Abstract
Candida ranks as among the most frequently encountered fungal infections that associated with high morbidity and mortality. Quinoxaline derivatives are a group of small molecules that showed a promising antimicrobial activity. This study aimed to investigate the fungicidal effects of 3-hydrazinoquinoxaline-2-thiol against Candida in comparison with Amphotericin B in vitro as a reference. Also, we aim to assess the efficacy of 3-hydrazinoquinoxaline-2-thiol in vivo using mice oral candidiasis model. Fifty-six Candida isolates were subjected to susceptibility testing by broth microdilution method for 3-hydrazinoquinoxaline-2-thiol and Amphotericin B. Therefore, Minimal inhibitory concentrations (MIC) were assessed and compared. The oral candidiasis mice model was used to evaluate the activity of 3-hydrazinoquinoxaline-2-thiol in vivo. Microbiological evaluation of progression and ELISA were used in this study. 3-hydrazinoquinoxaline-2-thiol was more effective than Amphotericin B against most clinical isolates of Candida albicans. Higher effectiveness was seen against Candida glabrata and Candida parapsilosis isolates. However, the efficiency against Candida tropicalis isolates varies. 3-hydrazinoquinoxaline-2-thiol was also effective against Pichia kudriavzevii and Clavispora lusitaniae. 3-hydrazinoquinoxaline-2-thiol showed a good efficacy in mice model against C. albicans cells ATCC 10231. 3-hydrazinoquinoxaline-2-thiol has shown promising antifungal and anti-inflammatory activity against different Candida species. More tests and experiments are needed.
1. Introduction
Several publications and expert opinions have emphasized the global burden of fungal diseases on human health [Citation1]. Fungal infections are becoming increasingly common, posing a significant challenge to healthcare practitioners. This rise is related to the expanding number of immunocompromised patients due to various reasons including the use of chemotherapy and immunosuppressive drugs, HIV, immunosuppressive disorders, and viral infection as COVID-19 [Citation2,Citation3].
Systemic fungal infections can be produced by an opportunistic organism that infects a vulnerable person. Systemic infections may be linked to a significant incidence of illness and death. Candida albicans and Aspergillus species are the most commonly seen pathogens, nevertheless, non-albicans Candida species are progressively gaining prominence [Citation4].
Polyenes, pyrimidines, allylamines, azoles and echinocandins are the five structural classes of antifungal medicines now utilized to treat fungal infections [Citation5]. Aside from drug resistance, polyenes have been demonstrated to be very toxic with a narrow therapeutic index. Both polyenes and echinocandins have a poor oral availability that limited there administration to intravenous and required prolong hospital stay [Citation6]. While, factor such as variable bioavailability, acute adverse effects, and the emergence of resistance limit the use of extended-spectrum triazoles, posaconazole, and voriconazole [Citation7]. Furthermore, voriconazole, posaconazole, itraconazole, and isavuconazole are known as cytochrome P450 3A4 inhibitors, and this enzyme plays a crucial role in the metabolism of immunosuppressive medications like cyclosporine, tacrolimus, and sirolimus. Consequently, when a triazole antifungal drug is taken alongside these immunosuppressant drugs, there is a potential for elevating the plasma levels of the immunosuppressants, which can lead to toxicity. Conversely, when the triazole antifungal drug is discontinued, it may inadvertently reduce the concentrations of these immunosuppressants, increasing the risk of rejection or graft-versus-host disease [Citation8].
Despite the availability of novel antifungal medications, treating fungal infections in the central nervous system (CNS) remains difficult due to poor access to drugs with high molecular weight or the hydrophilic nature of several antifungal drugs [Citation9]. Given these limitations of conventional FDA-approved antifungals, there is a need to discover novel antifungal drugs with lower toxicity, and favorable pharmacokinetic characteristics. In this context several novel antifungal compounds with novel mechanisms were reported, for example, MMV668271 which targets the ability of C. neoformans to withstand stress at the plasma membrane and cell wall. The compound has high potency and low toxicity in mammalian cells [Citation10]. Fosmanogepix, ibrexafungerp, rezafungin, encochleated amphotericin B, oteseconazole (VT-1161), VT-1598, PC945, and olorofim are promising experimental antifungal medicines in clinical development [Citation11].
Alfadil and his colleagues confirmed that quinoxaline derivatives such as 2,3-dimethylquinoxaline (DMQ) had a broad-spectrum antifungal activity [Citation12]. Moreover, the compound showed promising wound-healing activity [Citation13]. Therefore, the present study aims to explore another novel antifungal activity called 3-hydrazinoquinoxaline-2-thiol derivative with a promising antimicrobial activity. It will be assessed in vitro and in vivo against different Candida infection.
