Abstract
Plants of tomato (Solanum esculentum Mill. cv. Ibiza F1) are exposed to 0.25, 1, 2.5, 5 and 10 mM NO3 - or NO2 - through rooting medium during 7 days with the aim to study KNO2 - induces changes in biomass production and membrane lipid composition. Cultivated on the same doses of KNO3 plants serve as indicators. The accumulation of NO2 - increases with external KNO2 concentrations, and is considerably higher in roots than in primary leaves. Biomass production of the growing leaves and roots is strongly depressed at higher KNO2 levels, which is associated with a significant decrease in content of total lipids and changes in fatty acid composition. Total lipids are more decreased in roots than in primary leaves. Also, significant decreases in content of global lipid fractions are recorded in elevated nitrite-stressed plants in comparison with controls. The contents of lipid components decline in all plant organs under NO2 - treatment. However, amounts of phospholipid molecules are more affected in roots than in old leaves. Moreover, in all lipid classes, levels polyunsaturated acids decrease, and that of saturated (C16:0) one increase.
Résumé
Des plants de tomate (Solanum esculentum Mill. cv. Ibiza F1) sont cultivés sur milieu hydroponique contenant 0.25, 1, 2.5, 5 and 10 mM NO3 - ou NO2 - durant 7 jours dans le but d’étudier les modifications dans la production de biomasse et de la composition en lipides membranaires induites par KNO2 -. Les plants cultivés sur les mêmes doses en (KNO3) servent de témoins (KNO3). L’accumulation de NO2 - dans les tissus augmente avec l’accroissement de la concentration de KNO2 dans le milieu et elle est plus importante dans les racines que dans les feuilles. La production de biomasse des feuilles et des racines est fortement réduite aux doses élevées de KNO2. Cette réduction de biomasse est associée à une diminution significative de la teneur en lipides et des changements dans la composition en acides gras. Ainsi, les teneurs des fractions lipidiques globales enregistrent une baisse dans les plants stressés en comparaison avec les plants témoins. De la même façon, les teneurs des composants lipidiques diminuent dans les divers organes de la plante avec l’accroissement de la dose de NO2 -. Cependant, les molécules de phospholipides paraissent plus affectées dans les racines que dans les feuilles matures. De plus, dans toutes les classes lipidiques, les taux des acides polyinsaturés diminuent tandis que celui de l’acide saturé (C16:0) augmente.
Introduction
Nitrogen is essential for all plant and animal life. It is a basic constituent of proteins. The form of nitrogen within plants when consumed by animals has important effects on growth and reproduction. This element makes up 78% of atmosphere as gaseous molecular nitrogen, but most plants can use it only in fixed forms of nitrate and ammonium. Nitrate and nitrite are inorganic ions occurring naturally as part of the nitrogen cycle (Smith & Sharpley, Citation1990).
Nitrite in soils is possibly originated during nitrification or denitrification depending on the soil conditions or where certain pesticides, or sewage sludge have been used in agriculture (Shrestha et al., Citation2009; Goyal & Huffaker, Citation1984). A number of researchers have found that nitrite accumulates in the periphery of NH3, NH4OH, and urea bands in acid soils. These researchers conclude that nitrite accumulated because of a combination of high pH and high ammonium concentrations in the fertilizer band, which inhibit the activity of nitrobacter, responsible for oxidation of nitrite to nitrate in soils (Chapman & Liebig, Citation1952; Lee, Citation1979). An internal accumulation of nitrite (caused by an external increase, or by herbicide) is toxic to plants, leading to extensive morphological and metabolic changes (Hoque et al., Citation2008; Pecsvaradi & Zsoldos, Citation1996).
Decrease inhibitory effects of nitrite on both ion uptake and growth of seedlings are extremely documented (Peuke, Citation2010; Pecsvaradi & Zsoldos, Citation1996; Zsoldos et al., Citation1994). Therefore, elevated nitrite concentration was reported to cause an acidification of the chloroplast stroma, leading to decreases in chloroplastic enzyme activities and CO2 fixation, and then in photosynthesis (Shingles et al., Citation1996). It has also been shown the effects of NO2 in term of enzyme activities involved in nitrogen metabolism (Rowland-Bamford et al., Citation1989); including then variation in fluxes of amino-acids (N-metabolism) (Wingsle et al., Citation1987). A reduction in photorespiration with increasing NO2 concentrations, in carbon allocation, and chlorophyll fluorescence, was also recorded. (Ito et al., Citation1985; Shimazaki, Citation1988).
On the other hand, it has already been demonstrated that gaseous NO2 causes cellular plasmolysis caused by the breakdown of lipid membranes. Unsaturated lipids in monolayers readily bind molecules such as NO2 -. Direct peroxidation of fatty acids as a consequence of this attached NO2 has been studied extensively (Pryor & Lightsey, Citation1981; Malhotra & Khan, Citation1984). In addition, nitrite’s harmful effects on plants (Yamazaki & Sakihama, Citation2000) including, N-nitrosamine formation (Challis et al., Citation1982) direct mutation of DNA (Wink, et al., Citation1991), DNA single-strand breaks (Bittrich et al., Citation1993), and/or other oxidative reactions. Obviously, accumulation of nitrite in the cells is the key event to reveal the conditions which lead to production of NR-dependent toxic molecules (i.e. NO, O2 -, ONOO-) (Modolo et al., Citation2005; Yamasaki, Citation2000).
In this experiment, we investigated the effect of NO3 - alone and replaced by similar doses of NO2 - on production of dry matter, accumulation of nitrite, in relation to changes in membrane lipid composition, and membrane integrity in tomato leaves and roots at the vegetative stage.
