Abstract
Biological invasions are one of the world’s leading causes of biodiversity loss. Islands are particularly good models for studying the impact of invasive species. Bagaud island (58 ha), which is part of Port-Cros National Park (southeastern France), is currently suffering an invasion of Carpobrotus. A restoration project has been established with the aim of eradicating these species to protect native ecosystems. The objective of the present study was to examine the plant composition of the local vegetation and seed bank (in litter and soil) focusing on (i) Carpobrotus patches and (ii) native plant communities, with the ultimate goal of determining whether Carpobrotus removal is followed by native plant community colonization. A further objective of this study was to help identify the most effective protocol for adequate ecological restoration. Native plant communities found around Carpobrotus patches can vary depending on whether the patch is located on the coast or inland; we can therefore expect a variety of plant communities to form following Carpobrotus removal. Even though Carpobrotus litter can frequently contain seeds of native species, such Atriplex prostrata, Frankenia sp. and Sonchus asper, it also contained many more Carpobrotus seeds (77.6%). Therefore, it is important to remove Carpobrotus litter to prevent large numbers of Carpobrotus seeds from recolonizing after eradication. We conclude that the most effective ecological restoration protocol consists of the following: (i) the removal of living Carpobrotus plants and (ii) the removal of Carpobrotus litter. This protocol can be made even more effective by following it up in the near and mid-term by periodic and thorough checks for Carpobrotus reinvasion.
Introduction
Among ecological concerns that involve global changes (climate change, land-use changes, modified disturbance regime), biological invasions (Richardson et al. Citation2000; Milbau and Stout Citation2008) pose a major threat to native biodiversity. Drastic increases in human activity involving national and international commercial exchanges have greatly increased species introductions and invasions (Elton Citation1958; Vitousek et al. Citation1996; McKinney and Lockwood Citation1999; Mack et al. Citation2000; Mooney and Cleland Citation2001; Pascal Citation2009; Vilà et al. Citation2011). Invasive species are known to modify the composition and functioning of native ecosystems (D’Antonio and Haubensak Citation1998), and to cause the regression or local extinction of native species (see Vitousek et al. Citation1996; Mack et al. Citation2000; Vilà et al. Citation2011). Moreover, they show important public health impacts and various economic ramifications in agriculture, grazing, fisheries, tourism and recreation; they also imply obvious expenses for local ecological remediation efforts (e.g. Born, Rauschmayer, and Bräuer Citation2004).
Within this context, island ecosystems are good models for studying biological invasions. Because islands are geographically isolated, have low species richness with high endemism, and have simplified food webs (Nunn Citation1994; Cronk Citation1997; Whittaker Citation1998; Denslow Citation2001; Drake et al. Citation2002), their ecosystems are particularly vulnerable to biological invasions (Sax, Gaines, and Brown Citation2002; Sax and Gaines Citation2008), and subject to the local extinction of native species (Ricketts et al. Citation2005), except for some introduced birds (see Sol Citation2000; Cassey Citation2003). The thousands of islands and islets in the Mediterranean Basin, the third richest plant diversity hotspot in the world (Myers et al. Citation2000; Médail and Myers Citation2004), face multiple biological invasions because of ancient massive and frequent species introductions (Brunel et al. Citation2010). Carpobrotus edulis (L.) N. E. Br. and Carpobrotus acinaciformis (L.) L. Bolus native from South Africa, are considered major invasive plants in the Mediterranean Basin (Hulme Citation2004). Both taxa have already shown significant impacts on the diversity, structure and dynamics of the native plant and animal species (Palmer, Linde, and Pons Citation2004; Vilà et al. Citation2006; Orgeas et al. Citation2007; Galán Citation2008), and soil characteristics and community (Vilà et al. Citation2006; Conser and Connor Citation2009; de la Peña et al. Citation2010; Zedda et al. Citation2010; Santoro et al. Citation2011; Novoa et al. Citation2013). Among Mediterranean islands where Carpobrotus are invasive (Affre et al. Citation2010), the island of Bagaud (southeastern France) is particularly affected by their invasion (Médail, Affre, and Suehs Citation2004). On this island, Carpobrotus has locally caused (i) a decrease in the species richness and diversity of rare and endemic plants together with the disappearance of some functional groups and biological types; (ii) changes in soil chemistry (pH, C, N and C/N); and (iii) a decrease in pollinator visits to native plants (Suehs Citation2005; Affre Citation2011).
With the aim of protecting island ecosystems, the eradication of invasive species is sometimes the most effective means of mitigating their impact (Pascal and Chapuis Citation2000; Loope et al. Citation2006). Eradication, which is the elimination of all individuals of a species, is a management tool that allows prevention of impacts caused by exotic species (Genovesi Citation2011). A variety of techniques can be used to remove exotic species (hand removal, mechanical removal, fire, etc.), which are chosen based on different factors such as legal constraints, protection status, economic resources or the associated risk to native flora and fauna (D’Antonio and Meyerson Citation2002).
