, including inserted gaps (ITS: 653 bp, BenA: 573 bp, CaM: 694 bp, and RPB2: 886 bp).
Isolates CNUFC GRS15, CNUFC SF23, and CNUFC CY2264 were clustered with the ex-type strain of A. baeticus, A. griseoaurantiacus, and A. spinulosporus, respectively, with strong statistical support (). In , isolates CNUFC CY2234, CNUFC MOP1, and CNUFC CY224 were clustered with the ex-type strain of P. anthracinoglaciei, P. labradorum, and P. nalgiovense with 100% ML support, respectively. Isolates CNUFC SJ322 and CNUFC DW211 were grouped with the ex-type strain of T. atroroseus, and T. georgiensis, respectively, with strong statistical support ().
Figure 1. Maximum-likelihood (RAxML) analysis based on combined ITS, BenA, CaM, and RPB2 sequence data showing the relationship of the isolates CNUFC SF23, CNUFC CY2264, and CNUFC GRS15 with related species in sections Aenei, Nidulantes, and Usti of the genus Aspergillus. The numbers above or below the branches represent maximum-likelihood bootstrap percentages. Bootstrap values equal to or greater than 70% are indicated above or below the branches. Aspergillus ochraceoroseus NRRL 28622 was used as the outgroup. The newly generated sequence is indicated in blue.
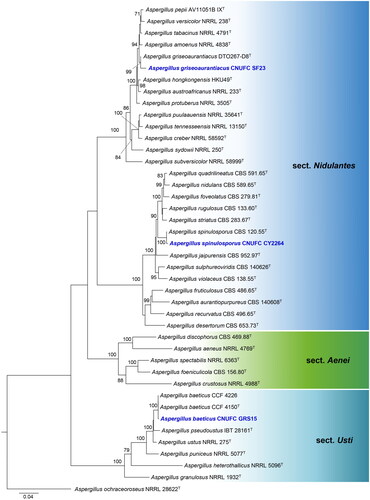
Figure 2. Maximum-likelihood (RAxML) analysis based on combined ITS, BenA, CaM, and RPB2 sequence data showing the relationship of the isolates CNUFC CY2234, CNUFC MOP1, and CNUFC CY224 with related species in sections Brevicompacta, Canescentia, Chrysogena, Exilicaulis, and Robsamsonia of the genus Penicillium. The numbers above or below the branches represent maximum-likelihood bootstrap percentages. Bootstrap values equal to or greater than 70% are indicated above or below the branches. Aspergillus glaucus NRRL 116 was used as the outgroup. The newly generated sequence is indicated in blue.
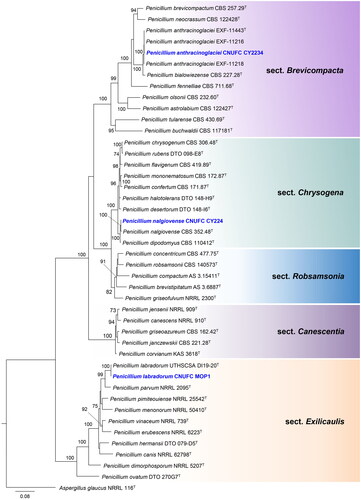
Figure 3. Maximum-likelihood (RAxML) analysis based on combined ITS, BenA, CaM, and RPB2 sequence data showing the relationship of the isolates CNUFC SJ322, and CNUFC DW211 with species in sections Helici, Purpurei, Talaromyces, Tenues, and Trachyspermi of the genus Talaromyces. The numbers above or below the branches represent maximum-likelihood bootstrap percentages. Bootstrap values equal to or greater than 70% are indicated above or below the branches. Trichocoma paradoxa CBS 103.73 was used as the outgroup. The newly generated sequence is indicated in blue.
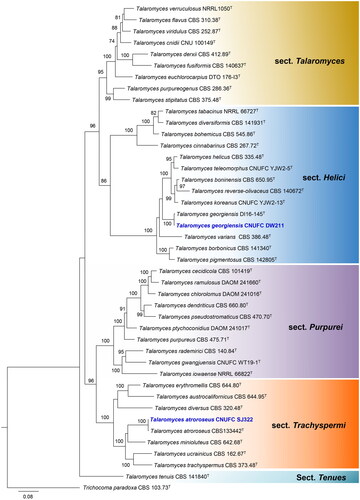
3.2. Taxonomy
Aspergillus baeticus A. Novakova & Hubka, Int. J. Syst. Evol. Microbiol. 62 (Pt.2): 2783 (2012) [MB#564188] ():
Figure 4. Morphology of Aspergillus baeticus CNUFC GRS15. (A, D) Colonies on Czapek yeast autolysate agar (CYA); (B, E) malt extract agar (MEA); (C, F) yeast extract sucrose agar (YES) (A–C: obverse view and D–F: reverse view); (G–I) Conidiophores; (J) Conidia. Scale bars = 10 µm.
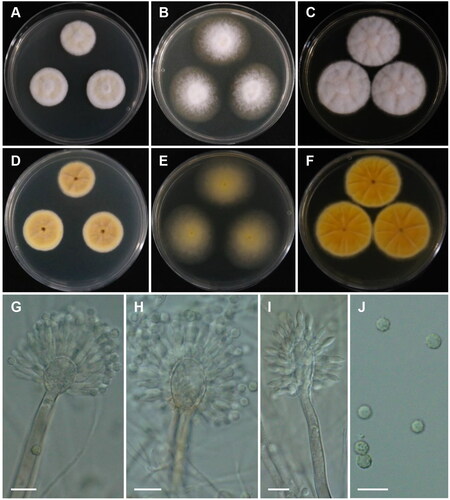
Description: On CYA at 25 °C, colonies slightly sulcate; mycelium white; texture floccose at center; sporulation sparse to moderate; no pigment or exudate produced; reverse yellow-brown at center to grayish yellow at margin, and reached 23–25 mm in diameter after seven days. On MEA at 25 °C, colonies raised at center, texture floccose; sporulation strong; no pigment or exudate produced; reverse moderate yellow, and reached 36–40 mm in diameter after seven days. On YES at 25 °C, colonies slightly raised at center; radially sulcate; texture velutinous to slightly floccose; sporulation sparse to moderate; reverse vivid yellow and reached 34–37 mm in diameter after seven days.