Material and methods
Plant material and growth conditions
Tomato seeds (Solanum esculentum Mill. cv. Ibiza F1) were washed in distilled water and sterilized in 10% (v/v) H2O2 for 20 min. The seeds were next thoroughly washed with distilled water and germinated on moistened filter paper at 25 °C in the dark. Uniform seedlings were then transferred to continuously aerated nutrient solutions (pH 6.0) containing 0,5 mM MgSO4, 0,5 mM KH2PO4, 0,25 mM CaCl2, 1 mM KNO3, 100 μM Fe-K-EDTA, 30 μM H3BO3, 5 μM MnSO4, 1 μM CuSO4, 1 μM ZnSO4, and 1 μM (NH4)6Mo7O24. After an initial growth period of ten days, nitrogen was added to the nutrient solution in two form (KNO3 and KNO2) at various concentrations (0.25, 1, 2.5, 5, and 10 mM), to keep constant the nutrient composition and pH, solutions were renewed continuously.
Plants were grown and treated hydroponically with NO2 in a growth chamber (26 °C/20 °C and 70%/90% relative humidity day/night regime, 16 h photoperiod with a light irradiance of 150 μmol m-2 s-1 at the canopy level). After 7 days of nitrite (KNO2) treatment, plants were separated into leaves and roots. Roots were rapidly washed in distilled water, and all samples were either dried to constant weight at 70 °C or stored in liquid nitrogen for later analysis. The data are averages of six biological replicates.
Nitrite essays
The nitrite concentration was determined in fresh plant material (primary, secondary leaves, and roots) by diazotizing with sulphanilamide and coupling with NED dihydrochloride to form a coloured azo-dye that was measured colorimetrically (Sanderson & Cocking, Citation1964).
Lipids determination
Extraction of total lipids was performed using CHCL3-MeOH-H2O (1:1:1) (Bligh & Dyer, Citation1964). Lipids classes were sped on silica gel TLC plates 60 (MERCK) using the following solvent systems: CHCL3-Me2CO-MeOH-HOAc-H2O (10:4:2:2:1) (Trémolière & Lepage, 1971) and petrol-Et2O-HOAc (70:30:0.4) (Mangold, Citation1964). Lipid spots were located by brief exposure to I2 vapour. Specific stains for phospholipids and galactolipids identified by comparison with lipid standards and lipid classes. Fatty acids from total lipids and lipid classes were transmethylated with MeOH-BF3 (Metcalfe et al., Citation1966). FAMES were analysed by FID-GC on metal column (1.8 m × 3 mm i. d.) filled with GP 3% SP-2300. The column was maintained isothermally at 190 °C. Carrier gas was N2 (20 ml min-1), and the operating temperature, in the injector and detector were respectively, 210 °C and 240 °C. For measuring the amounts of fatty amounts of fatty acids, 17:0 was added as int. standard. Calculation of fatty acid quantities was obtained using an integrator. The least significant differences (LSD) at 5% were used for mean comparison.
Results
Growth
The increase of KNO3 concentrations in the medium enhances the dry weight production of tomato plants (Fig. ). Whereas, the effect of elevated doses of nitrite on growth appears with 2,5 mM. The presence of 10 mM of NO2 in the medium shows a dramatic effect on dry biomass production of F1 and R, which falls about 64% and 152% respectively, compared to this obtained with 1 mM KNO2.
Fig. 1 Effects of increasing nitrite and nitrate concentration on dry weight (DW) of primary leaves (F1) and roots (R) of tomato during 7 days. Values are means ± E.S (n = 6). SE is indicated by bars when larger than symbol. The least significant differences (LSD) at 5% were used for mean comparison.Fig. 1. Matière sèche des feuilles matures (F1) et des racines (R) de tomate exposée à des doses variables de nitrate ou de nitrite pendant 7 jours. Chaque point est la moyenne de 6 répétitions ± E.S, intervalle de confiance calculé au seuil de probabilité 95%
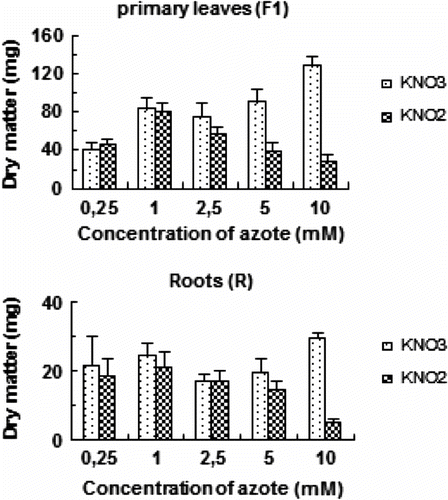
Changes in NO2 contents
As shown (Fig. ), in the presence of nitrite in the nutrient solution, different organs of tomato plants accumulate sizeable amounts of NO2. In both roots and primary leaves (F1), the concentration of this ion increases gradually with improved doses of KNO2 in the medium. At 10 mM about 70% of total plant content of NO2 is found in the roots.
Fig. 2 Variation of nitrite content of primary leaves (F1) and roots (R) of tomato seedlings after 7 days exposure to increased concentration of KNO2 in the culture medium. Values are means ± E.S (n = 6). SE is indicated by bars when larger than symbol. The least significant differences (LSD) at 5% were used for mean comparison. Fig. 2. Variation de la teneur en nitrite des feuilles matures (F1) et des racines (R) des plants de tomate exposés pendant 7 jours à des concentrations élevées de KNO2 dans le milieu de culture. Chaque point est la moyenne de 6 répétitions ± E.S, intervalle de confiance calculé au seuil de probabilité 95%.
