Abstract
The present study revealed that adaxial and abaxial petal epidermises of the blue-flowered Lysimachia arvensis consist of elongated, multi-micro-papillate cells, which may aid the rapid petal expansion. The epidermal cells are covered by a wrinkled relief, which is further ornamented by submicron features that increase in size the surface area of lobes; this may be a well-adapted mechanism of the small-sized flowers of L. arvensis with the short life span. The sculpturally increased surface area of adaxial epidermal cells of petals is expected to contribute to optical and adhesive properties, and wettability of the floral tissues. The adaxial and the abaxial petal surfaces of L. arvensis possess submicron cuticular folds, smaller than the sub-wavelength visible spectrum, which reflect radiation of shorter rather than longer wavelengths, whereas intense absorption was detected in the red spectral region. Also, three-celled capitate trichomes with a pigmented spherical head, which are densely distributed at the corolla margins of L. arvensis, may be involved in adhesive, defensive and functional properties of the floral tissues.
Introduction
Flowers are among the most spectacular products of nature. Blossoming of plants is regulated by mechanisms that act to ensure that flower emergence occurs in suitable environmental conditions (Elzinga et al. Citation2007; Tooke and Battey Citation2010). Major importance has been given to floral advertisement, which is related to multifunctional traits; for example, a three-dimensional cuticular relief, observed on petal surfaces, protects the floral tissues against physical, chemical and biological attack, it influences optical properties, it reduces the absorbance of UV radiation that reaches the cells and forms microsculptures (Kevan and Lane Citation1985; Jacobs, Koper, and Ursem Citation2007; Whitney and Glover Citation2007; Domínguez, Heredia-Guerrero, and Heredia Citation2011; Javelle et al. Citation2011; Glover et al. Citation2013). It is worth mentioning that multifunctional, submicron features of petal epidermises, which serve as interfaces between the tissues and their environment, provide a potential natural template for man-made applications, which have been transferred to biomimetic materials (Ma and Sun Citation2009; Bhushan and Her Citation2010; Shimomura Citation2010; Uchida et al. Citation2010; Qian et al. Citation2011; Telford et al. Citation2013).
Lysimachia arvensis (L.) U. Manns & Anderb. (Primulaceae), formerly Anagallis arvensis L. (Manns and Anderberg Citation2009), is a slender little plant, creeping on the ground with spreading stems in wild and cultivated fields, on road sides and in gardens, and considerable research has focused on it (Marsden-Jones and Weiss Citation1960; Verma Citation1972; Simpson and Neff Citation1983; Green, Havelange, and Bernier Citation1991; Hernández et al. Citation1991; Hao et al. Citation2004; Anderberg, Manns, and Källersjö Citation2007; Manns and Anderberg Citation2007; Jiménez-López et al. Citation2015). Lysimachia arvensis is probably native to the Mediterranean region that has been dispersed world-wide (Chrtek and Osbornová-Kosinová Citation1986; Brockett and Cooperrider Citation1983; Manns and Anderberg Citation2011; de Foucault, El Beyrouthy, and Arnold-Apostolides Citation2013). However, over the last few decades, the number and size of populations of this species declined due to modern land-use techniques, application of fertilizers, herbicide use and cleaning procedures (Brütting et al. Citation2012; Bergmeier and Strid Citation2014; Šilc, Lososová, and Vrbničanin Citation2014).
The objective of this study was to identify morphological features of petals of L. arvensis, to understand adaptations of floral tissues – expanded under field conditions – to the abiotic environment. Lysimachia arvensis blossoms by exhibiting bluish flowers under adequate water availability in the field, during spring, i.e. the main flowering period in the Mediterranean region (Harris Citation2007; Rhizopoulou et al. Citation2012; Farré-Armengol et al. Citation2014; Rhizopoulou and Pantazi Citation2015). Stereoscopy, scanning electron microscopy and atomic force microscopy were used to study the micromorphology of petals of L. arvensis. To the best of our knowledge, the topography of adaxial and abaxial petal surfaces of L. arvensis using high-resolution imaging, which enables detailed study of plant surfaces and understanding of relationships between floral tissues and environmental stimuli, has not hitherto been investigated.
Material and methods
Plant material and study area
Plants of the annual plant Lysimachia arvensis (L.) U. Manns & Anderb. (Primulaceae) grow under ambient conditions, creeping close to the ground, in the campus of the University of Athens (38°14.3′N, 23°47.8′E, altitude 250 m, above sea level). Flowering of plants of L. arvensis was observed in fifty individuals, on a regular basis, i.e. every day, between April and May. The mean monthly temperature ranged from 17 ± 2°C (April) to 22 ± 3°C (May), and the monthly precipitation varied from 26 ± 4 mm (April) to 15 ± 2 mm (May). Induction of flowers before the dry summer, in the Mediterranean region, may be interpreted as a predictably evolved response of plants to unfavourable water deficit conditions. Floral longevity was defined as the time from opening to the wilting of the whorls. Fifty expanded, turgid flowers were randomly harvested from L. arvensis plants growing in the field. The corolla diameter was measured on intact flowers of L. arvensis growing in open habitats using a ruler.