2. Materials and methods
2.1. Fungal species, media, and growth condition
Fifty-six fungal samples, clinical and reference strains were utilized for in vitro studies with a huge diversity of pathogenic Candida, including Candida albicans, Candida tropicalis, Candida parapsilosis, Candida glabrata, Clavispora lusitaniae and Pichia kudriavzevii. A reference American Type Culture Collection strain Candida albicans ATCC 10231 was used for both in vitro and in vivo studies. These strains were stored at −80 °C degree with 20% glycerol in Sabouraud dextrose broth (source). To cultivate cells, they were streaked in Sabouraud dextrose agar at 37 °C for 36 h. The clinical isolates used in this study were procured from the Department of Medical Laboratory Technology within the Faculty of Applied Medical Sciences at King Abdulaziz University, Jeddah, Saudi Arabia. The collection of samples was conducted in accordance with the ethical guidelines and research regulations established by the Faculty of Applied Medical Sciences at King Abdulaziz University (Ethics Committee reference number: 38-712-456). Since the clinical isolates were collected as part of the hospital’s routine laboratory procedures, the ethics committee granted an exemption from the requirement for informed consent in this research.
2.2. Drug and chemical
3-hydrazinoquinoxaline-2-thiol was purchased from Sigma Aldrich and used without further purification. The stock solution of 3-hydrazinoquinoxaline-2-thiol was prepared in 100% dimethyl sulfoxide (DMSO; Sigma Aldrich-D8418, Taufkirchen, Germany). The working solution of 3-hydrazinoquinoxaline-2-thiol was diluted in RPMI-1640 (Gibco, Maryland, United States of America) medium to a final DMSO concentration of less than 5%.
2.3. Preparation of 3-hydrazinoquinoxaline-2-thiol gel formulation
To prepare 1% hydrogel of 3-hydrazinoquinoxaline-2-thiol, 2 grams of hydroxypropyl methylcellulose (HPMC Sigma Aldrich-H7509, Taufkirchen, Germany) powder was added to an appropriate beaker and mixed with 50 ml of purified hot water and allowed to soak. Then, 10 ml of glycerol (Sigma Aldrich-G5516, Taufkirchen, Germany) was added and allowed further soaking. The beaker was covered and left for 24 h at room temperature to allow the gel to form. To formulate 3-hydrazinoquinoxaline-2-thio hydrogel at 1% w/w, 1 gram of 3-hydrazinoquinoxaline-2-thio were dissolved in 5 milliliters of 99% methanol and 35 ml distilled water. The drug solution in methanol was then gradually added to 45 grams of the plain HPMC gel, with continuous gentle mixing to form homogenous gel. The gel was stored in suitable umber glass jars at 4 °C in order to protect it from light [Citation14].
2.4. In vitro susceptibility of 3-hydrazinoquinoxaline-2-thio
In this study, the antifungal efficacy of 3-hydrazinoquinoxaline-2-thio was assessed through in vitro testing involving fifty-six clinical isolates and one reference strain. To determine the minimum inhibitory concentration (MIC), the procedure followed the guidelines outlined by the Clinical and Laboratory Standards Institute (CLSI) [Citation15].
An inoculum containing 5 × 103 colony-forming units per milliliter (CFU/ml) was prepared from a 36-hour culture grown on sabouraud dextrose agar incubated at 35 °C. In a 96-well microtiter plate, 100 μl of RPMI-1640 medium was distributed to each well. The compound 3-hydrazinoquinoxaline-2-thiol, initially at a concentration of 3 mg/mL, was added to the second column of the plate (200 μl per well). Subsequent columns underwent a two-fold serial dilution. This resulted in a range of concentrations for 3-hydrazinoquinoxaline-2-thio, spanning from 64 to 0.06 μg/mL. The inoculum was then added at 100 μl per well to the second and subsequent columns up to the last column, which served as the positive control, containing only the inoculum. The first column represented the negative control, consisting of wells with RPMI-1640 medium only. Following the setup, the plates were incubated at 37 °C for a duration of 36 h. This testing procedure was conducted in duplicate on the same day and was repeated in triplicate over the course of two weeks [Citation16,Citation17].