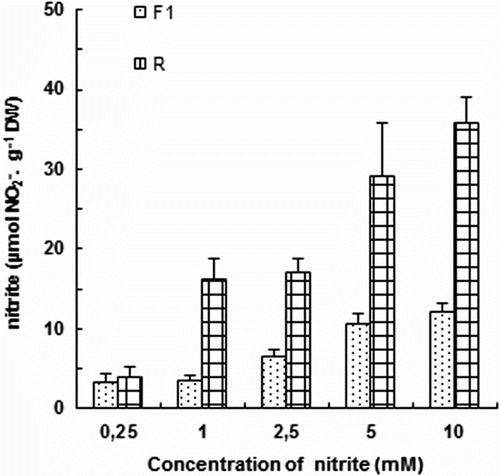
Changes in lipid contents
Total lipids
Besides, the amount of total lipids, which corresponds to the total fatty acids, is lower in treated plants than in control ones, and is remarkably dependent on nitrogen doses in the medium. The results (Fig. ) show a decrease in leaf lipids of 34% in primary leaves with elevated levels of 10 mM KNO3 and KNO2 respectively. In roots, there is however a marked drop in total lipids and this ranged from 43 to 66% with 5 and 10 mM KNO3 and KNO2 respectively (Fig. ).
Fig. 3 Variation of total lipid content in primary leaves (F1) and roots (R) of tomato seedlings after 7 days exposure to increased concentration of KNO2 or KNO3 in the culture medium. Values are means ± E.S (n = 6). SE is indicated by bars when larger than symbol. The least significant differences (LSD) at 5% were used for mean comparison. Fig. 3. Variation de la teneur en lipides totaux des feuilles matures (F1) et des racines (R) des plants de tomate exposés pendant 7 jours à des concentrations élevées de KNO2 ou de KNO3 dans le milieu de culture. Chaque point est la moyenne de 6 répétitions ± E.S, intervalle de confiance calculé au seuil de probabilité 95%.
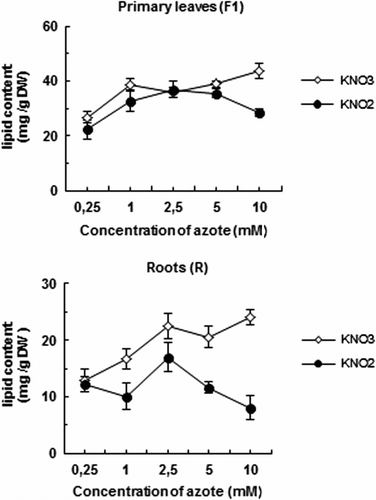
Lipid composition
In control plants, significant differences in the lipid composition of primary leaves and roots are observed (Table ). In leaves, glycolipids are the major constituent lipid classes, accounting for 55% of the total lipids. The root lipids are characterized by a high level of phospholipids, about 50% of the total lipids, followed by neutral lipids and glycolipids (Table ). Also, it should be noted that excess of NO2, has a more pronounced effect on roots total lipid content and fatty acid composition than in primary leaves (Table ). Thus, the glycolipids, phospholipids and the neutral lipids content in treated root plants shows only 29, 32 and 26% of these fatty acid than in control ones. In primary leaves (F1), these molecules form 61, 80 and 95% respectively (Table ).
Table 1. Lipid composition (mg. g-1 MS) in primary leaves (F1) and roots (R) of tomato seedlings after 7 days exposure to increased concentration of KNO2 or KNO3 in the culture medium. (Number between brackets represents the percent% of lipid classes). Values are means ± E.S (n = 6). The least significant differences (LSD) at 5% were used for mean comparison. Tableau 1. Composition en lipides (mg. g-1 MS) des feuilles matures (F1) et des racines (R) des plants de tomate exposés pendant 7 jours à des concentrations élevées de KNO2 ou de KNO3 dans le milieu de culture. (Les valeurs entre parenthèses représentent le pourcentage en classe lipidique). Chaque point est la moyenne de 6 répétitions ± E.S, intervalle de confiance calculé au seuil de probabilité 95%.
Moreover, in this lipid class the proportion of monogalactosyl diacylglycerol (MGDG) is higher than that of digalactosyl diacylglycerol (DGDG) (ca. 24% and 22% of total lipids, respectively in primary leaves). It is also interesting to note, that the percentage of MGDG in total lipids of roots is lower than that of DGDG (ca. 4% and 7% of total lipids, respectively) (Fig. ). Moreover, the MGDG/DGDG ratio changes for 1,08 in control primary leaves to 0,891 in treated ones. In roots the ratio vary from 0,66 to 0,59 (Fig. ).
Fig. 4 Effects of increasing nitrite concentration on glycolipids molecule content (MGDG, monogalactosyldiacylglycerol; DGDG, digalactosyldiacylglycerol; SL, sulfolipids) in primary leaves (F) and roots (R) of tomato seedlings after 7 days exposure to increased concentration of KNO2 or KNO3 in the culture medium. Values are means ± E.S (n = 6). SE is indicated by bars when larger than symbol. The least significant differences (LSD) at 5% were used for mean comparison. Fig. 4. Effets des concentrations élevées de nitrite sur la teneur des molécules de glycolipides (MGDG, monogalactosyldiacylglycerol; DGDG, digalactosyldiacylglycerol; SL, sulfolipids) des feuilles matures (F1) et des racines (R) des plants de tomate exposés pendant 7 jours à des concentrations élevées de KNO2 ou de KNO3 dans le milieu de culture. Chaque point est la moyenne de 6 répétitions ± SE, intervalle de confiance calculé au seuil de probabilité 95%.