After Bagaud became a strict nature reserve (réserve intégrale) in 2007, an ecological restoration programme was launched by Port-Cros National Park with the aim of eradicating the two Carpobrotus species by hand removal (Médail, Affre, and Suehs Citation2004). However, invasive species can leave a legacy (residual effects) after their removal, which makes long-term restoration difficult; this legacy has to be taken into account when planning restoration protocols and follow-up costs (D’Antonio and Meyerson Citation2002). The legacy may be in the form of a buried seed bank, i.e. viable seeds stored in the soil. Under these conditions, the invasive species may have an increased seed abundance, while native species’ seed richness and abundance may be significantly decreased (Holmes and Cowling Citation1997). The degree to which this occurs depends upon the length of time that the invader has been present (D’Antonio and Meyerson Citation2002). It is therefore important to assess the seed bank, which plays an essential role in the natural regeneration of a native plant community after restoration actions have been implemented (Bakker et al. Citation1996; Dulière, Mahy, and Delescaille Citation2001; Bossuyt and Hermy Citation2003).
In the present study, we examine, on Bagaud island, the plant composition of the vegetation and seed bank in (i) patches of Carpobrotus and (ii) native plant communities. The present analysis is, to our knowledge, the first thorough study of the legacy of Carpobrotus in the form of its seed bank; it was carried out to help identify adequate ecological restoration protocols and to gauge the necessity of planning a post-eradication follow-up intervention against Carpobrotus reinvasion.
Material and methods
Study site
Bagaud island, strict nature reserve since 2007, is part of Port-Cros National Park (southeastern France) in the Mediterranean Sea (43°00’42” N, 6°21’45” E). This 58-ha island is located 7.5 km from the mainland shore, 1.48 km long (north–south), 0.59 km wide (east–west) and exhibits a low relief with a peak elevation of 57 m. Like the island climate typical of the Mediterranean region, Bagaud is characterized by mild winters and a high relative air humidity (81% / year) that persists into the summer.
Carpobrotus invasion
Carpobrotus edulis and C. acinaciformis were introduced to Bagaud island intentionally in the mid-nineteenth century. This was done to stabilize the embankments that were created during military fort construction. These two taxa are now classified as major invasive species in southeastern France (Provence–Alpes–Côte d’Azur; Médail, Affre, and Suehs Citation2004). They are succulent chamaephytes, slightly ligneous at their base. They grow crawling on the ground, rooting at nodes and forming large, dense mats. Their invasiveness is based on various characteristics typical of invasive plants, such as (i) a lack of efficient pathogens in the invaded range (i.e. Enemy Release Hypothesis: Williamson Citation1996; Keane and Crawley Citation2002), (ii) a high genetic and clonal diversity, (iii) fast and vigorous clonal growth, (iv) various sexual reproduction strategies, (v) high and recurrent frequencies of hybridization and introgression, or (vi) fruit and seed dispersion by endozoochory through small mammals, e.g. rabbits and black rats (Suehs, Médail, and Affre Citation2001; Bourgeois et al. Citation2004, Citation2005; Suehs, Affre, and Médail Citation2004a, Citation2004b, Citation2005; Suehs et al. Citation2006). On the island of Bagaud, Carpobrotus acinaciformis is a hybrid of introgressed origin, therefore we refer to it as C. affine acinaciformis instead of C. acinaciformis (see Suehs, Affre, and Médail Citation2004a, Citation2004b). This invasive profile makes it possible for these taxa to have good local spatial dynamics, to colonize various types of coastal habitat, and to have a high evolutionary potential with major impacts on Mediterranean ecosystems (Suehs Citation2005; Affre Citation2011). Carpobrotus invasion is therefore associated with a decrease in native species’ richness and diversity, changes in soil chemistry, and disruption of pollinator networks (Suehs Citation2005; Affre Citation2011).
Experimental design
On the island of Bagaud, C. edulis mainly grows on the coastal rocks and cliffs while C. affine acinaciformis mainly grows inland; these two species are found on an area of about 2 ha (1 ha for each species) where they represent most of the vegetation cover (Passetti et al. Citation2012). Our study of the vegetation and seed bank was carried out on four areas in these two types of environments (coast and inland) each one invaded or non-invaded by Carpobrotus: (i) in C. edulis patches located on the coast, (ii) in C. affine acinaciformis patches located inland, (iii) in the coastal non-invaded native plant community and (iv) in the inland non-invaded native plant community (low mattoral) found closest to inland Carpobrotus patches. We studied these four areas separately rather than pooling the invaded versus the non-invaded areas because the two Carpobrotus species and the inland and coastal vegetation may have different seed banks, and soil depth was different inland and on the coast.