Micromorphology: Stipes smooth walled, 105.5–491(–601.5) × 5–7.5 μm. Vesicles broadly elliptical or elongated, (9.5–)11–18 × 13–20.5(–23.5) μm. Metulae 5–6.5 × (2.5–)3–4 μm. Phialides 6.5–8.5(–9) × 2–3.5 μm. Conidia globose to subglobose, finely roughened, (2.5–)3–4.5 μm.
Material examined: Republic of Korea, Samgak-dong, Buk-gu, Gwangju (35°11’58.1”N 126°53’58.1”E), from soil, October 12, 2019 (culture CNUFC GRS15).
Notes: Our strain did not produce Hülle cells on CYA and grew slower than that of A. baeticus (ex-type strain) on CYA at 25 °C (23–25 mm vs. 35–38 mm) [Citation47].
Aspergillus griseoaurantiacus Visagie, Hirooka & Samson, Studies in Mycology 78: 112 (2014) [MB#809197] ():
Figure 5. Morphology of Aspergillus griseoaurantiacus CNUFC SF23. (A, D) Colonies on Czapek yeast autolysate agar (CYA); (B, E) malt extract agar (MEA); (C, F) yeast extract sucrose agar (YES) (A–C: obverse view and D–F: reverse view); (G–I) Conidiophores; (J) Conidia. Scale bars = 10 µm.
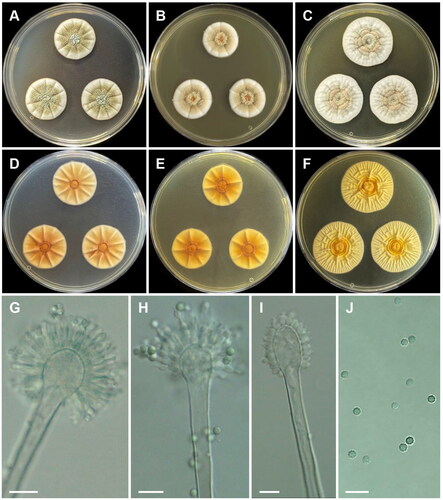
Description: On CYA at 25 °C, colonies radially sulcate; mycelium white; colony texture cottony at center; sporulation moderate; reverse moderate yellow and reached 26–28 mm in diameter after seven days. On MEA at 25 °C, colonies raised at center; radially sulcate; margins entire; texture velvety; sporulation moderate; reverse vivid yellow and reached 25–27 mm in diameter after seven days. On YES at 25 °C, colonies radially sulcate; mycelium white; colony texture floccose; sporulation strong at center and sparse at margins; reverse pale yellow and reached 32–38 mm in diameter after seven days.
Micromorphology: Stipes smooth walled, 241–449.5 × 4.5–6 μm. Vesicles spathulate or elongated, 8.5–17.5 μm wide. Metulae 3–5.5(–7) × 2.5–4(–5) μm. Phialides ampulliform, 3 per metula, 4–7.5(–8.5) × 2–3 μm. Conidia globose to subglobose, some ellipsoidal, finely roughened, 2.5–3.5 × 2–2.5 μm.
Material examined: Republic of Korea, 54–30 Mukdong-gil, Cheongyang-eup, Cheongyang-gun, Chungcheongnam-do (33°14’52.7”N 126°24’48.3”E), from soil, February 2021, H.B. Lee (culture CNUFC SF23).
Notes: The size of metulae of our strain was slightly shorter than that of the ex-type of A. griseoaurantiacus (3–5.5 [–7] × 2.5–4 [–5] μm vs. 4–10 × 3–5.5 µm) [Citation48]. In addition, the ex-type of A. griseoaurantiacus did not produce the globose and subglobose conidia observed in our strain [Citation48].
Aspergillus spinulosporus Hubka, S.W. Peterson, M. Kolařík, Plant Systematics and Evolution 302 (9): 1290 (2016) ():
Figure 6. Morphology of Aspergillus spinulosporus CNUFC CY2264. (A, D) Colonies on Czapek yeast autolysate agar (CYA); (B, E) malt extract agar (MEA); (C, F) yeast extract sucrose agar (YES) (A–C: obverse view and D–F: reverse view); (G, H) Conidiophores; (I) Conidia; (J) Hülle cells. Scale bars: G–I = 10 µm, J = 20 µm.
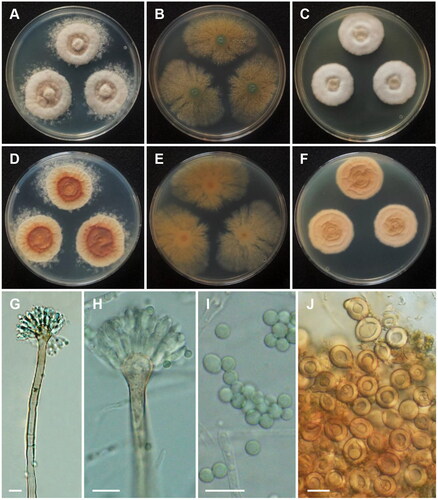
Description: On CYA at 25 °C, colonies raised at center; mycelium white; sporulation moderate; reverse deep orange at center and moderate orange yellow at margins and reached 29–33 mm in diameter after seven days. On MEA at 25 °C, colonies plane; poor sporulation; Hülle cells abundant; reverse pale yellow and reached 43–48 mm in diameter after seven days. On YES at 25 °C, colonies slightly raised at center; radially sulcate; mycelium white; colony texture floccose; sporulation absent; reverse light to moderate orange yellow and reached 25–30 mm in diameter after seven days.