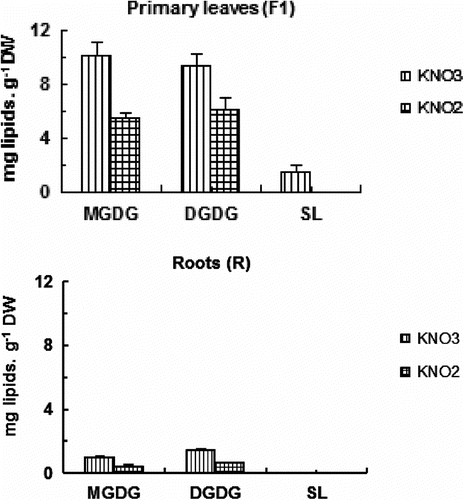
In the other hands, the most abundant phospholipids in both leaves and root tissues are phosphotidylcholine (PC) and phosphotidylglycerol (PG) (Fig. ). Concerning the phospholipids content, a substantial decrease is showed in roots (63% of control), compared to primary leaves content (40% of control); and this results show a decline in the content of all phospholipids molecules including phosphatidylcholine (PC), phosphatidylglycerol (PG), phosphatidylethanolamine (PE), phosphatidic acid (PA), and phosphatidylinositol (PI) (Fig. ).
Fig. 5 Effects of increasing nitrite concentration on phospholipids molecule content (phosphatidylcholine, PC; phosphatidylglycerol, PG; phosphatidyléthalonamine PE; phosphatidic acid, PA; phosphatidylinositol, PI) of tomato seedlings after 7 days exposure to increased concentration of KNO2 or KNO3 in the culture medium. Values are means ± E.S (n = 6). SE is indicated by bars when larger than symbol. The least significant differences (LSD) at 5% were used for mean comparison. Fig. 5. Effets des concentrations élevées de nitrite sur la teneur des molécules de phospholipides (phosphatidylcholine, PC; phosphatidylglycerol, PG; phosphatidyléthalonamine PE; phosphatidic acid, PA; phosphatidylinositol, PI) des feuilles mâtures (F1) et des racines (R) des plants de tomate exposés pendant 7 jours à des concentrations élevées de KNO2 ou de KNO3 dans le milieu de culture. Chaque point est la moyenne de 6 répétitions ± SE, intervalle de confiance calculé au seuil de probabilité 95%.
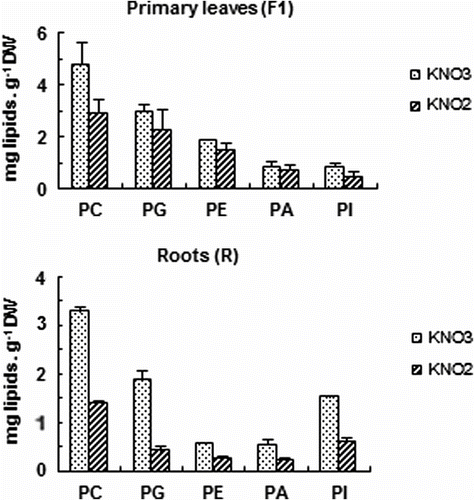
Fatty acid species
Some fatty acid species, palmitic (16:0), linoleic (18:2) and linolenic (18:3) acids are commonly higher in root and leaf lipids, and only differed in their relative proportions (Table a,b). The contribution of 18:3 in the leaves is significantly higher (ca. 47% in primary leaves of total fatty acids) and that of 16:0 and 18:2 are lower (ca. 21 and 14% in primary leaves of total fatty acids, respectively), and vice versa in the root lipids (ca. 12% of 18:3; and 28%, and 48% of 16:0, and 18:2) (Table a,b). Also present are palmitoleic (16:1), hexadecatrienoic (16:3), stearic (18:0) and oleic (18:1) acids. Furthermore, the fatty acid composition of leaf lipids is also dominated by the presence of an appreciable content of 16:3, approximately 12% (F1) of the total fatty acids. This profile is generally characteristic of the so-called 16:3 plants and is in agreement with previous work on Solanum esculuntum (Douce & Joyard Citation1980; Whitaker, Citation1986).
Table 2. a. Total fatty acid composition in primary leaves (F1) of tomato seedlings after 7 days exposure to increased concentration of KNO2 or KNO3 in the culture medium. (Results expressed in % of total fatty acid). Values are means ± E.S (n = 6). The least significant differences (LSD) at 5% were used for mean comparison. Tableau 2. a. Composition en acides gras totaux des feuilles mâtures (F1) des plants de tomate exposés pendant 7 jours à des concentrations élevées de KNO2 ou de KNO3 dans le milieu de culture. (Les résultats sont exprimés en % des acides gras totaux). Chaque point est la moyenne de 6 répétitions ± SE, intervalle de confiance calculé au seuil de probabilité 95%.
Table 2. b. Total fatty acid composition in roots (R) of tomato seedlings after 7 days exposure to increased concentration of KNO2 or KNO3 in the culture medium. (Results expressed in % of total fatty acid). Values are means ± E.S (n = 6). The least significant differences (LSD) at 5% were used for mean comparison. Tableau 2. b. Composition en acides gras totaux des racines (R) des plants de tomate exposés pendant 7 jours à des concentrations élevées de KNO2 ou de KNO3 dans le milieu de culture. (Les résultats sont exprimés en % des acides gras totaux). Chaque point est la moyenne de 6 répétitions ± SE, intervalle de confiance calculé au seuil de probabilité 95%.
