Abstract
The aim of this in silico investigation was to examine the influence of two ceramic inlay materials with different cavity designs on stresses in the inlay. Finite-element analysis and three-dimensional modelling were used to examine the stress in ceramic inlays resulting from a 250-N point load on occlusal surfaces. The adhesion properties and von Mises stress values in the enamel, dentin, ceramic materials and cement linings were simulated. Two ceramic inlay materials: porcelain ceramic (Empress II, Ivoclar Vivadent, Liechtenstein) and zirconia ceramic (ICE Zirkon, Zirkonzahn SRL, Gais, Italy), as well as two cavity corner designs: rectangular and rounded, were evaluated. The obtained von Mises stress results indicated that the maximum and minimum forces were concentrated in the enamel and dentin, respectively. The stress values in the dentin and inlay material were similar in the porcelain ceramic and zirconia ceramic groups. However, in the enamel, the stress values in the zirconia ceramic group were significantly lower than those in the porcelain ceramic group. Additionally, cavities with rounded corners were subject to significantly less stress compared to those with rectangular corners. Thus, the zirconia ceramic inlay demonstrated better performance under applied stress, based on the reduced stress values in the tooth structure. Cavities with rounded corners showed less stress than those with rectangular corners, which could be attributed to the enhanced stress distribution capabilities of rounded corners.
Introduction
Indirect restorations, as they are fabricated under ideal laboratory conditions, have more desirable physical properties than direct restorations.[Citation1,Citation2] All-ceramic restorations have been approved for the treatment of inlay cavities. These ceramics succeed in restoring the natural morphology of the teeth to achieve a good visual appearance.[Citation3,Citation4] Fracturing of the ceramic inlays is an acceptable complication that can lead to failure of the restoration. The quality of the adhesive technique, the material suitability and the form and dimension of the cavity preparation affect the likelihood of long-term success. For a successful restoration, suitable cavity design is of great importance in addition to the material selected.[Citation4–6]
The use of ceramics with adhesive techniques allows the tooth structure to be preserved and permits more aesthetic restorations.[Citation7] Zirconia or zirconium dioxide, a white crystalline material, is commonly used in dentistry. Zirconia has mechanical properties similar to those of metals and colour similar to the natural colour of teeth. Zirconia crystals can be configured as three patterns: monoclinic, cubic and tetragonal.[Citation8] Tetragonal zirconia, or yttrium-stabilized zirconia, has become available in dentistry owing to the use of computer-aided design/computer-aided manufacturing (CAD/CAM) or copy-milling techniques. Yttrium-stabilized zirconia exhibits perfect mechanical performance and outstanding strength and fracture resistance compared with other ceramics.[Citation9,Citation10]
Porcelain ceramic inlays are composed primarily of leucite-reinforced ceramics.[Citation11] This type of ceramic is characterized by an elastic modulus similar to that of enamel.[Citation12–14] The disadvantages of leucite-reinforced ceramic are its hardness (which is greater than that of enamel), low fracture resistance and low fatigue strength.[Citation15,Citation16]
Because restoration success depends on the stress and the physical properties of the materials used, restoration materials must be evaluated under compressive loading to determine their fatigue strength.[Citation17–21] Three-dimensional (3D) finite-element (FE) analysis has been used extensively in dental biomechanical studies to produce detailed and realistic animate or inanimate structures.
The model resulting from 3D FE analysis is divided into mathematically meaningful parts or elements. The elements are connected by ‘nodes’ of various geometric shapes. Certain nodes have a fixed displacement range or load value. A physical change in one element (e.g., an applied force or load) is transmitted to the other elements by the nodes, allowing the determination of stress/strain information for the particular system under investigation.[Citation22]
von Mises stress is a combination of normal and shear stresses occurring in all directions. This stress has to be considered when evaluating the appropriate restorative material to use, as well as the tooth tissue damage that may occur. By doing so, it is possible to determine whether or not permanent deformation or breakage has occurred in the material.[Citation23]
The purpose of the present study was to analyse the biomechanical performance of porcelain lithium disilicate and yttrium-stabilized zirconia ceramic inlays, for two cavity base designs, under a 250-N point load applied to the occlusal surface of the first molar. The adhesion properties and von Mises stress values in the enamel, dentin, ceramic materials and cement linings were simulated using 3D FE analysis.
