Abstract
The antifungal activity of Streptomyces griseorubens E44G against Rhizoctonia solani, the causal agent of root rot disease of corn, was investigated. The mycelial growth of R. solani was inhibited by S. griseorubens E44G, indicating that it has an antifungal potential. The antagonist, S. griseorubens E44G, was detected to have proteolytic activity, using the method of casein hydrolysis. Moreover, the protease production was optimized under submerged conditions. The purification and precipitation of protease were achieved by ammonium sulphate and Sephadex G-100 gel filtration chromatography. Protease activity was detected spectrophotometrically based on the production of tyrosine. The molecular weight of the enzyme (35 kDa) was determined by sodium dodecyl sulphate polyacrylamide gel electrophoresis . The optimum activity of the enzyme was detected at pH 8.5 and 60 °C. The results indicated that the enzyme was thermostable and retained full activity even after 1 hour of incubation at 60 °C. The purified enzyme substantially inhibited the growth of R. solani, indicating that this enzyme may be actually involved in the antagonistic process.
Introduction
Rhizoctonia solani Kuhn is a soil-borne plant pathogenic fungus with a wide host range (such as corn, potatoes, cereals, sugar beet and cucumber) and worldwide distribution. It causes various plant diseases such as collar rot, root rot, damping off and wire stem. The pathogen is characterized by genetically isolated populations called anastomosis groups.[Citation1] For instance, isolate AG 2-2 - IIIB of R. solani is an aggressive root rot pathogen of corn and sugar beet.[Citation2] Currently, treatment of corn seeds with chemical fungicides is the most effective method of preventing this disease. However, field application of these fungicides has been discouraged owing to their toxic effects on non-target organisms and due to the undesirable changes they inflict upon humans, animals and the environment.[Citation3] Health concerns and environmental hazards associated with the use of chemical fungicides have resulted in an increasing interest in biological control as a promising alternative or a supplemental way of reducing the use of agro-chemicals.[Citation4] Biological control is an effective and safe method of controlling plant diseases using other living organisms.[Citation5]
Many species of actinomycetes, particularly those belonging to genus Streptomyces, are well known as antifungal biocontrol agents that inhibit the growth of several plant pathogenic fungi.[Citation6] Proposed mechanisms may involve physical contact (hyperparasitism) and synthesis of hydrolytic enzymes (e.g. chitinases and proteases), toxic compounds or antibiotics, on one hand, and competition, on the other.[Citation7]
Proteases, in addition to being involved in the antagonistic process, play an important role in biotechnology and are widely used in the tanning industry, in the manufacturing of biological detergents, meat tenderization, peptide synthesis, food industry, pharmaceutical industry and in bioremediation processes.[Citation8–10] Microbes are considered a preferred source of proteases owing to their rapid growth and limited spatial requirements for cultivation and amenability to generation of new enzymes by genetic manipulation for various applications.[Citation11,Citation12]
There is still insufficient information on naturally occurring extracellular proteases produced by actinomycetes. However, the large demand of proteolytic enzymes encourages the search for new enzyme sources with an extended range of applications. Screening of protease producers such as Streptomyces spp. isolated from different environments may lead to the discovery of new alkaline proteases with unique physicochemical characteristics.
In our preliminary studies, we isolated several Streptomyces sp. strains from soil in Saudi Arabia and they acted as antagonists against some plant pathogenic fungi. One of the promising isolates for antifungal activity was identified as Streptomyces griseorubens E44G.[Citation13]
The aim of the present study was to purify and characterize the protease produced by the antagonist S. griseorubens E44G and its antifungal potential against R. solani, the causal agent of root rot disease of corn.
Materials and methods
Micro-organisms
The fungal pathogen was isolated from the roots of naturally diseased corn plants exhibiting typical symptoms of Rhizoctonia root rot disease. The isolated fungus was grown on potato dextrose agar (PDA; Difco, USA) plates and incubated at 28 °C for 4–6 days. Purification of the resulting fungus was done using the hyphal tip technique. The strain was then transferred into slants of PDA and kept at 4 °C for further studies. Pure culture of the isolated fungus was identified according to the cultural properties and morphological and microscopical characteristics.[Citation14]
The used Streptomyces isolate was isolated previously from soil in Saudi Arabia and identified as S. griseorubens E44G according to Bergey's Manual of Determinative Bacteriology [Citation15] based on morphological, biochemical and physiological characteristics. The identification was supported by results from 16S rRNA gene sequencing.[Citation13]
Assay of antifungal activity
A 7 mm diameter disk from a 5-day-old culture of S. griseorubens E44G was placed in the centre of a starch nitrate agar plate inoculated with R. solani. Three replicates were made. The starch nitrate plates were then incubated at 30 °C ± 1 °C. The inhibition zone, if any, was measured in diameter (mm) after 72 h of incubation.