2.5. Animals
Six to eight weeks-old male BALB/c mice (purchased form faculty of pharmacy in King Abdulaziz university animal house) were utilized in the study. These mice had unrestricted access to both food and water and were subject to daily monitoring for 30 days before the challenge was initiated. The animal experiments involved 32 male mice. These mice were categorized into five groups, with each group further subdivided into two shoebox cages. Each cage accommodated 4-5 mice. This grouping strategy was employed to maintain lower levels of aggression and promote overall health by recommendations from the National Research Council (US) Institute for Laboratory Animal Research in 2004. The animal cages were situated within a temperature-controlled environment, maintaining a stable temperature of 23 ± 2 °C, and they followed a light/dark cycle of 12 h each. Any animals that either survived until the conclusion of the experiment or were in a moribund state were humanely euthanized. This euthanasia process involved administering an An overdose of a combination of ketamine and xylazine was administered through intraperitoneal injection, and this was followed by cervical dislocation. Group 1 consisted of mice designated as the uninfected control group (n = 2). Group 2 was identified as the stress-exposed group, which received an inoculum but no additional treatment (n = 3). Group 3 was allocated to mice that received 3-hydrazinoquinoxaline-2-thiol at a concentration of 0.002 alongside an inoculum of C. albicans ATCC 10231 (n = 9). Group 4 included mice that received 3-hydrazinoquinoxaline-2-thiol at a concentration of 0.02 in conjunction with a C. albicans ATCC 10231 inoculum (n = 9). Group 5 was composed of mice that were administered 3-hydrazinoquinoxaline-2-thiol at a concentration of 0.2 in combination with a C. albicans ATCC 10231 inoculum (n = 9). All animal experiments were conducted in accordance with the animal care and use guidelines approved by King Abdulaziz University. The animal study protocol received approval from the Institutional Animal Care at King Abdulaziz University (faculty of pharmacy in King Abdulaziz university animal house) This approval adheres to the guidelines set forth by the Canadian Council on Animal Care (CCAC) [Citation18].
2.6. Animal preparation and oral infection
For the induction of oral candidiasis, the mice were subjected to treatment and infection with C. albicans ATCC 10231 in the following manner. The animals underwent immunosuppression through a series of two subcutaneous injections of prednisolone (Sigma Aldrich-P6004, Taufkirchen, Germany) at a dosage of 100 mg/kg. These injections were administered one day before and three days following the Candida albicans ATCC 10231 infection. Additionally, tetracycline hydrochloride (Sigma Aldrich-T7660, Taufkirchen, Germany) was introduced into the mice’s drinking water at a concentration of 0.9 mg/ml, commencing one day before the infection. The animals were rendered unconscious through intramuscular injections of 2 mg/ml chlorpromazine chloride (sigma), with 50 μl administered on each femur.
Small cotton pads (baby cotton buds from Johnson & Johnson) were saturated with a cell suspension of C. albicans ATCC 10231 containing 2.0 × 108 viable cells per milliliter. These saturated cotton pads were then used to swab the entire oral cavity of the anesthetized mice, inducing oral infections. The daily assessment of infection severity was based on the presence and intensity of whitish, curd-like patches observed on the surface of the tongue [Citation18].
2.7. Assessment of the progression of infections
In this model, the endpoint for infection evaluation was set at day 7. On this day, groups of mice were anesthetized and subsequently sacrificed to evaluate the severity of tongue lesions. The macroscopic assessment of the infection was represented by assigning scores ranging from 0 to 4, considering the extent and severity of whitish, curd-like patches on the tongue surface. The scoring criteria were as follows: 0 for normal; 1 for white patches covering less than 20%; 2 for white patches encompassing less than 90% but more than 21%; 3 for white patches covering more than 91%; and 4 for thick white patches resembling pseudomembranes and covering more than 91% [Citation19].
2.8. Microbiological evaluation of progression of the Candida infection
The assessment procedure was conducted as follows: the entire oral cavity, encompassing the buccal mucosa, tongue, soft palate, and other oral mucosal surfaces, was swabbed using a cotton pad. Samples were collected at day 7 (which equated to 24 h after the final treatment). This was achieved by gently rolling a sterile cotton swab over the oral cavity, followed by suspending it in 1 mL of sterile saline.
The cotton pad was trimmed at its end and then placed into a tube filled with 1 ml sterile saline. After thorough mixing using a Vortex mixer to release Candida cells ATCC 10231 from the swab, 25 µl samples from this suspension were duplicated, following a one-hundred-fold serial dilution on Sabouraud agar plates containing 0.05% chloramphenicol. Then, the cell suspension was incubated on Candida plates at 37 °C for 36 h and the resulting colonies were counted. The number of viable cells was determined using the drop count method to calculate the logarithm of CFU/mL [Citation19].