Vegetation sampling
The composition of plant communities was studied in May 2010, when most species were identifiable, in 60 quadrats of 1 m² each, randomly placed in the four areas: (i) 24 quadrats in C. edulis patches, (ii) 24 quadrats in C. affine acinaciformis patches, (iii) six quadrats in the coastal native plant community, and (iv) six quadrats in the inland native plant community. In each quadrat, the percentage cover of each plant species was visually assessed. We opted for an unbalanced sampling designed because the areas invaded by Carpobrotus were larger than those covered by the particular kind of native vegetation which should cover the invaded areas.
Seed bank sampling
The seed bank was sampled in May 2010 in the four areas. Six samples were taken at each depth: (i) in soil 0–5 cm depth in the four areas; (ii) in soil >5 cm depth in the inland area where the soil is deeper, and (iii) in Carpobrotus litter on invaded areas (Table ). Each sample was composed of three sub-samples mixed together to obtain a 1-litre sample. We studied these three depths because they can be considered separately within the framework of eradication: if one or several of these layers contain many Carpobrotus seeds, they may be removed to reduce post-eradication follow-up intervention against Carpobrotus reinvasion.
Table 1. Number of samples taken for the seed bank study for each area and soil depth.
The “seedling emergence method” (Ter Heerdt et al. Citation1996) was chosen to qualify and quantify the seed presence in the seed bank. First, the collected samples were concentrated, rinsing them with water and straining them through 2-mm and 200-μm sieves to reduce bulk and eliminate clay. The concentrated samples were then thinly spread in plastic vermiculite-filled trays topped with medical compresses (mesh size 100 μm) to prevent seed from migrating through the substrate. All trays were watered frequently from below as often as needed to keep the substrate moist. After 3 months, the samples were dried out, micro-ploughed, and watered again for 3 months. Emerged seedlings were identified (Mamarot and Rodrigue Citation2011), counted and removed weekly. Unidentified seedlings were grown so that their identities could be established later.
Statistical analyses
Analyses were performed using the program R (R Development Core Team Citation2008) and made use of the following R packages: “coin” (Hothorn et al. Citation2008), “ade-4” and “vegan”.
Seed bank species richness and density (per litre of soil) were analysed using permutation tests (Hothorn et al. Citation2008). The p-values for the multiple comparison tests were recalculated with the BH adjustment (Benjamini and Hochberg Citation1995). Six tests were run: three on seed bank species richness and three on seed bank density (with and without Carpobrotus). For each of these two variables, tests were run (i) on the seed bank in the litter between the two invaded areas, (ii) on the seed bank in soil 0–5 cm depth between the four areas, and (iii) on the seed bank in soil >5 cm depth between the inland invaded and non-invaded areas.
To study the seed bank and the vegetation composition, two non-metric multidimensional scaling analyses (Borcard, Gillet, and Legendre Citation2011) were run on (i) plant percentage cover (60 quadrats × 40 species) and (ii) seed bank samples whose seed density was determined including Carpobrotus (63 samples × 28 species). These analyses were followed by a multiple response permutation procedure analysis to determine the statistical significance of differences between the four sampled areas and the three soil depths.
To jointly study the composition of the vegetation with that of the seed bank, a co-inertia analysis (Dolédec and Chessel Citation1994) was run on: (i) the seed bank in the litter and surface soil (36 samples × 33 species) and (ii) vegetation in Carpobrotus patches (36 of the 48 samples × 45 species). The analysis was validated by a Monte Carlo permutation test (999 permutations). For the multivariate analyses, the two Carpobrotus taxa were named the same.
Results
Species richness of the seed bank
No significant differences in seed bank species richness were found in the litter between the two invaded areas (permutation test: Z = –1.16, p = 0.325), in soil 0–5 cm depth between the four areas (permutation test: maxT = 1.90, p = 0.228) or in soil >5 cm depth between the inland invaded and non-invaded areas (permutation test: Z = –0.22, p = 0.841). Total species richness consisted of 11 species in coastal C. edulis patches, 19 species in inland C. affine acinaciformis patches, nine species in the coastal native plant community, and 16 species in the inland native plant community.
Seed density of the seed bank
The mean number of seeds in the litter tended to be lower in the inland seed banks of C. affine acinaciformis patches (10.61 seeds/sample) compared with that of coastal seed banks C. edulis patches (44.39 seeds/sample) and tended to be lower when not taking Carpobrotus seeds into account (with Carpobrotus seeds, 42.78 seeds/sample versus without Carpobrotus seeds, 12.22 seeds/sample) (permutation test: maxT = 3.03, p = 0.012; Figure ).
Figure 1. Mean number of seeds in the litter in each 1-litre sample in the two invaded areas taking Carpobrotus seeds into account (white bars) and without including Carpobrotus seeds (grey bars) (permutation test: maxT = 3.03, p = 0.012). The multiple comparison tests calculated with the BH adjustment (Benjamini and Hochberg Citation1995) were not significant.