Microscopy and stereoscopy
The study was carried out on fully mature petal regions of L. arvensis (Figure ). Petal lobes were viewed using a Zeiss Stemi 2000 C stereoscope equipped with a ProgRes C3 digital camera, and the pictures were recorded using a personal computer. Samples from the middle part of the petal blade were carefully cut into square pieces (4 mm2) and fixed in 3% glutaraldehyde in sodium phosphate buffer at pH 7, at room temperature, for 2 h. The samples were then washed three times by immersion in buffer for 30 min each time, and post-fixed in 1% OsO4 in the same buffer at 4°C. Dehydration of the material was accomplished with a gradient of increasing concentrations of acetone solutions. Dehydrated tissues were embedded in SPURR (Serva) resin. Semi-thin sections of resin-embedded tissue (LKB Ultratome III microtome) were stained in Toluidine Blue ‘0’, in 1% borax solution, photographed and digitally recorded using a Zeiss Axioplan light microscope (Carl Zeiss Inc., Thornwood, NY, USA) equipped with a digital camera (Zeiss AxioCam MRc5). Also, dehydrated samples were dried at the critical point in a Bal-Tec CPD-030 dryer, mounted with double adhesive tape on stubs, and sputter coated with 20-nm gold in a Bal-Tec SCP-050. The adaxial and abaxial epidermises of petals were examined using a JEOL JSM 840 scanning electron microscope (JEOL Ltd, Tokyo, Japan); software associated with this microscope was used for the production of the digital images. Adaxial and abaxial petal areas (5 × 5 μm) were imaged by using a tap mapping atomic force microscope (Multimode SPM, Veeco, Santa Barbara, CA, USA). Several parameters were analysed and processed, using the software package Nanoscope III (Veeco, USA), to detect detailed information about the petal surfaces. The quantitative measurements include horizontal and vertical distances that represented the height of a step between nanofolds, surface roughness, surface distance, i.e. the length between the markers, and the surface area ratio that represents the density of nanofolds. Topological, quantitative components obtained from 40 different samples are presented in representative micrographs and the mean values given in Table are followed by the standard deviation (± SD); height, amplitude modes and distances between the cursors of the presented lines in Figure (B, Figure E) correspond to the average profile of three lines crossing each one of the 40 samples drawn by the investigator, according to McNally and Borgens (Citation2004).
Figure 1. Flowers of Lysimachia arvensis grown in the field (left, scale bar: 1 cm), the sampling region of fully expanded petals is indicated by an arrow and a transverse section through the petal expanded region is presented (right, scale bar: 10 μm).
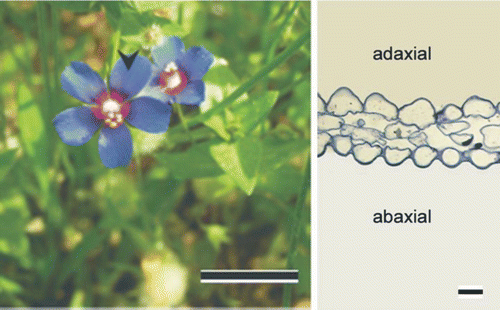
Table 1. Nanometric estimates of trais of the adaxial and the abaxial petal surfaces of Lysimachia arvensis using atomic force microscopy.
In situ measurements of optical properties of fresh petal tissues
Reflectance, transmittance and absorbance were measured in situ on fresh, expanded flowers of L. arvensis adjacent to those sampled for morphological measurements, using a UV/VIS spectrophotometer (Perkin Elmer Lambda-950) equipped with an integrating sphere and glass-fibre tubes (Stratakis et al. Citation2009; Gkikas, Argiropoulos and Rhizopoulou Citation2015).
Spectrophotometric measurements of absorption of petal extracts
Fresh petal-tissues were collected from the same corollas sampled for the in situ measurements of reflectance of L. arvensis and were frozen in liquid nitrogen; then, they were crushed into fine powder and the pigments were extracted with 1% (volume/volume) HCl in methanol and 80% ethanol solutions. Spectrophotometric measurements of absorption bands of solutions of filtered and purified petal extracts were estimated using a Novaspec II spectrophotometer (Pharmacia Biotech, Cambridge, UK).
Flower photography
Black and white photographs of L. arvensis flowers were taken through monochromatic filters (Omega Optical, Brattleboro, VT, USA) in selected spectra, i.e. at 550 nm, 450 nm and UV, under ambient conditions and natural light, using a portable camera-system based on an 8-bit charge-coupled device sensor, which was developed at the Institute of Electronic Structure and Laser (Foundation for Research & Technology Hellas, Heraklion, Greece).
Statistical analysis
Statistically significant differences between measurements in the two petal surfaces were estimated using one-way analysis of variance and Welch & Brown-Forsythe tests. Statistical analysis was performed with the SPSS statistical program. The reported values are means ± SD.
Results
The corollas of L. arvensis are small (5 ± 1 mm long, Figure : left) and exhibit a 2-day lifespan. Lysimachia arvensis takes approximately 1 month to flower and the duration of blossoming in the field averaged a 2-week period extending from April until May.
Transverse sections of petals show papillate epidermal cells at the adaxial and abaxial sides (Figure ; right) and a narrow mesophyll (Figure A). Adaxial and abaxial surfaces of petals of the small, short-lived flowers of L. arvensis possess multipapillate, elongated, epidermal cells (Figure B, Figure C, respectively), which exhibit approximately five (Figure C) to ten (Figure B) micro-papillae surfaces, arranged in a single row per cell. On the outer margin of petals, numerous (Figure A) capitate-stalked trichomes (approximately 15 hairs mm−1) consist of a nearly spherical head cell, a stalk cell and a basal cell, which emerges from the epidermis (Figure B).
Figure 2. Scanning electron micrographs of petal of Lysimachia arvensis. (A) Transverse section (scale bar: 5 μm), (B) adaxial epidermis and (C) abaxial epidermis (scale bar: 10 μm) consisting of multi-micro-papillae arranged in elongated cells, as indicated by arrows at the edges of cells.