2.9. Antifungal treatment
Seventy-two hours after the initial infection, a total of 32 animals were categorized into three groups. These groups underwent treatment by applying 0.5 mL of 3-hydrazinoquinoxaline-2-thiol (the application was carried out 3 h after the initial inoculation) with varying concentrations (0.002, 0.02, and 0.2 mg/ml) to the oral cavity once a day for a continuous period of eight days, spanning from day 0 to day 7. This topical agent was directly administered to cover the entire oral cavity, encompassing the tongue, buccal mucosa, and soft palate, using a cotton swab. For the control group, infected animals that did not receive treatment, 0.5 mL of sterile saline was orally administered (containing 0.8% agar) once a day. As a negative control group, animals that were immunosuppressed but not infected were employed. The assessment of antifungal efficacy was conducted on day 3 post-infection for this group [Citation19].
2.10. ELISA based quantification of selected inflammatory marker
For inflammation assessment, the quantification of TNF-α, IL-6, IL-B1, COX2, iNOS, and IFN gamma was conducted using dedicated ELISA Kits (TNF alpha ELISA Kit (ab208348), IL-6 ELISA Kit (ab222503), Mouse IL-1 B ELISA Kit (ab197742), COX2 ELISA Kit (ab210574), iNOS ELISA Kit (ab253219), Mouse TNF alpha ELISA Kit (ab208348)). These kits featured ELISA plates pre-coated with monoclonal antibodies targeting the specific cytokine under investigation, utilizing a quantitative sandwich enzyme immunoassay technique. All procedures strictly adhered to the manufacturer’s protocols, and the kits employed were procured from Abcam [Citation20].
2.11. Statistical analysis
Statistical analysis in the study involved employing GraphPad Prism version 9. For each experiment, a minimum of two repetitions were conducted, and the average values were calculated. We employed an unpaired t-test to assess significant differences and conduct statistical comparisons among the experimental groups. A significance level of 0.05 was utilized to assess statistical significance, with values equal to or less than 0.05 considered significant. The p-values were denoted as follows: *p < 0.05, **p < 0.01, ***p < 0.001, and ****p < 0.0001. Comprehensive details regarding the statistical analysis for each figure are provided in their respective legends.
3. Results
3.1. 3-hydrazinoquinoxaline-2-thiol is effective as Amphotericin B in vitro
Broth microdilution was used to assess the efficacy of 3-hydrazinoquinoxaline-2-thiol in vitro. The antifungal activity (MIC) of the 3-hydrazinoquinoxaline-2-thiol compared to Amphotericin B is presented in . 3-hydrazinoquinoxaline-2-thiol showed higher efficacy than Amphotericin B against most clinical isolates of Candida albicans after 24 hr. Higher efficacy against isolates of Candida glabrata and Candida parapsilosis was observed. However, the efficacy against isolates of Candida tropicalis is variable. 3-hydrazinoquinoxaline-2-thiol also showed good efficacy against Pichia kudriavzevii and Clavispora lusitaniae.
Table 1. The antifungal activity of 3-hydrazinoquinoxaline-2-thiol was evaluated using a broth microdilution assay and compared to that of Amphotericin B.
3.2. Macroscopically evaluation of oral mucosa of mice
To evaluate the oral status after inducing a Candida infection, we examined the oral symptoms in infected mice and assigned scores according to the size and intensity of white patches present on their tongues. illustrates the visual inspection of characteristic lesions, which are comprised of white patches on the tongues of mice with oral candidiasis.
Figure 1. An extensive visual examination was conducted to analyze the standard lesions observed on the tongues of mice afflicted with oral candidiasis. These lesions are typically characterized by the presence of white patches, indicative of fungal infection. The examination involved careful scrutiny of each mouse’s tongue under standardized conditions to distinguish between two distinct states: (A) tongues exhibiting a normal appearance without any discernible abnormalities, and (B) tongues displaying the characteristic white patches associated with oral candidiasis. This meticulous observation process allowed for the detailed characterization and differentiation of the two tongue conditions, facilitating a comprehensive assessment of the extent of fungal infection in the experimental mice.
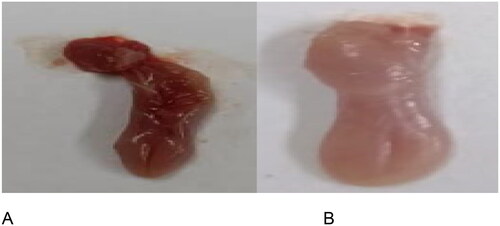
3.3. The in vivo anti Candida activity of 3-hydrazinoquinoxaline-2-thiol was assessed in a mice oral infection model
Quinoxaline is known for its notable biological properties, including antitubercular, antibacterial, and anti-inflammatory activities [Citation21]. Thus, we employed a mouse model to evaluate the in vivo effectiveness of 3-hydrazinoquinoxaline-2-thiol against C. albicans ATCC 10231, mirroring the results observed in vitro. When we administered 3-hydrazinoquinoxaline-2-thiol to mice at varying concentrations (0.002, 0.02, and 0.2 mg/ml), we observed a significant reduction in TNF-A levels compared to the infected mice group that received no treatment, with a p-value below 0.05 ().