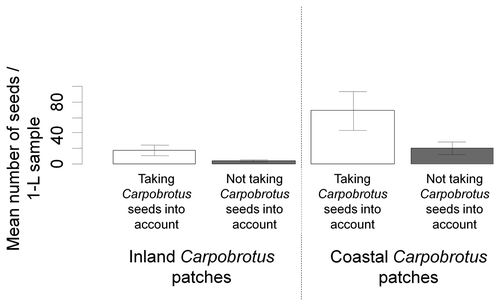
We observed no significant difference in the mean number of seeds in soil 0–5 cm deep between the four areas whether taking Carpobrotus seeds into account or not (permutation test: maxT = 2.73, p = 0.198). The coastal areas (whether invaded, 77.28 seeds/sample or not, 99.17 seeds/sample) tended to have more seeds than inland areas (whether invaded, 19.06 seeds/sample or not, 7.33 seeds/sample; Figure ). On the coast, invaded and non-invaded areas had numerous Carpobrotus seeds: invaded taking Carpobrotus seeds into account: 93.89 seeds/sample versus invaded not taking Carpobrotus seeds into account: 60.67 seeds/sample; non-invaded taking Carpobrotus seeds into account: 170.67 seeds/sample versus non-invaded not taking Carpobrotus seeds into account: 27.67 seeds/sample (Figure ). We observed no significant difference in the mean number of seeds in soil >5 cm depth between the inland invaded and non-invaded areas (permutation test: maxT = 1.08, p = 0.686).
Composition of the vegetation and the seed bank
In total, 56 species were identified in the vegetation and seed bank. Among these, 40 were found among the vegetation (e.g. Arisarum vulgare, Asparagus acutifolius, Avena barbata, Brachypodium distachyon, Brachypodium retusum), 28 species in the seed bank (e.g. Anagallis arvensis, Atriplex prostrata, Crepis foetida, Limonium pseudominutum) and 10 species were found in both vegetation and seed bank (Carpobrotus, Cistus salviifolius, Dorycnium hirsutum, Frankenia sp., Lotus cytisoides, Phillyrea angustifolia, Rosmarinus officinalis, Senecio cineraria, Sonchus asper subsp. asper and Sonchus asper subsp. glaucescens). Patches of Carpobrotus had more than 75% cover of the two invasive taxa (mean: 77 ± 20%); a few native species were occasionally found in these patches, such as Dactylis glomerata, Pistacia lentiscus, Rubia peregrina or Sonchus bulbosus. The co-inertia analysis did not allow us to detect any significant co-structure between the vegetation and the seed bank (RV = 0.23, p = 0.235), indicating that their compositions were different.
The non-metric multidimensional scaling analysis on the vegetation (stress: 0.06) showed a great similarity of composition between invaded patches characterized by Carpobrotus (Figure ). The multiple response permutation procedure analysis showed a significant difference between the four areas (observed Δ: 0.24; expected Δ: 0.56; p < 0.001). As shown in Figure , axis 1 delineates Carpobrotus patches from the quadrats that sampled native plant communities characterized by Bromus madritensis, Carex flacca, Dorycnium hirsutum, Lagurus ovatus, Pinus halepensis, Rosmarinus officinalis or Senecio cineraria; and axis 2 separates the coastal native plant community (Juncus acutus, Pinus halepensis) from the inland native plant community (Bromus madritensis, Cistus salviifolius, Lotus cytisoides, Rubia peregrina).
Figure 3. Non-metric multidimensional scaling analysis of plant species percentage cover (60 quadrats × 40 species). Only the most correlated species are shown. Samples are grouped according to the area.
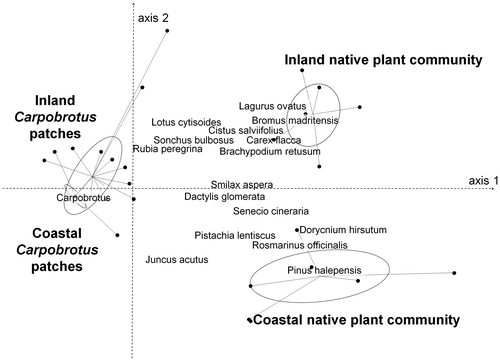
In total, 3264 seeds belonging to 28 species germinated in the seed bank study. Some of these species were annuals (Anagallis arvensis, Solanum nigrum, Atriplex prostata, Sonchus asper, Sonchus oleraceus) and others were perennials (Carpobrotus, Cistus salviifolius, Dorycnium hirsutum, Lotus cytisoides, Senecio cineraria). The non-metric multidimensional scaling analysis on the seed bank (stress: 0.12) did not clearly distinguish the four areas or the three soil depths, despite the significance of the multiple response permutation procedure analysis (observed Δ: 0.74; expected Δ: 0.80; p < 0.001; Figure ). Of the 3264 seeds that germinated, more than 90% belonged to one of the following three taxa: Carpobrotus (63.6%), Frankenia sp. (23.3%), which is almost exclusively found on the coast, and Atriplex prostata (6.6%), which is found inland.