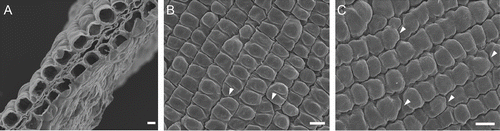
Figure 3. (A) Stereoscopic (scale bar: 0.5 mm) micrograph and (B) scanning electron micrograph (scale bar: 20 μm) showing the outer margin of petals of Lysimachia arvensis with the predominant three-celled capitate trichomes consisting of a nearly spherical head, a stalk cell and a basal cell.
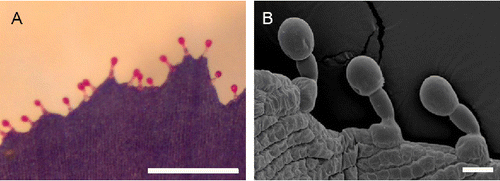
The epidermal cells of petals are covered by a ridged, cuticular relief (Figure ). High-resolution imaging of adaxial (Figure A) and abaxial (Figure D) petal epidermises of L. arvensis revealed cuticular folds at the nanometer scale (Table ). The adaxial and abaxial epidermises possess cuticular folds with a vertical distance of 150 nm and 106 nm, respectively, and a horizontal distance of 1450 nm and 450 nm, respectively. Therefore, adaxial epidermal cells of petals of the small flowers of L. arvensis possess a horizontal distance that is 3.5-fold higher than that of the abaxial epidermal cells (Table ); in other words, spacing between folds of the adaxial and abaxial epidermises is approximately 1.5 μm and 0.5 μm, respectively. The adaxial horizontal distance is approximately 9.5-fold higher than the adaxial vertical distance, and the abaxial horizontal distance is four-fold higher than the abaxial vertical distance (Table ).
Figure 4. Atomic force micrographs of (A–C) adaxial and (D–F) abaxial petal surface of Lysimachia arvensis. (A, D) Three-dimensional profiles; (B, E) integrated line of measured points on plane profiles; and (C, F) profile views of the line section.
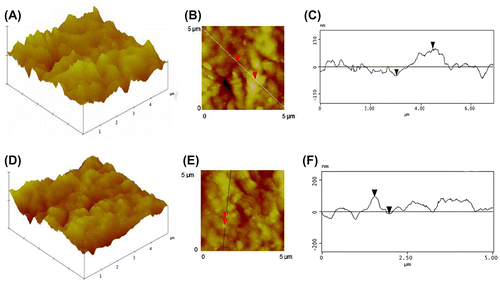
Absorption, reflectance and transmission measured on intact, expanded fresh petals of L. arvensis were found to be approximately 30% between 360 and 440 nm (Figure A). The absorption increased from 32% at 400 nm to 82% at 630 nm (Figure B), whereas reflectance and transmission fell to 10% between 580 and 630 nm (Figure A). In particular, reflectance was about 15% at 320 nm, 32% in the blue spectral range, 8% at 610 nm and 35% at 680 nm (Figure ). Also, the absorption of petal extracts of L. arvensis increased from 500 to 540 nm (Figure A), but the absorption of fresh tissues was higher than that of petal extracts (Figures , Figure A). A remarkable difference in absorption spectra between intact petals and solutions of petal extracts occurred at 600 nm, being approximately 80% and 10%, respectively (Figures B, Figure A). The presence of UV-absorbing compounds (Figures , Figure ) is also indicated and this may protect the short-lived tissues from harmful radiation. It appears that petals of L. arvensis are still absorptive at 550 nm (Figure A), whereas they are mostly reflective at 450 nm (Figure B). The central area of the corolla absorbs UV, contrasting against the UV-reflecting mid-part and periphery of the petal lobes (Figure C).
Figure 5. In situ spectral reflectance (Α), transmission (Α) and absorption (Α, Β) of petals of Lysimachia arvensis.
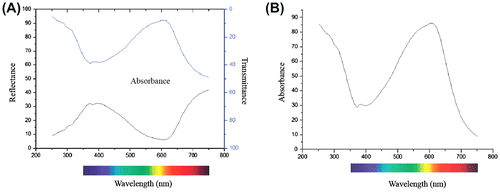
Figure 6. Absorption spectra of fresh petal extracts of Lysimachia arvensis in 1% HCl in (A) methanol and (B) 80% ethanol solutions, over the 250- to 700-nm wavebands.
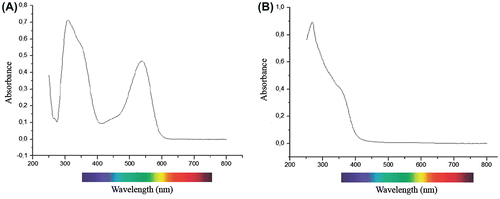
Figure 7. Representative images of intact, small-sized flowers (5 ± 1 mm, long) of Lysimachia arvensis photographed under ambient conditions. (A) Absorptive fully expanded petals and reflective axile anthers at 550 nm. (B) Reflective petal areas contrasting against the absorptive central part of the corolla (as indicated by the arrow) and axile anthers at 450 nm. (C) A defined UV absorptive central area (i.e. the dark part of the corolla) contrasting against the UV-reflecting mid-part of the lobe and the periphery.
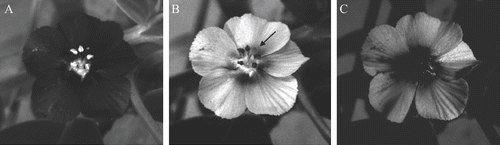
Discussion
The longitudinally elongated, multipapillate epidermal cells, found on both the adaxial and the abaxial surfaces of petals may aid the rapid petal expansion of the flowers of L. arvensis with the short lifespan (Barthlott Citation1981; Kay, Daoud, and Stirton Citation1981; Argiropoulos and Rhizopoulou Citation2012a).