Figure 2. The in vivo effectiveness of 3-hydrazinoquinoxaline-2-thiol using mice model against Candida albicans ATCC 10231. When 3-hydrazinoquinoxaline-2-thiol was administered to mice at various concentrations (0.002, 0.02, and 0.2 mg/ml), we noted a notable decrease in the levels of various inflammatory markers in comparison to the infected mice group that did not receive any treatment. This reduction was statistically significant, with a p-value below 0.05. G1 represents group received 0.002 mg/ml, G2 represents group received 0.02 mg/ml, G3 group received 0.2 mg/ml. (A) TNF-A, (B) IL6, (C) IL-B1, (D) Cox2, (E) INOs, (F) IFN-Y. The levels of TNF-A, IL6, IL-B1, Cox2, iNOS, and IFN-Y were measured in picograms per milliliter (pg/ml) for each respective inflammatory marker.
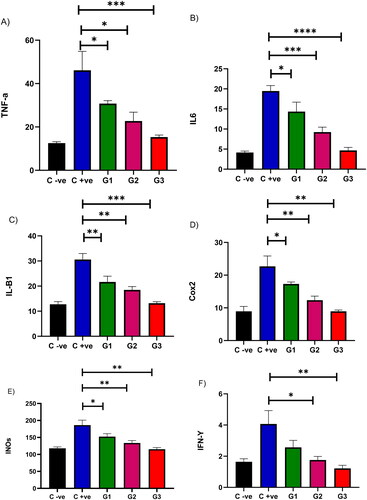
Furthermore, as we administered 3-hydrazinoquinoxaline-2-thiol to mice at different concentrations (0.002, 0.02, and 0.2), we noted a notable decrease in IL6 levels compared to the untreated infected mice group, and the p-value was less than 0.05. Intriguingly, a more pronounced reduction in IL6 was evident with higher doses of 3-hydrazinoquinoxaline-2-thiol ().
Comparable results were observed when we administered 3-hydrazinoquinoxaline-2-thiol to mice at various concentrations (0.002, 0.02, and 0.2 mg/ml). We observed a significant reduction in IL-B1 levels compared to the infected mice group that did not receive treatment, with a p-value below 0.05 ().
Moreover, when we applied 3-hydrazinoquinoxaline-2-thiol to mice at varying concentrations (0.002, 0.02, and 0.2), we observed a significant reduction in Cox2 levels compared to the infected mice group that received no treatment, with a p-value below 0.05. Interestingly, a more substantial reduction in Cox2 was observed when higher doses of 3-hydrazinoquinoxaline-2-thiol were administered ().
As we administered 3-hydrazinoquinoxaline-2-thiol to mice at different concentrations (0.002, 0.02, and 0.2 mg/ml), we noted a substantial decrease in INOs levels when compared to the infected mice group that did not receive treatment, and the p-value was less than 0.05 ().
Moreover, when we administered 3-hydrazinoquinoxaline-2-thiol to mice at different levels of concentration (0.002, 0.02, and 0.2 mg/ml), we witnessed a noteworthy decrease in IFN gama levels when compared to the untreated infected mice group, and the p-value was below 0.05. Interestingly, when a higher dose of 3-hydrazinoquinoxaline-2-thiol was administered, the reduction in IFN gama levels was almost identical between groups 2 and 3 (). Suggesting that 3-hydrazinoquinoxaline-2-thiol can be used for a dual effect, anti-inflammatory effect and anti-fungal effect.
3.4. Microbiology progression
Once colonization was confirmed through the visual observation of white patches on the tongue’s surface, our objective was to evaluate the microbiological development of Candida cells. As depicted in , there was a notable decrease in the quantity of C. albicans cells ATCC 10231 in the oral cavity of mice following the administration of 0.5 mL of 3-hydrazinoquinoxaline-2-thiol at different concentrations (0.002, 0.02, and 0.2 mg/ml). This implies that 3-hydrazinoquinoxaline-2-thiol exhibits promising in vitro antifungal activity, as evidenced by its performance in vivo.
Figure 3. In the context of mouse oral infections, at day 7, the group that received no treatment showed the highest colony-forming unit (CFU) counts. Conversely, the group treated with 0.5 mL of 3-hydrazinoquinoxaline-2-thiol at a concentration of 0.2 mg/ml exhibited the lowest CFU counts. A notable and statistically significant difference was observed between the control group and the treated groups of mice, with a p-value less than 0.05. Moreover, the CFU counts in the treated group decreased as the concentration of 3-hydrazinoquinoxaline-2-thiol increased.