Micromorphology: Hülle cells globose, subglobose to ovoid, 15–21.5 μm wide. Conidiophores with smooth stipes, hyaline to light brown, 181–383 × 5.5–8.5 μm. Vesicles subclavate, 10.5–16 μm wide. Metulae hyaline, 7.5–10 × 3–4.5 μm. Phialides hyaline, flask-shaped, 6.5–10 × 3–4 μm. Conidia globose, some oval, smooth, 3–4 μm.
Material examined: Republic of Korea, Kunryang-ri, Cheongyang-eup, Cheongyang, Chungnam Province (36°26’16.2”N 126°46’04.6”E), from soil, February 25, 2022, H.B. Lee (culture CNUFC CY2264).
Notes: There were differences in the size of conidiophore and Hülle cell when compared with the ex-type strain of A. spinulosporus described in Chen et al. [Citation49].
Penicillium anthracinoglaciei L. Perini, Frisvad & Zalar, Microbial Ecology 86, 282–296 (2022) [MB#835602] ():
Figure 7. Morphology of Penicillium anthracinoglaciei CNUFC CY2234. (A, D) Colonies on Czapek yeast autolysate agar (CYA); (B, E) malt extract agar (MEA); (C, F) yeast extract sucrose agar (YES) (A–C: obverse view and D–F: reverse view); (G, H) Conidiophores; (I) Conidia. Scale bars= 20 µm.
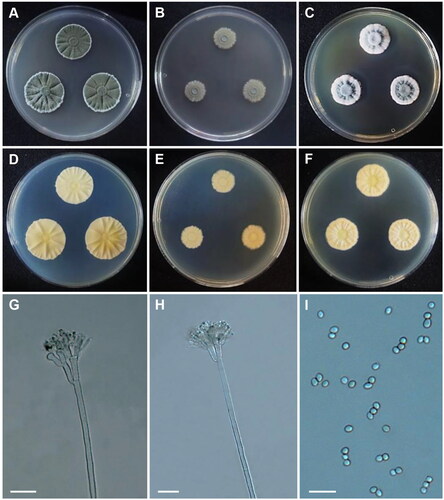
Description: On CYA at 25 °C, colonies radially sulcate; mycelium white; colony texture velvety; sporulation strong; reverse moderate yellow and reached 28–30 mm in diameter after seven days. On MEA at 25 °C, mycelium white and green; texture velvety; sporulation moderate; reverse pale yellow and reached 16–18 mm in diameter after seven days. On YES at 25 °C, colonies raised at center, radially sulcate; mycelium white sometimes green; colony texture floccose; sporulation absent at center and sparse at margins; reverse pale yellow and reached 20–24 mm in diameter after seven days.
Micromorphology: Conidiophores terverticillate. Stipes 85–215 × 3–4.5 μm. Metulae 6–11 × 3–4.5 μm. Phialides 5–13 × 2–4.5 μm. Conidia subglobose to ellipsoidal, 2.5–4 × 2–3.5 μm.
Material examined: Republic of Korea, Kunryang-ri, Cheongyang-eup, Cheongyang, Chungnam Province (36°26’16.2”N 126°46’04.6”E), from dead bee in rainwater, July 2022, H.B. Lee (culture CNUFC CY2234).
Notes: Compared with the ex-type strain of P. anthracinoglaciei, our strain grew faster on CYA (28–30 mm vs. 13–20 mm) [Citation50].
Penicillium labradorum Gibas, Wiederh, C. Sanders, Rothacker, E.R. Rogers & Fales, Medical Mycology, 58(8): 1061 (2020) ():
Figure 8. Morphology of Penicillium labradorum CNUFC MOP1. (A, D) Colonies on Czapek yeast autolysate agar (CYA); (B, E) malt extract agar (MEA); (C, F) yeast extract sucrose agar (YES) (A–C: obverse view and D–F: reverse view); (G, H) Conidiophores; (I) Conidia. Scale bars = 10 µm.
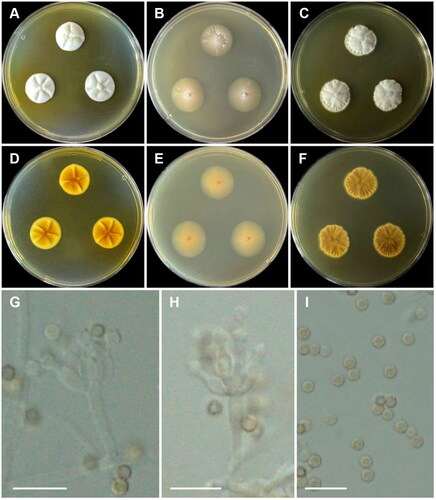
Description: On CYA at 25 °C, colonies wrinkled; mycelia white; sporulation poor; soluble pigments absent, exudates vivid yellow droplets; reverse moderate to strong yellow and reached 19–22 mm in diameter after seven days. On MEA at 25 °C, colonies plane; mycelia white, sporulation absent; reverse pale yellow and reached 17–22 mm in diameter after seven days. On YES at 25 °C, colonies sulcate; texture floccose, slightly raised at center, sporulation absent; reverse light to moderate yellowish brown and reached 13–21 mm in diameter after seven days.
Micromorphology: Conidiophores monoverticillate. Stipes 5.5–17.5 μm. Phialides smooth, ampulliform, 2–4 per stipe, 5–6.5(–7) × 2–3 μm. Conidia globose to subglobose, finely roughened, 2–2.5 μm.
Material examined: Republic of Korea, Gwangju, Buk-gu, Chonnam National University (35°10’34.0”N 126°54’21.0”E), from an indoor air sample, April 9, 2020 (culture CNUFC MOP1).
Notes: Compared with the ex-type strain of P. labradorum, there was a slight difference. Our strain grew faster on CYA (19–22 mm vs. 10–11 mm) and MEA (17–22 mm vs. 16–17 mm) [Citation39].