The three-celled capitate trichomes, densely arranged at the corolla lobe margins (approximately 15 hairs mm−1), consist of terminal spherical heads that appear purplish (Figure A), being filled with pigments (e.g. anthocyanins) that have most probably been transferred from epidermal cells through the basal and stalk cells (Fahn Citation1979; Manns and Anderberg Citation2007). Hence, capitate pigmented trichomes positioned on the periphery of petals form boundaries that provide contrast to the background and may play a role in visible distinction (Baird et al. Citation2013). It has been argued that if colour is to be used as a signal to be spotted by other organisms, then it must stand out from a green-grey-yellowish background (Lehrer, Srinivasan, and Zhang Citation1990; Miller, Owens, and Rørslett Citation2011). Trichomes found on petals and reproductive organs of plant species from other families are involved in defence, attractiveness, feeding deterrence and UV protection of the tissues (Vrachnakis Citation2002; Clegg and Durbin Citation2003; Martin and Glover Citation2007; Glas et al. Citation2012; Vitarelli et al. Citation2015). Also, glandular trichomes observed on floral tissues of other Lysimachia species secrete fluids, which are collected by pollinators (Simpson and Neff Citation1983; Schäffler, Balao, and Dötterl Citation2012). The brightly coloured, spherical heads of the capitate trichomes, probably mimicking shining nectar droplets, may facilitate pollen dispersal of the nectarless flowers of L. arvensis (Aldasoro, Aedo, and Navarro Citation2000; Possobom, Guimarães, and Machado Citation2015). In considering that pollen is the only reward for pollinators (Gibbs and Talavera Citation2001; Raine and Chittka Citation2007), the pigmented capitate trichomes on the margin of petals may attract insects by deceit. Also, the marginal, stalked, purplish capitate trichomes of the small flowers of L. arvensis, possess an amplified peripheral surface volume, which may play a role of floral ‘façade’, which may result, to some extent, in the protection of drifted pollen grains. It is worth mentioning that the size of the isopolar, tricolporate pollen grain of L. arvensis is the same order of magnitude as that of the head cells of the capitate trichomes prevailing at the corolla apex (Fernandez and Garcia-Martin Citation1985; Moneim, Atta, and Shehata Citation2003). The average pollen grain diameter is approximately 18 μm, the average polar and equatorial axis lengths are 18.57 μm and 18.25 μm, respectively (Moneim, Atta, and Shehata Citation2003), and the diameter of head cells of capitate trichomes is approximately 18 μm. It is probable that the pollen grains of L. arvensis adhered to the volume of capitate trichomes are retrieved and redistributed at the corolla lobes, facilitating self-fertility of the species during the closure of (autogamous) flowers at the end of the day (Imhoff et al. Citation1979; Simpson and Neff Citation1983; Gibbs Citation2014). Actually, flowers of L. arvensis open in the morning and close in the evening (Imhoff et al. Citation1979; Ortiz et al. Citation2015; personal observation). Interestingly, closed flowers of L. arvensis disappear within the greenish background, because the abaxial bluish petal surfaces are mostly covered by the abaxial, green sepal surfaces.
In addition, flowers of L. arvensis close in cloudy, misty and humid weather (Gibbs and Talavera Citation2001; personal observation); this closure may be coordinated to submicron traits of petals, because petal surface wettability has been found to be affected by cuticular, corrugated folds of the tissues (Wagner et al. Citation2003; Argiropoulos and Rhizopoulou Citation2012b; Taneda et al. Citation2015). High-resolution imaging revealed nanosculptures that may influence the adhesive properties of petals and affect the tissue water status (Barthlott Citation1981; Gale and Owens Citation1983; Miller, Owens, and Rørslett Citation2011; Whitney et al. Citation2011; Chimona et al. Citation2012; Koch et al. Citation2013; van Doorn and Kamdee Citation2014; Buschhaus, Hager, and Jetter Citation2015). It is expected that the vertical-to-horizontal distance ratio (0.10) of the adaxial (inner) petal surfaces, presumably leading to an elevated contact area between the folds of the petal surface and water, will affect both the firmness of the contact with water and the tissue water status, i.e. by retaining water during a misty, humid day, whereas, the vertical-to-horizontal distance ratio (0.25) of the abaxial surface indicates a decline in water retention on the outer petal surfaces.
It appears that cuticular microfolding of adaxial, epidermal site of the small corollas of L. arvensis increases in size the surface area of adaxial epidermal cells and aids optical and adhesive properties of petal surfaces of the short-lived flowers. The cuticular relief, representing an imprint of structural epidermal features at the nanoscale level, displays a higher horizontal-to-vertical distance ratio at the adaxial surface (9.63) than that at the abaxial surface (4.06). Vertical distances between folds estimated on the adaxial and the abaxial surfaces of petals of L. arvensis are comparable to the sub-wavelength regimen (Table ). Nanometric surface structures much smaller than the visible wavelength spectrum can be much more effective in reflecting radiation of shorter rather than longer wavelengths and producing blue colours, which could favour attraction of insect pollinators (Papiorek, Rohde, and Lunau Citation2013). Floral tissues exhibit blue colours through modifications of pigments and structural traits (Lee Citation2007; Yoshida, Mori, and Kondo Citation2009; Vignolini et al. Citation2013).
The surface roughness of the adaxial petal surfaces of L. arvensis (39 nm) may aid the capture of low angle light by the tissues that enhances the brilliance of flowers (Brodersen and Vogelmann Citation2007); this may be advantageous for the proximate to the ground open corollas, under ambient conditions. Hence, petal nanosculpturing may result in a coloured appearance with a greater reflectance spectrum and colour stability for a (relatively) longer-lasting period, in comparison to the pigmented coloration that fades faster with petal desiccation and loss of turgor (Mamareli and Rhizopoulou Citation2009; Glover and Whitney Citation2010).