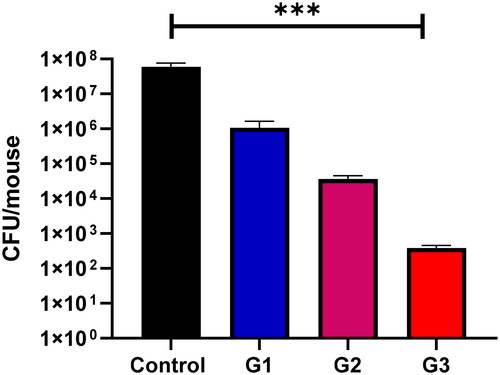
4. Discussion
Candida species are opportunistic infections that cause significant illness and mortality worldwide, posing a substantial danger to public health. Candida species can also lead to conditions such as vaginal yeast infections, oral thrush, skin yeast infections, bloodstream yeast infections (candidemia), and systemic infections [Citation22]. Candidemia stands as the predominant healthcare-associated infection, responsible for approximately 15% of bloodstream infections, and Candida species are the primary culprits behind 50-70% of systemic fungal infections [Citation23]. C. albicans are one of the most isolated pathogenic species. Other species, such as C. tropicalis, C. glabrata, C. krusei, C. famata, C. parapsilosis, C. guilliermondii, and C. lusitaniae, have been isolated in increasing numbers, primarily in HIV-infected patients [Citation24]. For the first time, we have demonstrated that 3-hydrazinoquinoxaline-2-thiol exhibits a dual effectiveness, both in vivo and in vitro, functioning as both an antifungal and anti-inflammatory agent.
The antifungal activity (MIC) of the 3-hydrazinoquinoxaline-2-thiol compared to Amphotericin B is presented in . 3-hydrazinoquinoxaline-2-thiol showed higher efficacy than Amphotericin B against most clinical isolates of Candida albicans after 24 hrs. Higher efficacy against isolates of C. glabrata and C. parapsilosis was observed. However, the efficacy against isolates of C. tropicalis is variable. 3-hydrazinoquinoxaline-2-thiol also showed good efficacy against Pichia kudriavzevii and Clavispora lusitaniae. Indicating that 3-hydrazinoquinoxaline-2-thiol displays promising antifungal properties and may present a viable remedy for various Candida infections, though additional testing is required to confirm its efficacy. Additionally, our research has revealed that the application of varying concentrations of 3-hydrazinoquinoxaline-2-thiol resulted in a significant reduction in the population of C. albicans cells ATCC 10231 within the oral cavity of mice. Furthermore, the levels of inflammatory markers were markedly diminished subsequent to the administration of 3-hydrazinoquinoxaline-2-thiol. Furthermore, these positive developments were evident in the overall tissue condition of the mice and the restoration of normal papillae morphology within the affected tissue lesions. Our data indicates that 3-hydrazinoquinoxaline-2-thiol has market potential, but further testing is essential.
Azoles are the favored and predominantly employed antifungal drugs for managing Candida infections. Different antifungal medications may be employed based on the specific infection type, its anatomical location, and the sensitivity profile of the fungal species involved. Polyenes, echinocandins, nucleoside analogues, and allylamines are among them [Citation25]. However, limitations of these drugs include resistance, toxicity and drug interaction provided a rationale for the development of new medications.
The presence of 2,3-dimethylquinoxaline (DMQ) in the Chromolaena odorata plant was discovered by Charles and Minakiri. They hypothesized that the antibacterial action of Chromolaena odorata was due to the presence of DMQ [Citation26]. Alfadil and his colleagues validated DMQ's wide antifungal action [Citation12]. Using Swiss ADME pharmacokinetic prediction software suggested that the compound has acceptable skin permeability Log Kp (skin permeation): −7.21 cm/s [Citation13,Citation27].
Numerous studies have shown that quinoxaline derivatives can eradicate various organisms by inhibiting DNA synthesis, ultimately leading to cell death. Additionally, quinoxaline derivatives have the ability to produce reactive oxygen species (ROS), which disrupt crucial cellular processes necessary for microbial survival, inducing oxidative stress and eventual cell demise [Citation28,Citation29]. Therefore, it is apparent that these distinct mechanisms collectively play a significant role in combating different Candida strains and resulting in the elimination of these cells.