Discussion
On Bagaud island the composition of the vegetation in Carpobrotus patches was homogeneous, and formed nearly monospecific mats of Carpobrotus (Médail, Affre, and Suehs Citation2004; Brunel et al. Citation2010). It is clear that their removal would open up new habitat that could potentially be colonized by native plant species: a few individuals of Dactylis glomerata, Pistacia lentiscus, Rubia peregrina and Sonchus bulbosus were found in Carpobrotus patches, and it is also possible for native vegetation to colonize beginning from Carpobrotus patch edges. On the other hand, native plant communities found around Carpobrotus patches were different between the coastal area, where Juncus acutus and Senecio cineraria were more particularly found, and inland, where Poaceae and Cistus salviifolius were more common. We can therefore expect different plant communities to form after Carpobrotus removal, depending on where on the island the removed Carpobrotus patch had been located. Patches will also be recolonized from the seed bank: at every depth at which we studied the seed bank, we detected a plant composition different from that of the above-ground vegetation, e.g. a large number of Atriplex prostrata and Frankenia sp. seeds were found under Carpobrotus patches); the resulting plant composition will depend on the eradication protocol.
Carpobrotus branches and leaves decompose very slowly, forming a thick litter (Conser and Connor Citation2009) that can be left in place or hauled away during Carpobrotus removal. If the litter is left in place, colonization from the seed bank will occur from the litter. Even when Carpobrotus litter was found to contain some native species seeds, such as Atriplex prostrata, Frankenia sp. and Sonchus asper, it also contained many Carpobrotus seeds (77.6%), particularly on the coast. However, Novoa et al. (Citation2012, Citation2013) also showed that C. edulis creates a chemical barrier for seed germination because it causes allelopathic substances to accumulate in the litter. Leaving Carpobrotus litter on areas from where Carpobrotus live plants have been removed would therefore be detrimental to the germination of all plant species.
If litter removal is combined with live Carpobrotus removal, the seeds available for recolonization will be those contained in the surface soil, here mainly composed of Atriplex prostrata, Frankenia sp., Juncus acutus and Lotus cytisoides in addition to Carpobrotus. Removing the litter would allow these seeds to germinate because the thick Carpobrotus mats (live branches + litter) form an obstacle to the germination of native plant species, particularly annuals (Vilà et al. Citation2006).
This restoration protocol would therefore allow more native species seeds to germinate but would require post-eradication follow up of Carpobrotus reinvasion in the short-term and mid-term. This is also true for coastal zones where C. edulis is mainly found, because its production of seeds and fruit is twice as high as that of C. affine acinaciformis (Suehs Citation2005).
Although deeper soil does contain non-trivial quantities of Carpobrotus seeds, these seeds are usually found in smaller numbers than in surface soil or litter. Deeper soil also contains fewer native seeds than surface soil. However, because of the intense level of effort that it would entail, it would be hardly worthwhile to remove both the litter and the upper soil layer, even if it would mean not having to carry out tedious post-eradication follow-up efforts. Moreover, this would remove all soil layers on the coastal areas, leading to primary succession.
This study has also shown that a large number of Carpobrotus seeds can be found in the native plant community seed banks, but they do not germinate within that context, and are probably dispersed by Rattus rattus on Bagaud island (Bourgeois et al. Citation2004, Citation2005). It is therefore very important during restoration interventions to limit the types of disturbances to native vegetation, which could lead to Carpobrotus germination in unaffected areas. It has been shown that C. edulis can colonize new habitats after a disturbance (e.g. fire as reported in D’Antonio and Meyerson Citation2002).
Conclusion
The present study allowed us to identify the most adequate ecological restoration protocol for local removal of Carpobrotus. To summarize, the protocol consists of (i) removing parts of Carpobrotus live plants and (ii) removing Carpobrotus litter, which contains many Carpobrotus seeds and which prevents the germination of all plant species. This eradication will have to be completed by short-term and mid-term post-eradication follow-up interventions against Carpobrotus reinvasion as the soil surface contains many Carpobrotus seeds. Surveillance of disturbance to native vegetation is also desirable.
Notes on contributors
Julie Chenot is a qualified technician in plant ecology (plant community, seed rain and seed banks) and on invasive species (plants and animals).
Elise Buisson is an associate professor at Université d’Avignon et des Pays de Vaucluse and at the Mediterranean Institute of Biodiversity and Ecology. She conducts research on plant communities, their functioning and vulnerability, and is interested in their organization, their resilience and their restoration. Most of her research concerns herbaceous plant communities.
Aurélie Passetti is an ecologist working for an environmental consulting firm; her areas of study include botany, scientific monitoring and invasive species and natural area management.
Laurence Affre is an associate professor at Aix-Marseille Université and at the Mediterranean Institute of Biodiversity and Ecology. She carries out research in plant population biology and experimental ecology in controlled conditions and in nature, particularly on rare and invasive plants.