Materials and methods
In this in silico study, stress analysis was performed on the first molar teeth, using a stress analysis software (ANSYS Rel.6.0, ANSYS Inc., Houston, TX, USA). The FE analysis model consisted of dental fillings, enamel and dentin. The tooth size and shape were compared with data from ‘Wheeler's dental anatomy, physiology and occlusion.’[Citation24] A computer model of the mandibular molar was generated. An additional inlay-shaped volume, having a 3.5 mm wide and 2.5 mm deep isthmus, was created using the FE analysis software preprocessor. The cavity preparation was created by deleting the inlay volume. A tooth model with an inlay-prepared cavity was obtained (). Inlays with a 0.1-mm cement layer were overlapped on the models in a similar way. The inlays were bonded to the tooth structure with composite resin cement.
Two ceramic restoration materials were used: zirconia ceramic (ICE Zirkon Zirkonzahn SRL, Gais, Italy) and porcelain ceramic (Empress II, Ivoclar Vivadent-Liechtenstein). At the interface, luting cement (Variolink II, Ivoclar Vivadent, Liechtenstein) and an adhesive agent (Optibond FL, Kerr, USA) were used.
lists the elasticity modules and Poisson's ratios of enamel,[Citation12] dentin,[Citation25] zirconia ceramic,[Citation26] porcelain ceramic,[Citation27] adhesive agent [Citation28] and luting cement.[Citation29]
Table 1. Materials used to model restoration of molars with porcelain and zirconia ceramic inlays.
The mandibular molar tooth was modelled as a three-layered structure consisting of enamel, dentin and filling material. Two different Class I cavity corner forms were used: cavities with rectangular and rounded corners (). Different restorative materials and the hard tissues of the tooth with different cavity corner forms were loaded under a force of 250 N applied vertically (). The 250-N load was spread over three areas of the tooth. In total, 12 models were prepared and evaluated. Maximum and minimum stresses were calculated for each model separately.
Figure 3. Vertical loading force of 250 N. Mesial loading of 250/3 N (A). Central loading of 250/3 N (B). Distal loading of 250/3 N (C).
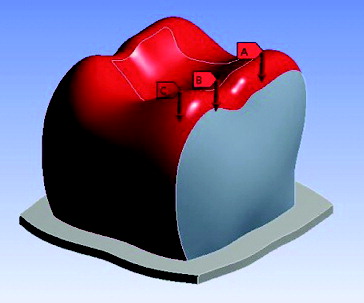
To perform this study, we used an Intel Pentium D CPU 3.00 GHz processor, with a 250 GB hard disk and 3.00 GB of RAM. The computer was equipped with the Windows XP Professional operation system (version 2002 with Service Pack 3). FE stress analysis and 3D model creation were performed using a laser scanner (NextEngine, Inc., Santa Monica, CA), 3D modelling software (Rhinoceros 4.0, McNeel North America, Seattle, WA, USA) and the stress analysis software (ANSYS Rel.6.0, ANSYS Inc., Houston, TX, USA).
Results and discussion
In this study, the maximum and minimum stress values for enamel, dentin and dental fillings for different cavity corner designs restored with different ceramic inlays were compared under vertical occlusal forces in 12 models. The model exhibiting the lowest stress value corresponded to the preferred material for restoration.
The results shown in and indicate that minimal forces were concentrated in the dentin, whereas maximum forces were present in the enamel. The stress values in the dentin and inlay material for the porcelain ceramic and zirconia ceramic groups were similar. However, in the enamel, the stress values in the zirconia ceramic group were considerably lower than those in the porcelain ceramic group. This indicates that the structure of zirconia ceramic retains the stress inside (i.e., less stress is transferred through the tooth structure). Also, the results indicate the enhanced stress distribution by the rounded corners ().
Table 2. Maximum and minimum stress values after force application (MPa).
Because the values obtained by FE stress analysis are variances that occur as a result of non-mathematical calculations, statistical analysis was not performed. Instead, careful review and analysis of the stress distributions were carried out.
Ceramic inlay restorations offer optimal aesthetics, biocompatibility and longevity. The use of adhesive bonding materials and resin composites improves the clinical success of the ceramic inlay restoration and significantly increases the fracture resistance. Zirconium can be used safely in inlay and onlay restorations, due to its high durability and superior detail ability.[Citation30]
The elasticity modulus describes the relative stiffness of the materials within their elastic range and is determined by tensile, bending, compressive and indentation techniques.[Citation31] The use of low-modulus restorative materials has been shown to facilitate stress release.[Citation32–34] Kramer and Frankenberger [Citation35] showed that the use of ceramic inlays was successful, even for large cavities; the lack of a contact edge for the enamel or the absence of a tubercle did not significantly affect the quality of the restoration.