Spectral reflectance and transmission of incident light falling on petals of L. arvensis were inversely related to the absorption (Figure A). Absorption, reflectance and transmission in fresh, intact petals were found to be approximately 35% between 360 and 440 nm. The absorption increased to 80% from 580 to 640 nm, and a maximum was detected in the 620–630 nm range (Figure B), whereas transmission reduced to 10% (Figure A). Absorption that varies from 35% at 490 nm to 75% at 550 nm (Figure B), indicates anthocyanins confined to petal lobes of L. arvensis (Ishikura Citation1978; Freyre and Griesbach Citation2004). It is expected that convexly shaped, adaxial epidermal cells of petals enhance the proportion of incident light that enters the tissues and can be absorbed by pigments (Kay, Daoud, and Stirton Citation1981; Bone, Lee, and Norman Citation1985; Vogelmann Citation1993; Pfündel, Agati, and Zerovic Citation2006; Lee Citation2007; Argiropoulos and Rhizopoulou Citation2013; Gkikas, Argiropoulos and Rhizopoulou Citation2015); light passing through epidermal cells interacts with pigments, which are involved in defence, protection and signalling (Gorton and Vogelmann Citation1996; Miller, Owens, and Rørslett Citation2011). On one hand, absorption in the 520–640 nm range produces blue colours that are the preferred attractant to insect pollinators (Kay, Daoud, and Stirton Citation1981; Morawetz et al. Citation2013). On the other hand, reflectance was low (< 10%) in the yellow-orange spectral range (Figure A). Elevated reflectance was detected at 300–450 nm and 670–700 nm, which is in agreement with results from other Lysimachia species (Schäffler, Balao, and Dötterl Citation2012). The absorbance of pigmented solutions of petal extracts was found to be substantially lower than that of intact petals, in the 520–620 nm range, and this could be attributed to the effect of some structural elements of petals.
Petals of L. arvensis seem to be UV protected by means of high absorption (> 80%) in the UV wavelength range (Frohlich Citation1972; Ishikura Citation1978; Gronquist et al. Citation2001; Jacobs, Koper, and Ursem Citation2007; Koski and Ashman Citation2014; Llorens et al. Citation2015; Papiorek et al. Citation2015). The UV absorptive, dark patch in the central part of the flower contrasts against the mid-part of the lobe and the periphery; this is shown to be a feature of flower recognition and a guide to floral visitors, which concomitantly preserve pollen grains from injurious effects of UV radiation (Chittka et al. Citation1994; Lunau Citation2000; Moneim, Atta, and Shehata Citation2003; Garcia et al. Citation2014).
Notes on contributors
Sophia Rhizopoulou is associate Professor of Plant Environmental Physiology in the National and Kapodistrian University of Athens (Department of Biology) and has published numerous research articles and books related to plant life in the Mediterranean region [see: http://publicationslist.org/sophia.rhizopoulou].
Emmanuel Spanakis is researcher at the University of Crete (Department of Materials Science and Technology, and Affiliated member of the Institute of Electronic Structure and Laser in Crete.
Apostolos Argiropoulos is a post-PhD fellow in the National and Kapodistrian University of Athens.
Acknowledgments
The research was supported by the grant PENED 03ED174 to S.R., co-financed by European Social Fund-ESF and the Greek Ministry of Research and Innovation.
References
- Aldasoro, J.J., C. Aedo, and C. Navarro. 2000. “Insect attracting structures on Erodium petals (Geraniaceae).” Plant Biology 2: 471–481.
- Anderberg, A. A., U. Manns, and M. Källersjö. 2007. “Phylogeny and floral evolution of the Lysimachieae (Ericales, Myrsinaceae): evidence from ndhF sequence data.” Willdenowia 37: 407–421.
- Argiropoulos, A., and S. Rhizopoulou. 2012a. “Topography and nanosculpture of petals’ surfaces of short-lived flowers of the wild species Cistus creticus, Cistus salviifolius, Eruca sativa and Sinapis arvensis.” Botanical Studies 53: 479–488.
- Argiropoulos, A., and S. Rhizopoulou. 2012b. “Micromorphology of petals of the invasive weed Oxalis pes-caprae.” Weed Biology and Management 12: 47–52.
- Argiropoulos, A., and S. Rhizopoulou. 2013. “Morphological features of petals of Nerium oleander L.” Plant Biosystems 147: 638–644.
- Baird, E., N. Boeddeker, M. R. Ibbotson, and M. V. Srinivasan. 2013. “A universal strategy for visually guided landing.” Proceedings of the National Academy of Sciences, USA 110: 18686–18691.
- Barthlott, W. 1981. “Epidermal and seed surface characters of plants: systematic applicability and some evolutionary aspects.” Nordic Journal of Botany 1: 345–355.
- Bergmeier, E., and A. Strid. 2014. “Regional diversity, population trends and threat assessment of the weeds of traditional agriculture in Greece.” Botanical Journal of the Linnean Society 175: 607–623.
- Bhushan, B., and E. K. Her. 2010. “Fabrication of superhydrophobic surfaces with high and low adhesion inspired from rose petal.” Langmuir 26: 8207–8217.
- Bone, R. A., D. W. Lee, and J. M. Norman. 1985. “Epidermal cells functioning as lenses in leaves of tropical rain-forest shade plants.” Applied Optics 24: 1408–1412.
- Brockett, B. L., and T. S. Cooperrider. 1983. “The Primulaceae of Ohio.” Castanea 48: 37–40.
- Brodersen, C. R., and T. C. Vogelmann. 2007. “Do epidermal lens cells facilitate the absorptance of diffuse light?” American Journal of Botany 94:1061–1066.