Penicillium nalgiovense Laxa, Zentralbl. Bakteriol. 2. Abt. 86 (5–7): 160 (1932) [MB#114239] ():
Figure 9. Morphology of Penicillium nalgiovense CNUFC CY224. (A, D) Colonies on Czapek yeast autolysate agar (CYA); (B, E) malt extract agar (MEA); (C, F) yeast extract sucrose agar (YES) (A–C: obverse view and D–F: reverse view); (G–I) Conidiophores; (J) Conidia. Scale bars = 10 µm.
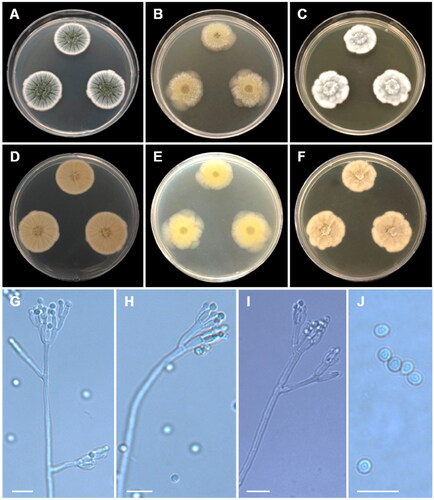
Description: On CYA at 25 °C, colonies radially sulcate; margins entire; mycelium white and greenish grey; colony texture velvety to floccose; sporulation moderate to strong; reverse grayish yellow and reached 29–30 mm in diameter after seven days. On MEA at 25 °C, colonies texture floccose, mycelia white; sporulation poor or absent; soluble pigments absent; reverse moderate to strong yellow and reached 26–30 mm in diameter after seven days. On YES at 25 °C, colonies raised at center; radially and concentrically sulcate; margins irregular; mycelia white; sporulation moderate; reverse grayish yellow and reached 26–29 mm in diameter after seven days.
Micromorphology: Conidiophores biverticillate, terverticillate, and quaterverticillate. Metulae 6–15 × 2–3 µm. Phialides flask-shaped, 4.5–12 × 2–2.5 µm. Conidia globose to subglobose, smooth, 2.5–3.5 µm in diam.
Material examined: Republic of Korea, Kunryang-ri, Cheongyang-eup, Cheongyang, Chungnam Province (36°26’16.2”N 126°46’04.7”E), from soil, May 15, 2022, H.B. Lee (culture CNUFC CY224).
Notes: The morphological characters, such as biverticillate, terverticillate, and quaterverticillate conidiophores, flask-shaped phialides, and globose to subglobose conidia, of our strain were similar to the ex-type strain of P. nalgiovense [Citation51].
Talaromyces atroroseus N. Yilmaz, Frisvad, Houbraken & Samson, PLoS One 8(12): e84102,8 (2013) ():
Figure 10. Morphology of Talaromyces atroroseus CNUFC SJ322. (A, D) Colonies on Czapek yeast autolysate agar (CYA); (B, E) malt extract agar (MEA); (C, F) yeast extract sucrose agar (YES) (A–C: obverse view and D–F: reverse view); (G, H) Conidiophores; (I) Conidia. Scale bars: G = 20 µm, H, I = 10 µm.
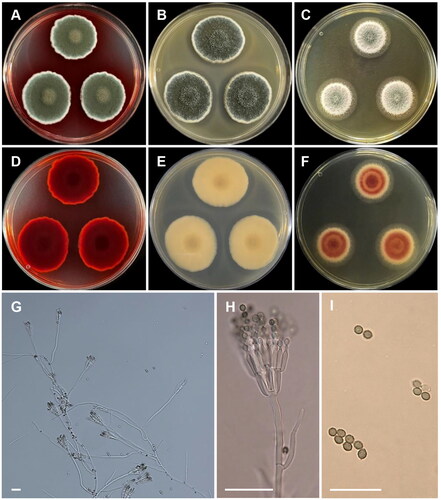
Description: On CYA at 25 °C, colonies texture floccose and velvety; mycelia white and olive green; sporulation strong; soluble pigments red; reverse dark red and reached 31–34 mm in diameter after seven days. On MEA at 25 °C, colonies texture floccose, mycelia white to grayish olive green; sporulation strong; soluble pigments absent; reverse pale greenish yellow and reached 32–38 mm in diameter after seven days. On YES at 25 °C, colonies slightly raised at center, texture velvety; mycelia white; sporulation poor; no soluble pigments; reverse vivid reddish orange and reached 29–37 mm in diameter after seven days.
Micromorphology: Conidiophores biverticillate. Stipes (83.5–)94–137.5(–154) × 2–3.5 μm. Metulae 8.5–12 × 2–3.5 μm. Phialides acerose, 8–11.5 × 2–3 μm. Conidia finely rough to rough, ellipsoidal, 2.5–3 × 2–2.5 μm.
Material examined: Republic of Korea, Namseong-dong, Sangju-si, Gyeongsangbuk-do (36.4109°N, 128.1591°E), from soil, July 31, 2019 (culture CNUFC SJ322).
Notes: The morphological characters of our strain were similar to the ex-type strain of T. atroroseus [Citation52].
Talaromyces georgiensis M. Guevara-Suarez, D.A. Sutton & N. Wiederhold, Mycoses 60 (10): 656 (2017) [MB#820460] ():
Figure 11. Morphology of Talaromyces georgiensis CNUFC DW211. (A, D) Colonies on Czapek yeast autolysate agar (CYA); (B, E) malt extract agar (MEA); (C, F) yeast extract sucrose agar (YES) (A–C: obverse view and D–F: reverse view); (G–I) Conidiophores; (J) Conidia. Scale bars = 10 µm.