The fatty acids most subject to variation are palmitic (16:0), and linolenic (18:3) acids. In primary leaves of plants with high NO2 doses, the percentage of 18:3, the abundant fatty acid, decrease and that of 16:0 increase. In addition, with 10 mM KNO2 a lowering of 25% in 16:3 is recorded in primary leaves (Table a, b). However, in roots the remarkable impoverishment in both 18:2 and 18:3 (28% and 52%, respectively), is accompanied by enrichment in 16:0 (44%). In is noteworthy that the C16/C18 ratio is increased in leaves and roots of treated tomato plants (Table a, b).
Fatty acid composition
The composition of fatty acid differs considerably between organs and categories of lipids (Tables a, b). At highest doses of NO2, glycolipids in primary leaves (F1) show a decrease percentage of 18:3 for MGDG, DGDG, and SL (8%, 17%, and 11%, respectively of control). The decrease is accompanied by an increase in proportion of 16:0 (23% in F1). Further, within MGDG of treated leaves the drop of 18:3 content results in an increase of 16:0 and 18:0. However, in the MGDG of treated roots the 16:1, and 18:2 proportions is reduced. In neutral lipids, the increase in 16:0 percentages is accompanied with a diminution in 18:3 in leaves, but 18:2 show a sharp increase in primary leaves, and 18:0 and 18:2 show a little increase in roots.
Table 3. a. Fatty acid composition of glycolipid and phospholipid molecules of primary leaves (F1) of tomato exposed during 7 days to 10 mM KNO2 or KNO3 in the culture medium. Values are means ± E.S (n = 6). The least significant differences (LSD) at 5% were used for mean comparison. Tableau 3. a. Composition des acides gras; glycolipides et phospholipides des feuilles matures (F1) des plants de tomate exposés pendant 7 jours à 10 mM KNO2 ou de KNO3 dans le milieu de culture. Chaque point est la moyenne de 6 répétitions ± SE, intervalle de confiance calculé au seuil de probabilité 95%.
Table 3. b. Fatty acid composition of glycolipid and phospholipid molecules of roots (R) of tomato exposed during 7 days to 10 mM KNO2 or KNO3 in the culture medium. Values are means ± E.S (n = 6). The least significant differences (LSD) at 5% were used for mean comparison. Tableau 3. b. Composition des acides gras; glycolipides et phospholipides des racines (R) des plants de tomate exposés pendant 7 jours à 10 mM KNO2 ou de KNO3 dans le milieu de culture. Chaque point est la moyenne de 6 répétitions ± SE, intervalle de confiance calculé au seuil de probabilité 95%.
The fatty acid composition of phospholipids is also changed by nitrite. In fact, within both PC and PG of leaves high level of NO2 results in a decrease of 18:2 and 18:3 proportions with an increase in 16:0 (Tables a). However, in PC of stressed roots, the decline of 18:3,18:2 is accompanied by an increase of 16:3 and 16:0, similar response is observed for PG, except the increase of percentage of 16:1 and the stability of 18:2 ones (Tables b).
Discussion
The oxides of nitrogen, NO3 and NO2, can cause reductions in growth but rarely visible injury (Wellburn, Citation1990). Recent work established a gradual increase of nitrite accumulation in soil layers. Such a nitrite accumulation in the topsoil layer may give rise to nitrite toxicity to plants and become a threat to the agro-ecosystem (Singh et al., Citation2007). In our study, significant depression of leaves and roots dry weight production in treated hydroponic tomato plant was observed (Fig. ), and this effect varied with the concentration of the potassium nitrite added to the nutrient solution.
Numerous authors have shown that plants can absorb and assimilate NO2 -. A complete reduction and assimilation pathway for NO2 - seems to be active in the roots of most plants (Yoneyama et al., Citation1980). Even though, little or no NO2 - may be exported from the roots to shoots (Yoneyama et al., Citation1980). It is noteworthy that in some seedlings the inhibitory effects of nitrite are different for the roots and the shoots. The roots are extremely sensitive to nitrite treatments (Wang et al., Citation2007; Zsoldos, 1994). Similarly, in our experiments tomato root dry weight was extremely reduced at high dose of NO2, this here coincides with a considerable accumulation of this salt, especially in this organ (Fig. ).
Our results also exhibit that plants exposed to high concentrations of NO2 usually show a sharp decline of content of all lipid classes. Again, the quantification of different lipid classes isolated from leaves and roots of this tomato plant showed that total lipid content and the lipid composition were affected by nitrite stress (Fig. , Table ). Previous studies have shown that lipid peroxidation degree, assessed by MDA (Malonyldialdehyde) production, increased with KNO2 concentrations in leaves and roots. The level of MDA was stimulated by the application of 5 and 10 mM KNO2 in F1, F2 and roots (Ezzine & Ghorbel, Citation2006).