In the present study, biomechanical analysis of the parametric inlay models was carried out using the FE method, which is used frequently in dental studies [Citation36,Citation37]. Using the FE technique, von Mises stress values were evaluated under loading of two ceramic inlay restorations. Zirconia ceramic exhibited superior durability compared to the porcelain ceramic. Moreover, our results were in agreement with the previous reports that the cavity with rounded corners better distributes the stress under an applied vertical force.[Citation31]
When the inlay materials were evaluated mathematically, stress formation in the supportive tooth structures was low in the zirconia ceramic inlay model (0.26–10.13 MPa). The zirconia ceramic inlay retained the stress inside; less stress was transferred through the tooth structure. The stress values in the porcelain ceramic ranged from 0.31 to 14.52 MPa. In this case, stress accumulation through the tooth structure was denser in the porcelain ceramic inlay model, due to its lower elasticity modulus. Thus, zirconia ceramic inlay may be a more appropriate restoration, especially when the supportive tooth structure is weak.[Citation31]
Changing the cavity corner design appeared to affect only the inlay material (dental filling). The stress values were reduced by almost half in the zirconia and porcelain models with rounded corner cavities compared to those with rectangular formed cavities, indicating better stress distribution. Further in vitro studies, such as stress distribution analysis, laboratory experiments and clinical studies, need to be conducted to more precisely determine the stress distributions of various ceramic restorations in inlay and onlay cavities with different cavity designs.
Conclusions
Within the limitations of this study, the obtained results showed the following. (1) The most preferable inlay restoration material was zirconia ceramic, because its structure held the stress inside, i.e., less stress was transferred through the tooth structure. Zirconia ceramic inlay is especially applicable when the supportive tooth structure is weak. (2) Cavities with rounded corners accumulated less stress and spread the stress more evenly to the base of the cavity than did cavities with rectangular corners. (3) The porcelain ceramic accumulated the most stress, the majority of which was transmitted directly to the tooth. (4) In materials with a low elasticity modulus, stresses formed on the surface; these stresses did not spread to the other parts of the tooth or restoration. Stresses on the surface of the material induced deformation and material wear. In materials with a high elasticity modulus, due to better transmission and distribution of the stresses, less deformation and wear occurred.
Acknowledgements
The authors thank Dr Emre Ari (Dicle University, Department of Mechanical Engineering) for his helpful advice on the FE analysis.
Disclosure statement
The authors declare that they have no competing interests.
References
- Saridag S, Sevimay M, Pekkan G. Fracture resistance of teeth restored with all-ceramic inlays and onlays: an in vitro study. Oper Dent. 2013;38(6):626–634.
- Thompson JY, Bayne SC, Heymann HO. Mechanical properties of a new mica-based machinable glass ceramic for CAD/CAM restorations. J Prosthet Dent. 1996;76(6):619–623.
- Holberg C, Winterhalder P, Wichelhaus A, Hickel R, Huth K. Fracture risk of lithium-disilicate ceramic inlays: a finite element analysis. Dent Mater. 2013;29(12):1244–1250.
- Frankenberger R, Taschner M, Garcia-Godoy F, Petschelt A, Kramer N. Leucite-reinforced glass ceramic inlays and onlays after 12 years. J Adhes Dent. 2008;10:393–398.
- Beier US, Kapferer I, Dumfahrt H. Clinical long-term evaluation and failure characteristics of 1,335 all-ceramic restorations. Int J Prosthodont. 2012;25:70–78.
- Boushell LW, Ritter AV. Ceramic inlays: a case presentation and lessons learned from the literature. J Esthet Restor Dent. 2009;21:77–87.
- Soares CJ, Martins LR, Pfeifer JM, Giannini M. Fracture resistance of teeth restored with indirect-composite and ceramic inlay systems. Quintessence Int. 2004;35(4):281–286.
- Manicone PF, Rossi Iommetti P, Raffaelli L. An overview of zirconia ceramics: basic properties and clinical applications. J Dent. 2007;35(11):819–826.
- Mehl C, Ludwig K, Steiner M, Kern M. Fracture strength of prefabricated all-ceramic posterior inlay-retained fixed dental prostheses. Dent Mater. 2010;26(1):67–75.
- Tinschert J, Natt G, Mohrbotter N, Spiekermann H, Schulze KA. Lifetime of alumina- and zirconia ceramics used for crown and bridge restorations. J Biomed Mater Res B. 2007;80(2):317–321.
- Topala FI, Sandu L, Porojan S, Faur N. 3D modeling and simulation of inlay restored posterior teeth. TMJ. 2010;60(1):69–73.
- Dejak B, Mlotkowski A. Three-dimensional finite element analysis of strength and adhesion of composite resin versus ceramic inlays in molars. J Prosthet Dent. 2008;99(2):131–140.
- Habelitz S, Marshall SJ, Marshall GW Jr, Balooch M. Mechanical properties of human dental enamel on the nanometre scale. Arch Oral Biol. 2001;46:1731–1783.