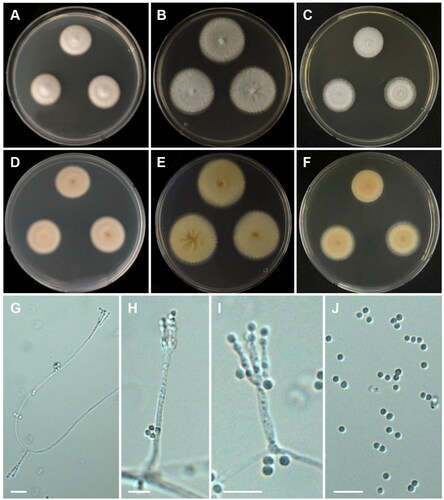
Description: On CYA at 25 °C, colonies raised at center; texture velvety; mycelium white; sporulation sparse; reverse yellowish white and reached 22–23 mm in diameter after seven days. On MEA at 25 °C, colonies cottony; mycelium white becoming greenish grey; sporulation moderate; reverse grayish yellow, and reached 31–33 mm in diameter after seven days. On YES at 25 °C, colonies cottony; mycelium white; margins entire; sporulation absent; exudates and soluble pigments absent; reverse pale yellow, and reached 23–26 mm in diameter after seven days.
Micromorphology: Conidiophores monoverticillate. Stipes rough-walled, 12.5–35.5(–37.5) x 2–3 μm. Phialides acerose, 2–4 per stipe, 7–13.5 × 2–3 μm. Conidia smooth to finely rough-walled, globose to subglobose, 2–3(–3.5) μm.
Material examined: Republic of Korea, Mannyeon-dong, Seo-gu, Daejeon East Dongsan of Hanbat Arboretum (36°22’06.4”N 127°23’24.9”E), from freshwater, February 15, 2020 (culture CNUFC DW211).
Notes: Compared with the ex-type strain of T. georgiensis, our strain grew slower on CYA (22–23 mm vs. 29–31 mm) and MEA (23–26 mm vs. 28–30 mm) [Citation26].
4. Discussion
Aspergillus, Penicillium, and Talaromyces species have been frequently isolated from various environments such as soil, water, air, seed, and food [Citation1,Citation8,Citation18,Citation19,Citation22]. However, isolation of fungi from insects is still limited. In this study, P. anthracinoglaciei species was isolated from insect sample (dead bee). They are of great interest to pharmacologists due to their ability to produce a wide range of biological activities, including anti-inflammatory, antibacterial, antioxidant, and anti-cancer properties. More than 315 bioactive metabolites of the genus Aspergillus and 221 compounds of the genus Talaromyces have been documented [Citation53,Citation54].
Eight isolates from different niches were identified as A. baeticus, A. griseoaurantiacus, A. spinulosporus, P. anthracinoglaciei, P. labradorum, P. nalgiovense, T. atroroseus, and T. georgiensis belonging to sections Usti, Nidulantes, Brevicompacta, Exilicaulis, Chrysogena, Trachyspermi, and Helici, respectively ().
Aspergillus spinulosporus acts as a biocontrol agent against Xanthomonas oryzae and produces signals that upregulate superoxide dismutase to protect plants. It also exhibits chitinolytic and amylolytic activities [Citation55]. Additionally, it causes infections in the central nervous system and is associated with unique microbiomes found in tumors [Citation56,Citation57]. Aspergillus spinulosporus has been isolated from patients with aspergillosis, birds, clinical samples, brain tissue, sputum, BAL samples, undetermined sources, and asthmatic patients [Citation56–61] and from soil in the current study. Aspergillus griseoaurantiacus exhibits the highest chitinase and chitosanase activities when cultured under solid-state fermentation of potato shell [Citation62]. Chitinase shows antifungal activity against the pathogenic fungus, Fusarium solani [Citation62]. Chitosan oligosaccharides, which are the degraded products of chitosan obtained through chitosanase, exhibit good antibacterial and antioxidant activities [Citation62]. Aspergillus griseoaurantiacus was isolated from house dust collected from Thailand, Mexico, and Yela of Kosrae Island [Citation48], as well as from a soil sample in this study. Aspergillus baeticus has been isolated from cave sediment, cave air and bat cadaver in Spain [Citation47,Citation63], and from soil environment in this study.
Penicillium anthracinoglaciei acts as decomposers or parasites of glacier ice algae by utilizing and converting the pigment purpurogallin carboxylic acid-6-O-β-d-glucopyranoside into purpurogallin carboxylic acid [Citation50]. The species was isolated from the surface of the Greenland Ice Sheet [Citation50] and from dead bee in rainwater in this study. Penicillium labradorum isolated from a Labrador Retriever with disseminated fungal disease shows some clinical signs, including lethargy, lymphadenopathy, tachypnea, moderate pitting edema, and non-weight bearing lameness in the right hind limb [Citation39]. Additionally, it was isolated from the bone tissue of a 6-year-old female Beagle dog [Citation64] and was identified as an indoor contaminant in this study. Penicillium nalgiovense has various applications in medicinal chemistry and organic synthesis. It is capable of producing isocoumarins such as dichlorodiaportin, diaportinol, and diaportinic acid [Citation65]. In addition, it is involved in coprogen, amphotericin B, and penicillin production, protease production, fermentation of salchichon, and serves as a starter culture in dry fermentation in the food industry [Citation66–70]. Penicillium nalgiovense was isolated from the soil from an abandoned penguin’s nest, from Ellischauer cheese [Citation51,Citation68], and from the soil in this study.
Talaromyces georgiensis has been isolated from clinical samples [Citation26], from animal joint fluid [Citation71], and a 4-year-old male Schnauzer dog [Citation64]. Our new isolate, T. georgiensis CNUFC DW211, was isolated from a freshwater habitat in Korea. Talaromyces atroroseus has been reported in respiratory specimens of patients with pulmonary disorders, house dust (from Mexico, Thailand, and South Africa), coprophilous, red sweet bell pepper, a contaminated Petri dish, mouse dung, soil, and as a parasite in Aspergillus niger culture [Citation8,Citation52,Citation72]. Talaromyces atroroseus from this study was isolated from soil sample. Talaromyces atroroseus secretes large amounts of red pigments that have great potential for coloring foods [Citation52] and many other compounds such as glauconic acid, glaucanic acid, Monascus red pigments, purpactins AC, purpuride, purpurogenone, N-glutarylmonascorubramine, monascorubrin, monascorubramine, rubropunctatin and ZG-1494α [Citation8,Citation52]. Therefore, future studies are required for the isolation and identification of compounds from Talaromyces atroroseus in Korea.