Indeed, under prominent doses of nitrite, we noted a significant decrease of lipid molecules and noticeable alterations of fatty acid composition in all lipid classes (Fig. and , Table a, b). Since, MGDG has been documented to be a major chloroplast membrane galactolipids (Lichenthaler & Park, Citation1963; Bishop, Citation1984) and as thylakoid membrane galactolipids predominantly originate from the chloroplast envelope (Joyard et al., Citation1980; Rawyler et al., Citation1995). The content of this species as compared to DGDG was decreased in these stressed-tomato leaves, ensuing a decline of the MGDG/DGDG ratio, might be caused by a high galactolipase activity (Gigon, 2004), which attack preferentially MGDG. Moreover, in thylakoid membranes, the curved sections which are formed by the specifically located unsaturated C=C bonds in chloroplast galactolipids such as MGDG, play a pivotal role in chloroplast electron transfer. Furthermore, these C=C bonds are especially prone to oxy-free radical attack (Carruthers & Melchior, Citation1986). Unsaturated lipids in monolayers readily bind molecules like NO2 (Felmeister et al., Citation1970) and direct peroxidation of fatty acids as a consequence of this attached NO2 has been studied extensively (Rowland & Gause, Citation1971; Pryor & Lightsey, Citation1981).
Also, we have found that initial levels of various phospholipids decrease in the following order: PC, PG, PE, PA and PI. This decrease is more pronounced in roots than in leaves (Fig. ). Such species predominate in most cell membranes, with the exception of thylakoid membranes and the chloroplast envelope, where these phospholipids are largely replaced by galactolipids in which the main phospholipids are PG (Mazliak, Citation1977), which is most commonly found in plastids, but can also be found at low level in the plasma membrane (Yoshiba, & Uemura, Citation1984). PC and PE are the main components of the plasmalemma, mitochondrial membranes, nuclear membranes, tonoplast, peroxisomes and glyoxisomes. As PC plays an important role in lipid metabolism (Mazliak, Citation1977). It has in fact been suggested that PC is the direct substrate in the desaturation of oleic in the endoplastic reticulum (Stobart et al., Citation1983; Murphy et al., Citation1984) and is the diacylglycerol donor for biosynthesis of tri-unsaturated galactolipids in the chloroplasts (Drapier et al., Citation1982; Oursel et al., Citation1987). The decrease of PC levels was more accentual in roots than in F1 (Fig. ). In general, PC level varied little, this confirms the major role of these phospholipids in survival. In our earlier findings, proportion decreases of these entities might be verified by some stimulation of the antioxidant enzymes. In fact, peroxidase activity of F1 was increased less by NO2 - stress than roots (Ezzine & Ghorbel, Citation2006). According to (Sakihama et al., Citation2003), nitrogen dioxide radical (NO2) rather than ONOO- produced by a gaiacol peroxidase is the intermediate which affect plant secondary metabolisms. (Paliyath et al., Citation1987) have shown that phospholipids degradation involves three enzymes: phospholipase D, phosphotidic acid phosphatase and lipolytic acyl hydrolase.
On the other hand, we suppose that the decrease in unsaturated fatty acid levels, specifically those of 18:2 and 18:3 observed here in the lipid molecular species of treated tomato plants (Table , table a, b), might be related to direct reaction of oxygen free radicals with unsaturated lipids. Otherwise, it would seem that glycolipids are deacylated and the resulting C18:3 are attacked by lipoxygenase (Draper, Citation1969). On the other hand, we found an accumulation of 16:0 rather than 18:0 suggest an alteration of fatty acid synthase activity. Thus, the C16/C18 ratio was modified. Two types of reactions take place within fatty acids. Attachment of NO2 to a double bond may cause a cis to trans isomerization or removal of hydrogen from methylene groups. Both processes may also initiate lipid peroxidation as well as cause changes in the surface properties of monolayers (Wellburn, Citation1990).
Conclusion
Summing up, lipid peroxidation is the system most easily ascribed to oxidative damage as well as the most frequently measured and leads to impairment of membrane function. There are stronger indications that atmospheric NO2 inhibits lipid biosynthesis rather than causing damage to existing lipids in membranes. Thus, nitrite is a macro-nutrient, and each addition of this nitrogen form can result in a physiological response; changes in growth inhibition, and visible injury via lipid breakdown and cellular plasmolysis at higher levels of NO2. At lower concentrations of this nutriment lipid biosynthesis may dominate, rather than damage of existing lipids.
References
- Bishop , D.G. 1984 . “ The structural role of lipids in the chloroplast thylakoid membrane ” . In structure function and metabolism of plant lipids , Edited by: Siegenthaler , P. A. and Eichenberger , W. 383 – 388 . Amsterdam : Elsevier Science Publishers .
- Bittrich , H. , Matzig , A.K. and Kraker , I & K.E. 1993 . Appel, NO2-induced DNA single strand breaks are inhibited by antioxidative vitamins in V79 cells . Chem. Biol. Interact , 86 : 199 – 221 .
- Bligh , E.G. and Dyer , W.J. 1964 . A rapid method of total lipid extraction and purification . Can. J. Biochem , 226 : 497 – 509 .
- Carruthers , A. and Melchior , D.L. 1986 . How bilayer lipids effects membrane protein activity . Trends Biochem. Sci , 11 : 331 – 335 .
- Challis, B.C., D.E.G. Shucker, G.H. Fine, E.G. Goff, and G.A. Hoffman., 1982. Amine nitration and nitrosation by aqueous nitrogen dioxide. In: N-nitroso compounds: occurrence and biological effects (Bartsch H, O’Neill IK, castegnaro M, Okada M, eds), IARC Scientific Publication no, 41. Lyon: International Agency for Research on, Cancer, pp. 11–20.
- Chapman , H.D. and Liebig , G.F.J. 1952 . Field and laboratory studies of nitrite accumulation in soils . Soil Sci. Soc. Am. Proc , 16 : 276 – 282 .