- Brütting, C., K. Wesche, S. Meyer, and I. Hensen. 2012. “Genetic diversity of six arable plants in relation to their Red List status.” Biodiversity and Conservation 21: 745–761.
- Buschhaus, C., D. Hager, and R. Jetter. 2015. “Wax layers on Cosmos bipinnatus petals contribute unequally to total petal water resistance.” Plant Physiology 167: 80–88.
- Chimona, C., A. Stamellou, A. Argiropoulos, and S. Rhizopoulou. 2012. “Study of variegated and white flower petals of Capparis spinosa expanded at dusk in arid landscapes.” Journal of Arid Land 4: 171–179.
- Chittka, L., A. Shmida, N. Troje, and R. Menzel. 1994. “Ultraviolet as a component of flower reflections and the colour perception of hymenoptera.” Vision Research 34: 1489–1508.
- Chrtek, J., and J. Osbornová-Kosinová. 1986. “Notes on the synanthropic plants of Egypt: 2. Anagallis arvensis s. l. (Primulaceae).” Folia Geobotanica et Phytotaxonomica 21: 405–421.
- Clegg, M. T., and M. L. Durbin. 2003. “Tracing floral adaptations from ecology to molecules.” Nature Reviews Genetics 4: 206–215.
- de Foucault, B., M. El Beyrouthy, and N. Arnold-Apostolides. 2013. “Some results of recent phytosociological investigations in Central Lebanon.” Acta Botanica Gallica 160: 65–75.
- Domínguez, E., J. A. Heredia-Guerrero, and A. Heredia. 2011. “The biophysical design of plant cuticles: an overview.” New Phytologist 189: 938–949.
- Elzinga, J. A., A. Atlan, A. Biere, L. Gigord, A. E. Weis, and G. Bernasconi. 2007. “Time after time: flowering phenology and biotic interactions.” Trends in Ecology & Evolution 22: 432–439.
- Fahn, A. 1979. Secretory Tissues in Plants. London: Academic Press.
- Farré-Armengol, G., I. Filella, J. Llusià, U. Niinemets, and J. Peñuelas. 2014. “Changes in floral bouquets from compound-specific responses to increasing temperatures.” Global Change Biology 20: 3660–3669.
- Fernandez, I., and F. Garcia-Martin. 1985. “Morfología polínica de la familia Primulaceae en Andalucía Occidental.” Anales de la Asociación de Palinólogos de Lengua Española 2: 107–115.
- Freyre, R., and R. J. Griesbach. 2004. “Inheritance of flower color in Anagallis monelli L.” HortScience 39: 1220–1223.
- Frohlich, M. W. 1972. “Appearance of vegetation in ultraviolet light: Absorbing flowers, reflecting backgrounds.” Science 194 (4267): 839–841.
- Gale, R. M. O., and S. J. Owens. 1983. “Cell distribution and surface morphology in petals, androecia and styles of Commelinaceae.” Botanical Journal of the Linnean Society 87: 247–262.
- Garcia, J. E., A. D. Greentree, M. Shrestha, A. Dorin, and A. G. Dyer. 2014. “Flower colours through the lens: Quantitative measurement with visible and ultraviolet digital photography.” PLoS ONE 9 (5): e96646.
- Gibbs, P. E. 2014. “Late-acting self-incompatibility – the pariah breeding system in flowering plants.” New Phytologist 203: 717–734.
- Gibbs, P. E., and S. Talavera. 2001. “Breeding system studies with three species of Anagallis (Primulaceae): self-incompatibility and reduced female fertility in A. monelli L.” Annals of Botany 88: 139–144.
- Gkikas, D., A. Argiropoulos, and S. Rhizopoulou. 2015. “Epidermal focusing of light and modelling of reflectance in floral-petals with conically shaped epidermal cells.” Flora - Morphology, Distribution, Functional Ecology of Plants 212: 38–45.
- Glas, J. J., B. C. Schimmel, J. M. Alba, R. Escobar-Bravo, R. C. Schuurink, and M. R. Kant. 2012. “Plant glandular trichomes as targets for breeding or engineering of resistance to herbivores.” International Journal of Molecular Sciences 13: 17077–17103.
- Glover, B. J., and H. M. Whitney. 2010. “Structural colour and iridescence in plants: the poorly studied relations of pigment colour.” Annals of Botany 105: 505–511.
- Glover, B. J., R. H. Walker, E. Moyroud, and S. F. Brockington. 2013. “How to spot a flower.” New Phytologist 197: 687–689.
- Gorton, H. L., and T. C. Vogelmann. 1996. “Effects of epidermal cell shape and pigmentation on optical properties of Antirrhinum petals at visible and ultraviolet wavelengths.” Plant Physiology 112: 879–888.
- Green, P. B., A. Havelange, and G. Bernier. 1991. “Floral morphogenesis in Anagallis: Scanning-electron-micrograph sequences from individual growing meristems before, during, and after the transition to flowering.” Planta 185: 502–512.
- Gronquist, M., A. Bezzerides, A. Attygalle, J. Meinwald, M. Eisner, and T. Eisner. 2001. “Attractive and defensive functions of the ultraviolet pigments of a flower (Hypericum calycinum).” Proceedings of the National Academy of Sciences 8: 13745–13750.
- Hao, G., Y. M. Yuan, C. M. Hu, X. J. Ge, and N. X. Zhao. 2004. “Molecular phylogeny of Lysimachia (Myrsinaceae) based on chloroplast trnL–F and nuclear ribosomal ITS sequences.” Molecular Phylogenetics and Evolution 31: 323–339.
- Harris, S. 2007. The Magnificent Flora Graeca. How the Mediterranean came to the English Garden. Oxford: The Bodleian Library.