Acknowledgements
The implementation of this study has been made possible thanks to the major support of Port-Cros National Park, the Institut Méditerranéen de Biodiversité et d’Ecologie (IMBE) and numerous funding partners (the European Union, Conservatoire du littoral, Fondation Total). We thank the staff at Port-Cros National Park, and Eric Vidal and Frédéric Médail (IMBE) for their commitment and logistical support. We also thank all the people who helped with our field work, in particular Daniel Pavon (IMBE botanist) who provided invaluable assistance in identifying plants and seedlings.
References
- Affre, L. 2011. “Ecological and Evolutionary Insights from Plant Invasions: The Case of Carpobrotus spp. (Aizoaceae) in the Mediterranean Basin.” In Advances in Medicine and Biology, edited by L.V. Berhardt, 1–40. New York, NY: Nova Science Publishers, Hauppauge.
- Affre, L., C. M. Suehs, S. Charpentier, M. Vilà, G. Brundu, P. Lambdon, A. Traveset, and P. E. Hulme. 2010. “Consistency on the habitat degree of invasion for three invasive plant species across Mediterranean islands.” Biological Invasions 12: 2537–2548.
- Bakker, J. P., P. Poschlod, R. J. Strykstra, R. M. Bekker, and K. Thompson. 1996. “Seed banks and seed dispersal: important topics in restoration ecology. Review.” Acta Botanica Neerlandica 45(4): 461–490.
- Benjamini, Y., and Y. Hochberg. 1995. “Controlling the False Discovery Rate: a practical and powerful approach to multiple testing.” Journal of the Royal Statistical Society Series B57: 289–300.
- Borcard, D., F. Gillet, and P. Legendre. 2011. Numerical Ecology with R. New York: Springer.
- Born, W., F. Rauschmayer, and I. Bräuer. 2004. “Economic evaluation of biological invasions—a survey.” Ecological Economics 55: 321–336.
- Bossuyt, B., and M. Hermy. 2003. “The potential of soil seedbanks in the ecological restoration of grassland and heathland communities.” Belgian Journal of Botany 136: 23–34.
- Bourgeois, K., E. Vidal, C. Suehs, and F. Médail. 2004. “Extreme invasional meltdown: multitrophic interactions catalyse Mediterranean island invasions.” In Ecology, conservation and management of Mediterranean climate ecosystems, edited by M. Arianoutsou and V. P. Papanastasis, 1–5. Rotterdam: Millpress Science Publishers.
- Bourgeois, K., C. M. Suehs, E. Vidal, and F. Médail. 2005. “Invasional meltdown potential: facilitation between introduced plants and mammals on French Mediterranean islands.” Écoscience 12(2): 248–256.
- Brunel, S., G. Schrader, G. Brundu, and G. Fried. 2010. “Emerging invasive alien plants for the Mediterranean Basin.” OEPP/EPPO Bulletin 40: 407–422.
- Cassey, P. 2003. “A comparative analysis of the relative success of introduced landbirds on islands.” Evolutionary Ecology Research 5: 1011–1021.
- Conser, C., and E. F. Connor. 2009. “Assessing the residual effects of Carpobrotus edulis invasion, implications for restoration.” Biological Invasions 11: 349–358.
- Cronk, Q. 1997. “Islands: stability, diversity, conservation.” Biodiversity and Conservation 6(3): 477–493.
- D’Antonio, C. M., and K. Haubensak. 1998. “Community and ecosystem impacts of introduced species in California.” Fremontia 26: 16–18.
- D’Antonio, C. M., and L. A. Meyerson. 2002. “Exotic plant species as problems and solutions in ecological restoration: a synthesis.” Restoration Ecology 10: 703–713.
- Denslow, J. 2001. “The ecology of insular biotas.” Trends in Ecology and Evolution 16: 423–424.
- de la Peña, E., N. de Clercq, D. Bonte, S. Roiloa, S. Susana Rodríguez-Echeverría, and H. Freitas. 2010. “Plant-soil feedbacks as a mechanism of invasion by Carpobrotus edulis.” Biological Invasions 12(10): 3637–3648.
- Dolédec, S., and D. Chessel. 1994. “Co-inertia analysis: an alternative method for studying species–environment relationships.” Freshwater Biology 31: 277–294.
- Drake, D., C. Mulder, D. Towns, and C. Daugherty. 2002. “The biology of insularity: an introduction.” Journal of Biogeography 29: 563–569.
- Dulière, J. F., G. Mahy, and L.-M. Delescaille. 2001. “L’étude de la banque de graines du sol, un outil efficace pour orienter la restauration des milieux semi-naturels.” Parcs et Réserves 56(3): 16–26.
- Elton, C. 1958. The ecology of invasions by animals and plants. London: Methuen.
- Galán, P. 2008. “Efecto de la planta invasora Carpobrotus edulis sobre la densidad del eslizón tridáctilo (Chalcides striatus) en una localidad costera de Galicia.” Boletín de la Asociación Herpetológica Española 19: 117–121.
- Genovesi, P. 2011. “Eradication.” In Encyclopedia of Biological Invasions, edited by D. Simberloff and M. Rejmanek, 198–203. Berkeley and Los Angeles: University of California Press.