- Albakry M, Guazzato M, Swain MV. Biaxial flexural strength, elastic moduli, and x-ray diffraction characterization of three pressable all-ceramic materials. J Prosthet Dent. 2003;89:374–380.
- Gorman CM, McDevitt WE, Hill RG. Comparison of two heat-pressed all-ceramic dental materials. Dent Mater. 2000;16:389–395.
- Ohyama T, Yoshinari M, Oda Y. Effects of cyclic loading on the strength of all-ceramic materials. Int J Prosthodont. 1999;12:28–37.
- Eyupoglu TA, Onal B, Erdilek N, Goren B, Ergucu Z. Evaluation of the mechanical performance of inlay restorations on molar teeth: a 3-D finite element analysis. GÜ Diş Hek Fak Derg. 2008;25(1):27–33.
- Ausiello P, Rengo S, Davidson CL, Watts DC. Stress distributions in adhesively cemented ceramic and resin-composite Class II inlay restorations: a 3D-FEA study. Dent Mater. 2004;20:862–872.
- Bott B, Hannig M. Effect of different luting materials on the marginal adaptation of Class I ceramic inlay restorations in vitro. Dent Mater. 2003;19:264–269.
- Dietschi D, Moor L. Evaluation of the marginal and internal adaptation of different ceramic and composite inlay systems after an in vitro fatigue test. J Adhes Dent. 1999;1:41–56.
- Shor A, Nicholls JI, Phillips KM, Libman WJ. Fatigue load of teeth restored with bonded direct composite and indirect ceramic inlays in MOD class II cavity preparations. Int J Prosthodont. 2003;16:64–69.
- Sonugelen M, Artuc C. Ağız Protezleri ve Biyomekanik, [Oral Prostheses and Biomechanics]. İzmir: Ege Univ Diş Hek Fak Yayınları; 2002. p. 3–8. Turkish.
- Beer FP, Johnston ER. Mechanics of materials. New York (NY): McGraw-Hill; 1981. p. 15–17.
- Ash MM, Nelson N. Wheeler's dental anatomy, physiology, and occlusion. 8th ed. Philadelphia (PA): Saunders; 2002. p. 297–306.
- Arola D, Galles LA, Sarubin MF. A comparison of the mechanical behavior of posterior teeth with amalgam and composite MOD restorations. J Dent. 2001;29: 63–73.
- Sannino G, Pozzi A, Schiavetti R, Barlattani A. Stress distribution on a three-unit implant-supported zirconia framework. A 3D finite element analysis and fatigue test. Oral Implantol (Rome). 2012;5(1):11–20.
- Rubin C, Krishnamurthy N, Capilouto E, Yi H. Stress analysis of the human tooth using a three-dimensional finite element model. J Dent Res. 1983;62:82–86.
- Tortopidis D, Lyons MF, Baxendale RH, Gilmour WH. The variability of bite force measurement between sessions, in different positions within the dental arch. J Oral Rehabil. 1998;25:681–686.
- Bader JD, Shugars DA, Sturdevant JR. Consequences of posterior cusp fracture. Gen Dent. 2004;52:128–131.
- Blatz MB. Long term clinical success of all ceramic posterior restorations. Quintessence Int. 2002;33:415–426.
- Belli S, Eskitaşcioglu G, Eraslan O, Senawongse P, Tagami J. Effect of hybrid layer on stress distribution in a premolar tooth restored with composite or ceramic inlay: an FEM study. J Biomed Mater Res B. 2005;74(2):665–668.
- Kemp-Schölte CM, Davidson CL. Complete marginal seal of class V resin restorations effected by increased flexibility. J Dent Res. 1990;69:1240–1243.
- Davidson CL, Abdalla AI. Effect of thermal and mechanical load cycling on the marginal integrity of Class II resin composite restorations. Am J Dent. 1993;6:39–42.
- Dauvillier BS. Visco-elastic parameters of dental restorative materials during setting. J Dent Res. 2000; 79:818–823.
- Kramer N, Frankenberger R. Clinical performance of bonded leucite- reinforced glass ceramic inlays and onlays after eight years. Dent Mater. 2005;21:262–271.
- Magne P, Perakis N, Belser UC, Krejci I. Stress distribution of inlay-anchored adhesive fixed partial dentures: a finite element analysis of the influence of restorative materials and abutment preparation design. J Prosthet Dent. 2002;87:516–527.
- Ona M, Watanabe C, Igarashi Y, Wakabayashi N. Influence of preparation design on failure risks of ceramic inlays: a finite element analysis. J Adhes Dent. 2011;13:367–373.