The present study documented its efficacy against several clinical isolates of C. albicans (in vivo), C. glabrata and C. parapsilosis in vitro. We will assess the in vivo effectiveness of 3-hydrazinoquinoxaline-2-thiol against C. glabrata and C. parapsilosis. Furthermore, future work on 3-hydrazinoquinoxaline-2-thiol should prioritize several key areas to comprehensively evaluate its potential as a therapeutic agent against microbial infections. Safety assessment is paramount, involving rigorous toxicity studies to ascertain its impact on mammalian cells and organs, thus establishing its safety profile. Pharmacokinetic studies are essential to elucidate the compound’s absorption, distribution, metabolism and excretion properties, guiding dosing regimens for optimal efficacy. Pharmacodynamic investigations will provide insights into its mechanism of action and spectrum of activity against diverse pathogens, including biofilm cultures [Citation27,Citation29]. Resistance assays are crucial to assess the likelihood of microbial resistance emergence and potential synergistic effects with existing antibiotics [Citation30]. Finally, clinical trials, starting with phase I trials in healthy volunteers, will assess safety, tolerability, and pharmacokinetics, paving the way for subsequent phase II and III trials in patients with specific infectious diseases [Citation31–Citation33]. By addressing these areas, future research can provide valuable insights into the safety, pharmacokinetics, dynamics of resistance, and antimicrobial activity of 3-hydrazinoquinoxaline-2-thiol, facilitating its development as a promising therapeutic option against microbial infections (Supplementary file).
5. Conclusion
In a groundbreaking development, we have provided compelling evidence of the remarkable effectiveness of 3-hydrazinoquinoxaline-2-thiol, representing its inaugural demonstration of efficacy in both in vivo and in vitro environments. Our research demonstrates favorable MIC values against a range of Candida strains, alongside a notable reduction in viable cell counts and inflammatory markers in murine models. These findings highlight the promising potential of this compound as a viable solution for combating various Candida species. Further research and development efforts are necessary to advance this compound toward commercial viability.
Supplemental Material
Download MS Word (21.3 KB)Disclosure statement
The authors report no conflict of interest in this work.
Additional information
Funding
References
- Krysan DJ. The unmet clinical need of novel antifungal drugs. Virulence. 2017;8(2):135–137. doi:10.1080/21505594.2016.1276692.
- Von Lilienfeld-Toal M, Wagener J, Einsele H, et al. Invasive fungal infection: new treatments to meet new challenges. Dtsch Arztebl Int. 2019;116(16):271–278.
- José P, Alvarez-Lerma F, Maseda E, et al. Invasive fungal infection in crtically ill patients: hurdles and next challenges. J Chemother. 2019;31(2):64–73. doi:10.1080/1120009X.2018.1557799.
- Spencer S, Abbygail C, Brubaker KR, et al. Systemic fungal infections : a pharmacist/researcher perspective. Fungal Biol Rev. 2023;44:100293.
- Mota Fernandes C, et al. The future of antifungal drug therapy: novel compounds and targets. Antimicrob Agents Chemother. 2021;65(2):1–13.
- Parente-Rocha JA, Bailão AM, Amaral AC, et al. Antifungal resistance, metabolic routes as drug targets, and new antifungal agents: an overview about endemic dimorphic fungi. Mediators Inflamm. 2017;2017:9870679. vol doi:10.1155/2017/9870679.
- Miceli MH, Kauffman CA. Isavuconazole: a new Broad-Spectrum triazole antifungal agent. Clin Infect Dis. 2015;61(10):1558–1565. doi:10.1093/cid/civ571.
- Groll AH, et al. Drug-drug interactions between triazole antifungal agents used to treat invasive aspergillosis and immunosuppressants metabolized by cytochrome P450 3A4. Transpl Infect Dis. 2017;19(5):1–11.
- Ashley ED. Antifungal drugs: special problems treating Central nervous system infections. J. Fungi. 2019;5(4):1–8.
- Mayer FL, Kronstad JW. Discovery of a novel antifungal agent in the pathogen box. mSphere. 2017;2(2):e00120-17. doi:10.1128/mSphere.00120-17.
- Jacobs SE, Zagaliotis P, Walsh TJ. “Novel antifungal agents in clinical trials [version 2 ; peer review : 2 approved],” 2022.
- Alfadil A, Alsamhan HA, Ali AS, et al. In vivo and in vitro antifungal activity of 2,3-Dimethylquinoxline. J Pharm Res Int. 2021;33:198–207.
- Elfadil A, Ali AS, Alrabia MW, et al. The wound healing potential of 2,3 dimethylquinoxaline hydrogel in rat excisional wound model. J Pharm Res Int. 2023;35(8):1–8. doi:10.9734/jpri/2023/v35i87339.
- Khedekar YB, Mojad AA, Malsane PN. Formulation, development, and evaluation of silymarin loaded topical gel for fungal infection. Ournal Adv Pharm. 2019;08(01):6–9.