In this study, eight new records of Eurotiales were identified from different niches in Korea. Our results suggest that there are numerous undescribed species awaiting discovery in Korea. Further exploration and sampling could result in the discovery of fungal groups that could be utilized for various taxonomic and ecological research.
Disclosure statement
No potential conflict of interest was reported by the author(s).
Correction Statement
This article has been corrected with minor changes. These changes do not impact the academic content of the article.
Additional information
Funding
References
- Houbraken J, Kocsubé S, Visagie CM, et al. Classification of Aspergillus, Penicillium, Talaromyces and related genera (Eurotiales): an overview of families, genera, subgenera, sections, series and species. Stud Mycol. 2020;95:5–169. doi: 10.1016/j.simyco.2020.05.002.
- Houbraken J, de Vries RP, Samson RA. Modern taxonomy of biotechnologically important Aspergillus and Penicillium species. Adv Appl Microbiol. 2014;86:199–249. doi: 10.1016/B978-0-12-800262-9.00004-4.
- Cairns TC, Nai C, Meyer V. How a fungus shapes biotechnology: 100 years of Aspergillus niger research. Fungal Biol Biotechnol. 2018;5(1):13. doi: 10.1186/s40694-018-0054-5.
- Sun P, Wang M, Wang S, et al. Effect of fermentation with Penicillium roqueforti and Penicillium nalgiovense on physicochemical properties and microstructure of duck meat products. J Light Technol. 2022;37(4):18–25.
- Mousavi B, Hedayati MT, Hedayati N, et al. Aspergillus species in indoor environments and their possible occupational and public health hazards. Curr Med Mycol. 2016;2(1):36–42. doi: 10.18869/acadpub.cmm.2.1.36.
- Seyedmousavi S, Guillot J, Arné P, et al. Aspergillus and aspergilloses in wild and domestic animals: a global health concern with parallels to human disease. Med Mycol. 2015;53(8):765–797. doi: 10.1093/mmy/myv067.
- Frisvad JC. Taxonomy, chemodiversity, and chemoconsistency of Aspergillus, Penicillium, and Talaromyces species. Front Microbiol. 2014;5:773. doi: 10.3389/fmicb.2014.00773.
- Yilmaz N, Visagie CM, Houbraken J, et al. Polyphasic taxonomy of the genus Talaromyces. Stud Mycol. 2014;78:175–341. doi: 10.1016/j.simyco.2014.08.001.
- Tsang CC, Tang JYM, Lau SKP, et al. Taxonomy and evolution of Aspergillus, Penicillium and Talaromyces in the omics era – past, present and future. Comput Struct Biotechnol J. 2018;16:197–210. doi: 10.1016/j.csbj.2018.05.003.
- Chávez R, Bull P, Eyzaguirre J. The xylanolytic enzyme system from the genus Penicillium. J Biotechnol. 2006;123(4):413–433. doi: 10.1016/j.jbiotec.2005.12.036.
- Meyer V, Wu B, Ram AF. Aspergillus as a multi-purpose cell factory: current status and perspectives. Biotechnol Lett. 2011;33(3):469–476. doi: 10.1007/s10529-010-0473-8.
- De Vries RP, Riley R, Wiebenga A, et al. Comparative genomics reveals high biological diversity and specific adaptations in the industrially and medically important fungal genus Aspergillus. Genome Biol. 2017;18(1):28. doi: 10.1186/s13059-017-1151-0.
- Kumari M, Taritla S, Sharma A, et al. Antiproliferative and antioxidative bioactive compounds in extracts of marine-derived endophytic fungus Talaromyces purpureogenus. Front Microbiol. 2018;9:1777. doi: 10.3389/fmicb.2018.01777.
- Lei L-R, Gong L-Q, Jin M-Y, et al. Research advances in the structures and biological activities of secondary metabolites from Talaromyces. Front Microbiol. 2022;13:984801. doi: 10.3389/fmicb.2022.984801.
- Nicoletti R, Bellavita R, Falanga A. The outstanding chemodiversity of marine-derived Talaromyces. Biomolecules. 2023;13(7):1021. doi: 10.3390/biom13071021.
- Nicoletti R, Andolfi A, Becchimanzi A, et al. Anti-insect properties of Penicillium secondary metabolites. Microorganisms. 2023;11(5):1302. doi: 10.3390/microorganisms11051302.
- Micheli PA. Nova plantarum genera juxta Tournefortii methodum disposita. Florence, Italy: Typis Bernardi Paperinii; 1729.
- Samson RA, Visagie CM, Houbraken J, et al. Phylogeny, identification and nomenclature of the genus Aspergillus. Stud Mycol. 2014;78(1):141–173. doi: 10.1016/j.simyco.2014.07.004.
- Chen AJ, Hubka V, Frisvad JC, et al. Polyphasic taxonomy of Aspergillus section Aspergillus (formerly Eurotium), and its occurrence in indoor environments and food. Stud Mycol. 2017;88(1):37–135. doi: 10.1016/j.simyco.2017.07.001.
- Sklenář F, Jurjević Ž, Peterson SW, et al. Increasing the species diversity in the Aspergillus section Nidulantes: six novel species mainly from the indoor environment. Mycologia. 2020;112(2):342–370. doi: 10.1080/00275514.2019.1698923.
- Link HF. Observationes in ordines plantarum naturales. Dissertatio 1. Mag Ges Naturf Freunde Berlin. 1809;3:3–42.