- Holmes, P. M., and R. M. Cowling. 1997. “Diversity, composition and guild structure relationships between soil-stored seed banks and mature vegetation in alien plant-invaded South African fynbos shrubland.” Plant Ecology 133: 107–122.
- Hothorn, T., K. Hornik, M. A. Van de Wiel, and A. Zeileis. 2008. “Implementing a Class of Permutation Tests: The coin Package.” Journal of Statistical Software 28: 1–23.
- Hulme, P. E. 2004. “Islands, invasions and impacts: a Mediterranean perspective.” In Ecologia insular / Island Ecology, edited by J. M. Fernández-Palacios and C. Morici, 359–383. Cabildo Insular de la Palma: Asociaciòn Española de Ecologia Terrestre (AEET).
- Keane, R. M., and M. J. Crawley. 2002. “Exotic plant invasions and the enemy release hypothesis.” Trends in Ecology and Evolution 17: 164–170.
- Loope, L., A. Sheppard, M. Pascal, and H. Jourdan. 2006. “L’éradication : une mesure de gestion des populations allochtones.” In Les espèces envahissantes dans l’archipel néo-calédonien, edited by M.-L. Beauvais, A. Coléno, H. Jourdan and D. Chouchan, 401–417. Paris: IRD.
- Mack, R. N., D. Simberloff, M. Lonsdale, H. Evans, M. Clout, and F. A. Bazzaz. 2000. “Biotic invasions: causes, epidemiology, global consequences and control.” Ecological Applications 10: 689–710.
- Mamarot, J., and A. Rodrigue. 2011. Mauvaises herbes des cultures. Paris: Acta.
- McKinney, M. L., and J. L. Lockwood. 1999. “Biotic homogenization: a few winners replacing many losers in the next mass extinction.” Trends in Ecology and Evolution 14(11): 450–453.
- Médail, F. and N. Myers. 2004. “Mediterranean Basin.” In Hotspots revisited: Earth’s biologically richest and most endangered terrestrial ecoregions edited by R.A. Mittermeier, P. Robles Gil, M. Hoffmann, J. Pilgrim, T. Brooks, C.G. Mittermeier, J. Lamoreaux, and G.A.B. da Fonseca, 144–147. Monterrey: CEMEX, Washington: Conservation International, and Mexico: Agrupación Sierra Madre.
- Médail, F., L. Affre, and C. Suehs. 2004. “Carpobrotus sp. C. edulis (L.) N.E. Br. & C. aff. acinaciformis (L.) L. Bolus. Les griffes de sorcières.” In Plantes invasives en France : état des connaissance et propositions d’actions edited by S. Muller, 52–55. Paris: Collections Patrimoines Naturels, Publications Scientifiques du Muséum National d’Histoire Naturelle.
- Milbau, A., and J. C. Stout. 2008. “Factors associated with alien plants transitioning from casual, to naturalized, to invasive.” Conservation Biology 22(2): 308–317.
- Mooney, H. A., and E. E. Cleland. 2001. “The evolutionary impact of invasive species.” Proceedings of the National Academy Society London 98: 5446–5451.
- Myers, N., R. A. Mittermeier, C. G. Mittermeier, G. A. B. da Fonseca, and J. Kent. 2000. “Biodiversity hotspots for conservation.” Nature 403: 853–858.
- Novoa, A., L. González, L. Moravcová, and P. Pyšek. 2012. “Effects of Soil Characteristics, Allelopathy and Frugivory on Establishment of the Invasive Plant Carpobrotus edulis and a Co-Occuring Native, Malcolmia littorea.” PLoS ONE 7(12): 1–11.
- Novoa, A., L. González, L. Moravcová, and P. Pyšek. 2013. “Constraints to native plant species establishment in coastal dune communities invaded by Carpobrotus edulis: implications for restoration.” Biological Conservation 164: 1–9.
- Nunn, P. 1994. Oceanic islands. Oxford: Blackwell.
- Orgeas, J., P. Ponel, S. Fadda, A. Matocq, and A. Turpaud. 2007. “Conséquences écologiques de l’envahissement des griffes de sorcière (Carpobrotus ssp.) sur les communautés d’insectes d’un îlot du Parc national de Port Cros (Var).” Scientific Reports of Port-Cros National Park 22: 233–257.
- Palmer, M., M. Linde, and G. X. Pons. 2004. “Correlational patterns between invertebrate species composition and the presence of an invasive plant.” Acta Oecologia 26: 219–226.
- Pascal, M. 2009. “Les invasions biologiques : comprendre pour maitriser. La conquête des espèces.” Pour la science 65: 4–6.
- Pascal, M., and J. Chapuis. 2000. “Éradication de mammifères introduits en milieux insulaires: questions préalables et mise en application.” Revue d’écologie 7: 85–104.