- Hernández, L., A. Havelange, G. Bernier, and P. B. Green. 1991. “Growth behavior of single epidermal cells during flower formation: Sequential scanning electron micrographs provide kinematic for Anagallis.” Planta 185: 139–147.
- Imhoff, C. H., A. Lecharny, R. Jacques, and J. Brulfert. 1979. “Two phytochrome-dependent processes in Anagallis arvensis L.: flowering and stem elongation.” Plant Cell & Environment 2: 67–72.
- Ishikura, N. 1978. “Light absorption patterns of anthocyanin-containing cells.” Plant Cell Physiology 19: 887–893.
- Jacobs, J. F., G. J. M. Koper, and W. N. J. Ursem. 2007. “UV protective coatings: A botanical approach.” Progress in Organic Coatings 58: 66–171.
- Javelle, M., V. Vernoud, P. M. Rogowsky, and G. C. Ingram. 2011. “Epidermis: The formation and functions of a fundamental plant tissue.” New Phytologist 189: 17–39.
- Jiménez-López, J., M. Talavera, P. L. Ortiz, and M. Arista. 2015. “Nuclear microsatellite primers in the annual herb Lysimachia arvensis (Myrsinaceae) and closely related taxa.” Biochemical Systematics and Ecology 58: 242–246.
- Kay, Q. O. N., H. S. Daoud, and C. H. Stirton. 1981. “Pigment distribution, light reflection and cell structure in petals.” Botanical Journal of Linnean Society 83: 57–89.
- Kevan, P. G., and M. A. Lane. 1985. “Flower petal microtexture is a tactile cue for bees.” Proceedings of the National Academy of Sciences 82: 4750–4752.
- Koch, K., M. Bennemann, H. F. Bohn, D. C. Albach, and W. Barthlott. 2013. “Surface microstructures of daisy florets (Asteraceae) and characterization of their anisotropic wetting.” Bioinspiration & Biomimetics 8: 036005.
- Koski, M. H., and T. L. Ashman. 2014. “Dissecting pollinator responses to a ubiquitous ultraviolet floral pattern in the wild.” Functional Ecology 28: 868–877.
- Lee, D. 2007. Nature’s Palette, the Science of Plant Color. Chicago, IL: The University of Chicago Press.
- Lehrer, M., M. V. Srinivasan, and S. W. Zhang. 1990. “Visual edge detection in the honeybee and its chromatic properties.” Proceedings of the Royal Society of London B 238: 321–330.
- Llorens, L., F. R. Badenes-Pérez, R. Julkunen-Tiitto, C. Zidorn, A. Fereres, and M. A. Jansen. 2015. “The role of UV-B radiation in plant sexual reproduction.” Perspectives in Plant Ecology, Evolution and Systematics 17: 243–254.
- Lunau, K. 2000. “The ecology and evolution of visual pollen signals.” In Pollen and Pollination, edited by A. Dafni, M. Hesse and E. Pacini, 89–111. Vienna: Springer.
- Ma, Y., and J. Sun. 2009. “Humido-and thermo-responsive free-standing films mimicking the petals of the morning glory flower.” Chemistry of Materials 21: 898–902.
- Mamareli, P., and S. Rhizopoulou. 2009. “Study of flowering Cichorium intybus L.” Poster presented at the 11th Symposium of the Hellenic Botanical Society, Athens. Accessed October 8—11. http://users.uoa.gr/~cthanos/Papers/11EBE.pdf, 111.
- Manns, U., and A. A. Anderberg. 2007. “Relationships of Anagallis foemina and A. arvensis (Myrsinaceae): New insights inferred from DNA sequence data.” Molecular Phylogenetics and Evolution 45: 971–980.
- Manns, U., and A. A. Anderberg. 2009. “New combinations and names in Lysimachia (Myrsinaceae) for species of Anagallis, Pelletiera and Trientalis.” Willdenowia 39: 49–54.
- Manns, U., and A. A. Anderberg. 2011. “Biogeography of ‘tropical Anagallis’ (Myrsinaceae) inferred from nuclear and plastid DNA sequence data.” Journal of Biogeography 38: 950–961.
- Marsden-Jones, E. M., and F. E. Weiss. 1960. “The genetics and pollination of Anagallis arvensis subsp. arvensis and Anagallis arvensis subsp. foemina.” Proceedings of the Linnean Society of London 71: 27–29.
- Martin, C., and B. J. Glover. 2007. “Functional aspects of cell patterning in aerial epidermis.” Current Opinion in Plant Biology 10: 70–82.
- McNally, H. A., and R. B. Borgens. 2004. “Three-dimensional imaging of living and dying neurons with atomic force microscopy.” Journal of Neurocytology 33: 251–258.
- Miller, R., S. J. Owens, and B. Rørslett. 2011. “Plants and colour: flowers and pollination.” Optics & Laser Technology 43: 282–294.
- Moneim, A., I. A. Atta, and A. A. Shehata. 2003. “On the delimitation of Anagallis arvensis L. (Primulaceae). 1. Evidence based on micromorphological characters, palynological features and karyological studies.” Pakistan Journal of Biological Sciences 6: 29–35.
- Morawetz, L., A. Svoboda, J. Spaethe, and A. G. Dyer. 2013. “Blue colour preference in honey bees distract visual attention for learning closed shapes.” Journal of Comparative Physiology A 199: 817–827.
- Ortiz, P. L., R. Berjano, M. Talavera, L. Rodríguez-Zayas, and M. Arista. 2015. “Flower colour polymorphism in Lysimachia arvensis: How is the red morph maintained in Mediterranean environments?” Perspectives in Plant Ecology, Evolution and Systematics 17: 142–150.
- Papiorek, S., K. Rohde, and K. Lunau. 2013. “Bees’ subtle colour preferences: How bees respond to small changes in pigment concentration.” Naturwissenschaften 10: 633–643.