- Douce R. and J. Joyard., 1980. Plant galactolipids. In the Biochemistry pf Plants. A comprehensive treatment (Stumps, P. K. and E.E. conn, eds.), Vol. 4: lipids: structure and fonction, Academic Press, New York. London, pp. 321–357.
- Draper , S.R. 1969 . Lipid changes in senescing cucumber cotyledons . Phytochemistry , 8 : 1641
- Drapier , D. , Dubacq , J.P. , Trémolières , A. and Mazliak , P. 1982 . Cooperative pathway for lipid biosynthesis in young pea leaves: Oleate exportation and subsequent integration into complex lipids of added microsomes . Plant. Cell. Physiol , 23 : 125 – 133 .
- Ezzine , M. and Ghorbel , M.H. 2006 . Physiological and biochemical responses resulting from nitrite accumulation in tomato (Lycopersicon esculentum Mill. cv. Ibiza F1) . Journal of Plant Physiol , 163 : 1032 – 1039 .
- Felmeister , A.M. , Amarat , M. and Weiner , N.D. 1970 . Interactions of gaseous air polluants within egg lecithin and phosphatidyl ethanolamine monomolecular films . Atmospheric Environment , 4 : 311 – 316 .
- Gigon , A. , Matos , A.R. , Laffray , D. , Zuily-Fodil , Y. and Pham-Thi , A. 2004 . Effect of Drought Stress on Lipid Metabolism in the Leaves of Arabidopsis thaliana (Ecotype Columbia) . Annals of Botany , 94 ( 3 ) : 345 – 351 .
- Goyal, H.H., and R.C. Huffaker. 1984. Nitrogen toxicity in plants. In: Nitrogen in crop production (by R.D. Hauck)., pp. 97–118.
- Hoque , M. , Ajwa , M. and Smith , H.A.R. 2008 . Nitrite and ammonium toxicity on lettuce grown under hydroponics . Communications in Soil Science and Plant Analysis , 39 ( 1–2 ) : 207 – 216 .
- Ito , O. , Okano , K. , Kuroiwa , M. and Totsuka , T. 1985 . Effects of NO2 and NO3 alone or in combinaison on kidney bean (Phaseolus vulgaris L.): growth, partitioning of assimilates and root activities . J. Exp. Bot , 36 : 652 – 662 .
- Joyard , J. , Douce , R. , Siebertz , H.P. and Heinz , E. 1980 . Distribution of radioactive lipids between envelopes and thylakoids from chloroplasts labelled in vivo . Eur. J. Biochem , 108 : 171 – 176 .
- Lee , R.B. 1979 . The effect of nitrite on root growth of barley and maize . New Phytol , 83 : 615 – 622 .
- Lichenthaler , H.K. and Park , R.B. 1963 . Chemical composition of chloroplast lamellae from spinach . Nature , 198 : 1070 – 1072 .
- Malhotra , S.S. and Khan , A.A. 1984 . “ Biochemical and physiological impact of major pollutant ” . In Air Pollution and Plant Life , Edited by: Treshow , M. 113 – 157 . Chichester : John Wiley & Sons .
- Mangold , H.K. 1964 . Thin layer chromatography of lipids . J. Am. Oil. Chem. Soc , 41 : 762 – 773 .
- Mazliak , P. 1977 . “ Glyco- and phospholipids of biomembranes in higher plants ” . In lipids and lipid polymers in higher plants , Edited by: Tevini , M. and Lichtenthaler , H. K. 48 – 74 . Berlin : Springer-Verlag .
- Metcalfe , L.D. , Scmitz , A.A. and Pelka , J.R. 1966 . Rapid preparation of fatty acid esters from lipids for gas-chromatographic analysis . Anal. Chem , 38 : 514 – 515 .
- Modolo , L.V. , Augusto , O. , Almeida , I.M. , Magalhaes , J.R. and Salgado , I. 2005 . Nitrite as the major source of nitric oxide production by Arabidopsis thaliana in response to Pseudomonas syringae . FEBS Lett , 579 : 3814 – 3820 .
- Murphy , D.J. , Latzko , E. , Woodrow , I.E. and Mukhersee , K.D. 1984 . “ The oleate desaturase of pea leaf microsomes ” . In structure function and metabolism of plant lipids , Edited by: Siegenthaler , P. A. and Eichenberger , W. 45 – 50 . Amsterdam : Elsevier Science Publishers .
- Oursel , A. , Escoffier , A. , Kader , J.C. , Dubacq , J.P. and Trémolières , A. 1987 . Last step in the cooperative pathway for galactolipid synthesis in spinach leaves: formation of monogalactosyldiacylglycerol with C18 polyunsaturated acyl groups at both carbon atoms of the glycerol . FEBS Lett , 219 : 393 – 399 .
- Paliyath , G. , Lynch , D.V. and Thompson , J.E. 1987 . Regulation of membrane phospholipid catabolism in senescing carnation flowers . Physiol. Plant , 71 : 503 – 511 .
- Pecsvaradi , A. and Zsoldos , F. 1996 . Nitrate reductase and nitrite reductase activity in nitrite-and chloratestressed rice seedling . Plant Physiol. Biochem , 34 ( 2 ) : 659 – 663 .
- Peuke , A.D. 2010 . Correlations in concentrations, xylem and phloem flows, and partitioning of elements and ions in intact plants. A summary and statistical re-evaluation of modelling experiments in Ricinus communis . Journal of Experimental Botany , 61 ( 3 ) : 635 – 655 .
- Pryor , W.A. and Lightsey , J.W. 1981 . Mechanisms of nitrogen dioxide reactions: initiation of lipid peroxidation and the production of nitrous acid . Science , 214 : 435 – 437 .