- Visagie CM, Houbraken J, Frisvad JC, et al. Identification and nomenclature of the genus Penicillium. Stud Mycol. 2014;78(1):e343371. doi: 10.1016/j.simyco.2014.09.001.
- Pangging M, Nguyen TTT, Lee HB. Seven new records of Penicillium species belonging to section Lanata-Divaricata in Korea. Mycobiology. 2021;49(4):363–375. doi: 10.1080/12298093.2021.1952814.
- Torres-Garcia D, Gené J, García D. New and interesting species of Penicillium (Eurotiomycetes, Aspergillaceae) in freshwater sediments from Spain. MycoKeys. 2022;86:103–145. doi: 10.3897/mycokeys.86.73861.
- Benjamin CR. Ascocarps of Aspergillus and Penicillium. Mycologia. 1955;47(5):669–687. doi: 10.1080/00275514.1955.12024485.
- Guevara-Suarez M, Sutton DA, Gené J, et al. Four new species of Talaromyces from clinical sources. Mycoses. 2017;60(10):651–662. doi: 10.1111/myc.12640.
- Sun BD, Chen AJ, Houbraken J, et al. New section and species in Talaromyces. MycoKeys. 2020;68:75–113. doi: 10.3897/mycokeys.68.52092.
- Nguyen TTT, Frisvad JC, Kirk PM, et al. Discovery and extrolite production of three new species of Talaromyces belonging to sections Helici and Purpurei from freshwater in Korea. J Fungi. 2021;7(9):722. doi: 10.3390/jof7090722.
- Nguyen TTT, Lee HB. A new species and five new records of Talaromyces (Eurotiales, Aspergillaceae) belonging to section Talaromyces in Korea. Mycobiology. 2023;51(5):320–332. doi: 10.1080/12298093.2023.2265645.
- National Institute of Biological Resources. National species list of Korea. I. Plants, fungi, algae, prokaryotes. Seoul: Designzip; 2019.
- Pangging M, Nguyen TTT, Lee HB. Seven undescribed Aspergillus species from different niches in Korea. Mycobiology. 2022;50(4):189–202. doi: 10.1080/12298093.2022.2116158.
- Nguyen TTT, Noh KJK, Lee HB. New species and eight undescribed species belonging to the families Aspergillaceae and Trichocomaceae in Korea. Mycobiology. 2021;49(6):534–550. doi: 10.1080/12298093.2021.1997461.
- White TJ, Bruns T, Lee S, et al. Amplification and direct sequencing of fungal ribosomal RNA genes for phylogenetics. In: Innis MA, Gelfand DH, Sninsky JJ, White TJ, editors. PCR protocols: a guide to methods and applications. San Diego (CA): Academic Press, 1990. p. 315–322.
- de Hoog GS, Gerrits van den Ende AH. Molecular diagnostics of clinical strains of filamentous Basidiomycetes. Mycoses. 1998;41(5–6):183–189. doi: 10.1111/j.1439-0507.1998.tb00321.x.
- Aveskamp MM, Verkley GJ, de Gruyter J, et al. DNA phylogeny reveals polyphyly of Phoma section Peyronellaea and multiple taxonomic novelties. Mycology. 2009;101(3):363–382. doi: 10.3852/08-199.
- Hubka V, Nováková A, Peterson SW, et al. A reappraisal of Aspergillus section Nidulantes with descriptions of two new sterigmatocystin-producing species. Plant Syst Evol. 2016;302(9):1267–1299. doi: 10.1007/s00606-016-1331-5.
- Hong SB, Cho HS, Shin HD, et al. Novel Neosartorya species isolated from soil in Korea. Int J Syst Evol Microbiol. 2006;56(2):477–486. doi: 10.1099/ijs.0.63980-0.
- Liu YJ, Whelen S, Hall BD. Phylogenetic relationships among ascomycetes: evidence from an RNA polymerase II subunit. Mol Biol Evol. 1999;16(12):1799–1808. doi: 10.1093/oxfordjournals.molbev.a026092.
- Rothacker T, Jaffey JA, Rogers ER, et al. Novel Penicillium species causing disseminated disease in a Labrador Retriever dog. Med Mycol. 2020;58(8):1053–1063. doi: 10.1093/mmy/myaa016.
- Index Fungorum. http://www.indexfungorum.org/names/names.asp [accessed 1 October 2023].
- Katoh K, Rozewicki J, Yamada KD. MAFFT online service: multiple sequence alignment, interactive sequence choice and visualization. Brief Bioinform. 2019;20(4):1160–1166. doi: 10.1093/bib/bbx108.
- Tamura K, Stecher G, Peterson D, et al. MEGA7: molecular evolutionary genetics analysis version 7.0. Mol Biol Evol. 2013;30(12):2725–2729. doi: 10.1093/molbev/mst197.
- Rambaut A. FigTree, version 1.3. 1. Computer program distributed by the author; 2009. Available from: http://www.treebioedacuk/software/fgtree
- Pitt JI. The genus Penicillium and its teleomorphic states Eupenicillium and Talaromyces. London (UK): Academic Press Inc. Ltd.; 1919. 634 pp.
- Blakeslee A. Lindner’s roll tube method of separation cultures. Phytopathology. 1915;5:68–69.
- Frisvad JC. Physiological criteria and mycotoxin production as aids in identification of common asymmetric penicillia. Appl Environ Microbiol. 1981;41(3):568–579. doi: 10.1128/aem.41.3.568-579.1981.
- Nováková A, Hubka V, Saiz-Jimenez C, et al. Aspergillus baeticus sp. nov. and Aspergillus thesauricus sp. nov., two species in section Usti from Spanish caves. Int J Syst Evol Microbiol. 2012;62(Pt 11):2778–2785. doi: 10.1099/ijs.0.041004-0.
- Visagie CM, Hirooka Y, Tanney JB, et al. Aspergillus, Penicillium and Talaromyces isolated from house dust samples collected around the world. Stud Mycol. 2014;78(1):63–139. doi: 10.1016/j.simyco.2014.07.002.