- Papiorek, S., R. R. Junker, I. Alves-dos-Santos, G. A. R. Melo, L. P. Amaral-Neto, M. Sazima, M. Wolowski, L. Freitas, and K. Lunau. 2015. “Bees, birds and yellow flowers: Pollinator-dependent convergent evolution of UV-patterns.” Plant Biology. doi:10.1111/plb.12322.
- Pfündel, E. E., G. Agati, and Z. G. Zerovic. 2006. “Optical properties of plant surfaces.” In Biology of the Plant Cuticle, edited by M. Riederer and C. Müller, 216–247. Oxford: Blackwell.
- Possobom, C. C. F., E. Guimarães, and S. R. Machado. 2015. “Structure and secretion mechanisms of floral glands in Diplopterys pubipetala (Malpighiaceae) and a neotropical species.” Flora – Morphology. Distribution, Functional Ecology of Plants 211: 26–39.
- Qian, J., F. Chen, X. Zhao, and Z. Chen. 2011. “China rose petal as biotemplate to produce two-dimensional ceria nanosheets.” Journal of Nanoparticle Research 13: 7149–7158.
- Raine, N. E., and L. Chittka. 2007. “The adaptive significance of sensory bias in a foraging context: floral colour preferences in the bumblebee Bombus terrestris.” PLoS ONE 2 (6): e556.
- Rhizopoulou, S., A. Lykos, P. Delipetrou, and I. Vallianatou. 2012. “Living collection of Flora Graeca Sibthorpiana: from the folios of the monumental edition to the beds of a botanic garden in Greece.” Sibbaldia 10: 171–196.
- Rhizopoulou, S., and H. Pantazi. 2015. “Constraints on floral water status of successively blossoming Mediterranean plants under natural conditions.” Acta Botanica Gallica 162: 97–102.
- Schäffler, I., F. Balao, and S. Dötterl. 2012. “Floral and vegetative cues in oil-secreting and non-oil-secreting Lysimachia species.” Annals of Botany 110: 125–138.
- Shimomura, M. 2010. “The new trends in next generation biomimetics material technology: learning from biodiversity.” Quarterly Review 3: 53–75.
- Šilc, U., Z. Lososová, and S. Vrbničanin. 2014. “Weeds shift from generalist to specialist: narrowing of ecological niches along a north-south gradient.” Preslia 86: 35–46.
- Simpson, B. B., and J. L. Neff. 1983. “Floral Biology and floral rewards of Lysimachia (Primulaceae).” American Midland Naturalist 110: 249–256.
- Stratakis, E., V. Zorba, M. Barberoglou, C. Fotakis, and G. A. Shafeev. 2009. “Laser writing of nanostructures on bulk Al via its ablation in liquids.” Nanotechnology 20: 105303.
- Taneda, H., A. Watanabe-Taneda, R. Chhetry, and H. Ikeda. 2015. “A theoretical approach to the relationship between wettability and surface microstructures of epidermal cells and structured cuticles of flower petals.” Annals of Botany 115: 923–937.
- Telford, A. M., B. S. Hawkett, C. Such, and C. Neto. 2013. “Mimicking the wettability of the rose petal using self-assembly of waterborne polymer particles.” Chemistry of Materials 25: 3472–3479.
- Tooke, F., and N. H. Battey. 2010. “Temperate flowering phenology.” Journal of Experimental Botany 61: 2853–2862.
- Uchida, K., N. Nishikawa, N. Izumi, S. Yamazoe, H. Mayama, Y. Kojima, S. Yokojima, S. Nakamura, K. Tsujii, and M. Irie. 2010. “Phototunable diarylethene microcrystalline surfaces: lotus and petal effects upon wetting.” Angewandte Chemie 49: 5942–5944.
- van Doorn, W. G., and C. Kamdee. 2014. “Flower opening and closure: an update.” Journal of Experimental Botany 65: 5749–5757.
- Verma, B. K. 1972. “Epidermal structure and ontogeny of stomata in leaves of Anagallis arvensis L.” Annals of Botany 36: 857–860.
- Vignolini, S., E. Moyroud, B. J. Glover, and U. Steiner. 2013. “Analysing photonic structures in plants.” Journal of the Royal Society Interface 10: 20130394.
- Vitarelli, N. C., R. Riina, M. B. R. Caruzo, I. Cordeiro, J. Fuertes-Aguilar, and R. M. Meira. 2015. “Foliar secretory structures in Crotoneae (Euphorbiaceae): Diversity, anatomy, and evolutionary significance.” American Journal of Botany 102: 833–847.
- Vogelmann, T. C. 1993. “Plant tissue optics.” Annual Review of Plant Physiology and Plant Molecular Biology 44: 231–251.
- Vrachnakis, T. G. 2002. “On the epidermal elements of Origanum calcaratum Juss. (Labiatae).” Phyton 42: 39–67.
- Wagner, P., R. Fürstner, W. Barthlott, and C. Neinhuis. 2003. “Quantitative assessment to the structural basis of water repellency in natural and technical surfaces.” Journal of Experimental Botany 54: 1295–1303.
- Whitney, H. M., and B. J. Glover. 2007. “Morphology and development of floral features recognised by pollinators.” Arthropod-Plant Interactions 1: 147–158.
- Whitney, H. M., R. Poetes, U. Steiner, L. Chittka, and B. J. Glover. 2011. “Determining the contribution of epidermal cell shape to petal wettability using isogenic Antirrhinum lines.” PloS ONE 6 (3): e17576.
- Yoshida, K., M. Mori, and T. Kondo. 2009. “Blue flower color development by anthocyanins: from chemical structure to cell physiology.” Natural Product Reports 26: 884–915.