- Rawyler , A. , Meylan-Bettex , M. and Siegenthaler , P.A. 1995 . Galactolipid export from envelope to thylakoid membranes in intact chloroplasts II. General process with a key role for the envelope in the establishment of lipid asymmetry in thylakoid membranes . Biochim. Biophys. Acta , 1233 : 123 – 133 .
- Rowland , J.R. and Gause , E.M. 1971 . Reactions of NO2 with blood and lung components . Archives of International Medicine , 128 : 94 – 100 .
- Rowland-Bamford , A.J. , Lea , P.J. and Wellburn , A.R. 1989 . NO2 flux into leaves of nitrate reductase-deficient barley mutants and corresponding changes in nitrate reductase activity . Environ. Exp. Bot , 29 : 439 – 444 .
- Sakihama , Y. , Tamaki , R. , Shimoji , H. , Ichiba , T. , Fukushi , Y. and Tahara , S. 2003 . Enzymatic nitration of phytophenolics: evidence for peroxynitrite-independent nitration of plant secondary metabolisms . FEBS Lett , 533 : 377 – 80 .
- Sanderson , G.W. and Cocking , E.C. 1964 . Enzymic assimilation of nitrate in tomato plants. I: Reduction of nitrate to nitrite . Plant Physiol , 39 : 416 – 422 .
- Shimazaki, K.I. 1988. Thylakoid membrane reactions to air polluants. In Air Pollution and Plant Metabolism. Proceedings of the 2nd International Symposium on Air Pollution and Plant Metabolism. Munich, 1987 (Ed. By S. Schulte-Hostede, N.M.).
- Shingles , R. , Michael , H.R. and Richard , E.M. 1996 . Nitrite transport in chloroplast inner envelope vesicles. 1. Direct measurement of proton-linked transport . Plant Physiol. , 11 ( 2 ) : 1375 – 1381 .
- Shrestha , J. , Rich , J.J. , Ehrenfeld , J.G. and Jaffe , P.R. 2009 . Oxidation of ammonium to nitrite under iron-reducing conditions in wetland soils: laboratory, field demonstrations, and push-pull rate determination . Soil Science , 174 ( 3 ) : 156 – 164 .
- Singh , M. , Pabbi , S. , Bhattacharya , A.K. and Singh , A.K. 2007 . Nitrite accumulation in coastal clay soil of India under inadequate subsurface drainage . Agricultural water management , 91 ( 1–3 ) : 78 – 85 .
- Smith , S.J. and Sharpley , A.N. 1990 . Soil nitrogen mineralisation in the presence of surface and incorporated crop residues . Agron. J , 82 : 112 – 116 .
- Stobart , A. , Stymne , S. and Glad , G. 1983 . The role of the acyl CoA pool in the synthesis of polyunsaturated 18-carbon fatty acids and triacylglycerol production in the microsomes of developing safflowers seeds . Biochim. Biophys. Acta , 752 : 198 – 208 .
- Trémolières , A. and Lepage , M. 1971 . Changes in lipid composition during greening of etiolated pea seedlings . Plant Physiol , 47 : 329
- Wang , R. , Xing , X. and Crawford , N. 2007 . Nitrite acts as a transcriptome signal at micromolar concentrations in Arabidopsis roots . Plant Physiol , 145 ( 4 ) : 1735 – 1745 .
- Wellburn , A.R. 1990 . Why are atmospheric oxides of nitrogen usually phytotoxic and not alternative fertilizers? . New Phytol , 115 : 395 – 429 .
- Whitaker , B.D. 1986 . Fatty-acid composition of polar lipids in fruit and leaf chloroplasts of “16:3” and “18:3” plant species . Planta , 169 : 313 – 319 .
- Wingsle , G.N. , Tlandmark , T. and Ericsson , A. 1987 . Induction of nitrate reductase in needles of Scots pine seedlings by NOx and NO3 - . Physiologia plantarum , 70 : 399 – 403 .
- Wink , D.A. , Kasprzak , K.S. , Maragos , C.M. , Elespuru , R.K. , Misra , M. , Dunams , T.M. , Cebula , T.A. , Koch , W.H. , Andrews , A.W. , Allen , J.S. and Keefer , L.K. 1991 . DNA deaminating ability and genotoxicity of nitric oxide and its progenitors . Science , 254 : 1001 – 1003 .
- Yamasaki , H. 2000 . Nitrite-dependent nitric oxide production pathway: implications for involvement of active nitrogen species in photoinhibition in vivo . Phil. Trans. R. Soc. Lond , 355 : 1477 – 1488 .
- Yamazaki , H. and Sakihama , Y. 2000 . Simultaneous production of nitric oxide and peroxynitrite by plant nitrate reductase: in vitro evidence for the NR-dependent formation of active nitrogen species . Febs Lett , 468 : 89 – 92 .
- Yoneyama , T. , Iwata , E. and Yazaki , J. 1980 . Nitrite utilization in the roots of higher plants . Soil Sci. Plant. Nutr , 26 : 9 – 23 .
- Yoshiba , S. and Uemura , M. 1984 . Protein and lipid compositions of isolated plasma membranes from orchard grass (Dactylis glomerata L.) and changes during cold acclimation . Plant. Physiol , 75 : 31 – 37 .
- Zsoldos , F. , Vashegyi , A. and Pecsvaradi , A. 1994 . Effects of pH and nitrite on potassium uptake and growth of rice seedlings . J. Plant Physiol , 144 : 358 – 361 .