- Passetti, A., A. Aboucaya, E. Buisson, J. Gauthier, F. Médail, M. Pascal, P. Ponel, E. Vidal 2012. “Restauration écologique de la Réserve Intégrale de l’île de Bagaud et “état zéro” des suivis scientifiques : synthèse méthodologique.” Journal du Parc National de Port Cros 26:149–171.
- R Development Core Team. 2008. R: A language and environment for statistical computing. Vienna, Austria: R Foundation for Statistical Computing. http://www.R-project.org
- Richardson, D., P. Pyšek, M. Rejmánek, M. Barbour, F. Panetta, and C. West. 2000. “Naturalization and invasion of alien plants: concepts and definitions.” Diversity and Distributions 6: 93–107.
- Ricketts, T. H., E. Dinerstein, T. Boucher, T. Brooks, S. Butchart, M. Hoffmann, J. F. Lamoreux, et al. 2005. “Pinpointing and preventing imminent extinction.” Proceedings of the National Academy of Sciences of the USA 51: 18497–18501.
- Santoro, R., T. Jucker, M. L. Carranza, and A. T. R. Acosta. 2011. “Assessing the effects of Carpobrotus invasion on coastal dune soils. Does the nature of the invaded habitat matter?” Community Ecology 12: 234–240.
- Sax, D. F., and S. D. Gaines. 2008. “Species invasions and extinction: The future of native biodiversity on islands.” Proceedings of the National Academy of Sciences of the USA 105: 11490–11497.
- Sax, D. F., S. D. Gaines, and J. H. Brown. 2002. “Species invasions exceed extinctions on islands worldwide: a comparative study of plants and birds.” American Naturalist 160: 766–783.
- Sol, D. 2000. “Are islands more susceptible to be invaded than continents? Birds say no.” Ecography 23(6): 687–692.
- Suehs, C.M. 2005. “Ecological and evolutionary factors influencing the invasion of Carpobrotus spp (Aizoaceae) in the Mediterranean region.” PhD thesis, Université Aix Marseille III, France.
- Suehs, C. M., F. Médail, and L. Affre. 2001. “Ecological and genetic features of the invasion by the alien Carpobrotus Plants in Mediterranean island habitats.” In Plant Invasions : species and ecosystem management, edited by G. Brundu, J. H. Brock, I. Camarga, L. Child and M. Wade, 145–158. Leiden: Blackhuys Publishers.
- Suehs, C. M., L. Affre, and F. Médail. 2004a. “Invasion dynamics of two alien Carpobrotus (Aizoaceae) taxa on a Mediterranean Island: I. Genetic diversity and introgression.” Heredity 92: 31–40.
- Suehs, C. M., L. Affre, and F. Médail. 2004b. “Invasion dynamics of two alien Carpobrotus (Aizoaceae) taxa on a Mediterranean Island: II. Reproductive strategies.” Heredity 93: 1–7.
- Suehs, C. M., L. Affre, and F. Médail. 2005. “Unexpected insularity effects in invasive plant mating systems: the case of Carpobrotus (Aizoaceae) taxa in the Mediterranean Basin.” Biological Journal of the Linnean Society 85: 65–79.
- Suehs, C. M., S. Charpentier, L. Affre, and F. Médail. 2006. “The evolutionary potential of invasive Carpobrotus (Aizoaceae) taxa: are pollen-mediated gene flow potential and hybrid vigor levels connected?” Evolutionary Ecology 20: 447–463.
- Ter Heerdt, G. N. J., G. L. Verweij, R. M. Bekker, and J. P. Bakker. 1996. “An improved method for seed-bank analysis: Seedling emergence after removing the soil by sieving.” Functional Ecology 10: 144–151.
- Vilà, M., M. Tessier, C. M. Suehs, G. Brundu, L. Carta, A. Galanidis, P. Lambdon, et al. 2006. “Local and regional assessments of the impacts of plant invaders on vegetation structure and soil properties of Mediterranean islands.” Journal of Biogeography 33: 853–861.
- Vilà, M., J. L. Espinar, M. Hedja, P. E. Hulme, V. Jarošík, J. L. Maron, J. Pergl, U. Schaffner, Y. Sun, and P. Pyšek. 2011. “Ecological impacts of invasive alien plants: a meta-analysis of their effects on species, communities and ecosystems.” Ecology Letters 14(7): 702–708.
- Vitousek, P. M., C. M. D’Antonio, L. L. Loope, and R. Westbrooks. 1996. “Biological Invasions as Global Environmental Change.” American Scientist 84: 468–478.
- Whittaker, R. 1998. Island biogeography. Ecology, Evolution, and Conservation. Oxford: Oxford University Press.
- Williamson, M. H. 1996. Biological Invasions. London: Chapman & Hall.
- Zedda, L., A. Cogoni, F. Flore, and G. Brundu. 2010. “Impacts of alien plants and man-made disturbance on soil-growing bryophyte and lichen diversity in coastal areas of Sardinia (Italy).” Plant Biosystems 144(3): 547–562.