- Cockerill FR, Wikler MA, Alder J, et al. Methods for dilution antimicrobial susceptibility tests for bacteria that grow aerobically; Approved Standard—ninth Ed. vol. 32 (2012). Available from: https://clsi.org/media/1928/m07ed11_sample.pdf. Access, 2002.
- Tan CM, Therien AG, Lu J, et al. Restoring Methicillin-resistant Staphylococcus aureus susceptibility to β-lactam antibiotics. Sci Transl Med. 2012;4(126):126ra35. doi:10.1126/scitranslmed.3003592.
- Mikkelsen K, Sirisarn W, Alharbi O, et al. The novel membrane-associated auxiliary factors AuxA and AuxB modulate β-lactam resistance in MRSA by stabilizing lipoteichoic acids. Int J Antimicrob Agents. 2021;57(3):106283. doi:10.1016/j.ijantimicag.2021.106283.
- Halawi MH, et al. Two weeks of chronic unpredictable stress are sufficient to produce oral candidiasis in balb/C mice. Asian J Microbiol Biotechnol Environ Sci. 2020;22(2):2020–2254.
- Takakura N, Sato Y, Ishibashi H, et al. A novel murine model of oral candidiasis with local symptoms characteristic of oral thrush. Microbiol Immunol. 2003;47(5):321–326. doi:10.1111/j.1348-0421.2003.tb03403.x.
- Fathy M, Nikaido T. In vivo modulation of iNOS pathway in hepatocellular carcinoma by nigella sativa. Environ Health Prev Med. 2013;18(5):377–385.
- Abdelgalil MM, Ammar YA, Elhag Ali GAM, et al. A novel of quinoxaline derivatives tagged with pyrrolidinyl scaffold as a new class of antimicrobial agents: design, synthesis, antimicrobial activity, and molecular docking simulation. J Mol Struct. 2023;1274:134443. doi:10.1016/j.molstruc.2022.134443.
- Pappas PG, Kauffman CA, Andes DR, et al. Clinical practice guideline for the management of candidiasis: 2016 update by the infectious diseases society of america. Clin Infect Dis. 2016;62(4):e1–e50. doi:10.1093/cid/civ933.
- Barchiesi F, Orsetti E, Osimani P, et al. Factors related to outcome of bloodstream infections due to Candida parapsilosis complex. BMC Infect Dis. 2016;16(1):1–7.
- Patil S, Rao RS, Majumdar B, et al. Clinical appearance of oral Candida infection and therapeutic strategies. Front Microbiol. 2015;6(Dec.):1391. doi:10.3389/fmicb.2015.01391.
- de Oliveira Santos GC, Vasconcelos CC, Lopes AJO, et al. Candida infections and therapeutic strategies: mechanisms of action for traditional and alternative agents. Front Microbiol. 2018;9(Jul.):1–23. doi:10.3389/fmicb.2018.01351.
- Charles JI, Minakiri IS. Evaluation of the antibacterial activity of Chromolaena odorata in wistar rats and its chemical characterization. Am Sci Res J Eng Technol Sci. 2018;42(1):111–129.
- Daina A, Michielin O, Zoete V. SwissADME: a free web tool to evaluate pharmacokinetics, drug-likeness and medicinal chemistry friendliness of small molecules. Sci Rep. 2017;7(1):42717. doi:10.1038/srep42717.
- Cheng G, Sa W, Cao C, et al. Quinoxaline 1,4-di-N-oxides: biological activities and mechanisms of actions. Front Pharmacol. 2016;7(MAR):64. doi:10.3389/fphar.2016.00064.
- Chacón-Vargas KF, Andrade-Ochoa S, Nogueda-Torres B, et al. Isopropyl quinoxaline-7-carboxylate 1,4-di-N-oxide derivatives induce regulated necrosis-like cell death on Leishmania (Leishmania) mexicana. Parasitol Res. 2018;117(1):45–58. doi:10.1007/s00436-017-5635-3.
- Gonzales PR, Pesesky MW, Bouley R, et al. Synergistic, collaterally sensitive β-lactam combinations suppress resistance in MRSA. Nat Chem Biol. vol2015;11(11):855–861. doi:10.1038/nchembio.1911.
- Hutchings M, Truman A, Wilkinson B. Antibiotics: past, present and future. Curr Opin Microbiol. 2019;51:72–80. doi:10.1016/j.mib.2019.10.008.
- Ashburn TT, Thor KB. Drug repositioning : identifying and developing new uses for existing drugs. Nat Rev Drug Discov. 2004;3(8):673–683.
- Coates AR, Halls G, Hu Y. Novel classes of antibiotics or more of the same? Br J Pharmacol. 2011;163(1):184–194. doi:10.1111/j.1476-5381.2011.01250.x.