- Chen AJ, Frisvad JC, Sun BD, et al. Aspergillus section Nidulantes (formerly Emericella): polyphasic taxonomy, chemistry and biology. Stud Mycol. 2016;84(1):1–118. doi: 10.1016/j.simyco.2016.10.001.
- Perini L, Gostinčar C, Likar M, et al. Interactions of fungi and algae from the Greenland Ice Sheet. Microb Ecol. 2022;86(1):282–296. doi: 10.1007/s00248-022-02033-5.
- Laxa O. Über die Reifung des Ellischauer Käses. Zentralblatt für Bakteriologie und Parasitenkunde Abteilung. 1932;2(86):160–165.
- Frisvad JC, Yilmaz N, Thrane U, et al. Talaromyces atroroseus, a new species efficiently producing industrially relevant red pigments. PLOS One. 2013;8(12):e84102. doi: 10.1371/journal.pone.0084102.
- Zhang X, Li Z, Gao J. Chemistry and biology of secondary metabolites from Aspergillus genus. J Nat Prod. 2018;8(4):275–304.
- Zhai MM, Li J, Jiang CX, et al. The bioactive secondary metabolites from Talaromyces species. Nat Prod Bioprospect. 2016;6(1):1–24. doi: 10.1007/s13659-015-0081-3.
- Jain A, Chatterjee A, Das S. Synergistic consortium of beneficial microorganisms in rice rhizosphere promotes host defense to blight-causing Xanthomonas oryzae pv. oryzae. Planta. 2020;252(6):106. doi: 10.1007/s00425-020-03515-x.
- York A. Tumour-specific microbiomes. Nat Rev Microbiol. 2020;18(8):413–413. doi: 10.1038/s41579-020-0405-0.
- Li Q, Kong D, Wang Y, et al. Characterization of a rare clinical isolate of A. spinulosporus following a central nervous system infection. Microbes Infect. 2022;24(5):104973. doi: 10.1016/j.micinf.2022.104973.
- Siqueira JP, Wiederhold N, Gené J, et al. Cryptic Aspergillus from clinical samples in the USA and description of a new species in section Flavipedes. Mycoses. 2018;61(11):814–825. doi: 10.1111/myc.12818.
- Sabino R, Burco J, Valente J, et al. Molecular identification of clinical and environmental avian Aspergillus isolates. Arch Microbiol. 2019;201(2):253–257. doi: 10.1007/s00203-019-01618-y.
- Tavakoli M, Rivero-Menendez O, Abastabar M, et al. Genetic diversity and antifungal susceptibility patterns of Aspergillus nidulans complex obtained from clinical and environmental sources. Mycoses. 2020;63(1):78–88. doi: 10.1111/myc.13019.
- Steenwyk JL, Lind AL, Ries LN, et al. Pathogenic allodiploid hybrids of Aspergillus fungi. Curr Biol. 2020;30(13):2495–2507.e7. doi: 10.1016/j.cub.2020.04.071.
- Shehata AN, Abd El Aty AA, Darwish DA, et al. Purification, physicochemical and thermodynamic studies of antifungal chitinase with production of bioactive chitosan-oligosaccharide from newly isolated Aspergillus griseoaurantiacus. Int J Biol Macromol. 2018;107(Pt A):990–999. doi: 10.1016/j.ijbiomac.2017.09.071.
- Jurado V, Del Rosal Y, Liñan C, et al. Diversity and seasonal dynamics of airborne fungi in Nerja Cave, Spain. Appl Sci. 2021;11(13):6236. doi: 10.3390/app11136236.
- Monpierre L, Soetart N, Valsecchi I, et al. Penicillium and Talaromyces spp. emerging pathogens in dogs since 1990s. Med Mycol J. 2023;61(8):myad087.
- Larsen TO, Breinholt J. Dichlorodiaportin, diaportinol, and diaportinic acid: three novel isocoumarins from Penicillium nalgiovense. J Nat Prod. 1999;62(8):1182–1184. doi: 10.1021/np990066b.
- Andersen SJ, Frisvad JC. Penicillin production by Penicillium nalgiovense. Lett Appl Microbiol. 1994;19(6):486–488. doi: 10.1111/j.1472-765x.1994.tb00988.x.
- Emri T, Tóth V, Nagy CT, et al. Towards high-siderophore-content foods: optimisation of coprogen production in submerged cultures of Penicillium nalgiovense. J Sci Food Agric. 2013;93(9):2221–2228. doi: 10.1002/jsfa.6029.
- Svahn KS, Chryssanthou E, Olsen B, et al. Penicillium nalgiovense Laxa isolated from Antarctica is a new source of the antifungal metabolite amphotericin B. Fungal Biol Biotechnol. 2015;2(1):1–8. doi: 10.1186/s40694-014-0011-x.
- Ludemann V, Greco M, Rodríguez MP, et al. Conidial production by Penicillium nalgiovense for use as starter cultures in dry fermented sausages by solid state fermentation. LWT Food Sci Technol. 2010;43(2):315–318. doi: 10.1016/j.lwt.2009.07.011.
- Papagianni M, Sergelidis D. Purification and biochemical characterization of a novel alkaline protease produced by Penicillium nalgiovense. Appl Biochem Biotechnol. 2014;172(8):3926–3938. doi: 10.1007/s12010-014-0824-3.
- Okada K, Kano R, Hasegawa T, et al. Granulomatous polyarthritis caused by Talaromyces georgiensis in a dog. J Vet Diagn Invest. 2020;32(6):912–917. doi: 10.1177/1040638720957964.
- Guevara-Suarez M, García D, Cano-Lira JF, et al. Species diversity in Penicillium and Talaromyces from herbivore dung, and the proposal of two new genera of Penicillium-like fungi in Aspergillaceae. Fungal Syst Evol. 2020;5(1):39–75. doi: 10.3114/fuse.2020.05.03.