Screening for proteolytic activity
Proteolytic activity was detected by casein hydrolysis on agar plates containing yeast nitrogen base (Difco, USA) medium supplemented with 0.5% casein, 0.5% glucose and 2% agar (w·v−1), pH 7.0.[Citation16] The plates were incubated at 28 °C for 7–8 days. Enzyme activity was indicated by the formation of a clear zone around colonies after precipitation with 1 mol·L−1 HCl solution. A commercial protease solution (Sigma P-4032) at 0.001% (w·v−1) was used as the positive control.
Growth and culture conditions
Cultures of S. griseorubens E44G were grown in Erlenmeyer flasks containing 500 mL of liquid culture medium (w·v−1) [1% glucose, 0.2% peptone, 0.3% yeast extract, 0.1% beef extract, 2.5% wheat bran as a substrate]. The culture was then incubated for 72 h with agitation (150 r·min−1) at 30 °C. At the end of the incubation period, the cell-free extract was obtained by centrifugation at 10,000 r·min−1 for 15 min and the pelleted cellular mass was discarded.
Enzyme activity assay
Protease activity in the culture supernatant was determined using casein as a substrate, according to the method of Tsuchida et al.[Citation17] Enzyme activity was determined by incubating 250 μL of the culture supernatant with 500 μL of 1% (w·v−1) casein sodium salt (Sigma) in 50 mmol·L−1 buffer (pH 7) for 20 min at 40 °C. The reaction was stopped by the addition of 1 mL of 20% (w·v−1) trichloroacetic acid and incubation at room temperature for 15 min. Then, the reaction mixture was centrifuged to separate the non-reacted casein at 10,000 r·min−1 for 5 min. The supernatant was mixed with 2.5 mL of 0.4 mol·L−1 Na2CO3 and 1 mL of 3-fold diluted Folin–Ciocalteu phenol reagent was added. The resulting solution was incubated at room temperature in the dark for 30 min and the absorbance of the blue colour developed was measured at 660 nm against a reagent blank using a tyrosine standard.[Citation18] One unit (U) of enzyme activity was defined as the amount of enzyme that, under the assay conditions described, gives rise to an increase of 0.1 units of absorbance in 1 h at 30 °C.[Citation19]
Determination of protein content
To determine the protein content, a standard curve of protein concentration was generated using Coomassie Brilliant Blue (CBB) and bovine serum albumin (BSA), according to Bradford.[Citation20] The absorbance was plotted against the protein content. The protein content of the unknown sample was calculated from the standard curve.
Enzyme purification
Ammonium sulphate precipitation
Protein content was precipitated in the supernatant by adding dry ammonium sulphate to 70% final concentration. The solution was centrifuged at 8,000 r·min−1 for 20 min. All subsequent steps were carried out at 4 °C. The protein pellet was resuspended in gel permeation buffer (50 mmol·L−1 NaH2PO4, 150 mmol·L−1 NaCl, 1 mmol·L−1 ethylenediaminetetraacetic acid; pH 7), and dialyzed overnight against the same buffer.
Sephadex G-100 gel filtration chromatography
The dialysate was loaded onto a Sephadex G-100 column (2.4 cm × 75 cm) equilibrated with a gel permeation buffer. The flow-rate was 1 mL·min−1 and 4 mL fractions were collected continuously after the starting point of elution (V0) was reached. After screening the fractions for protease activity, the contents of positive tubes corresponding to a single peak were pooled (F10 and F11).
Sodium dodecyl sulphate-polyacrylamide gel electrophoresis (SDS-PAGE)
Protease samples were subjected to electrophoresis in polyacrylamide gels (12 %) under denaturing conditions, according to Laemmli.[Citation21] After electrophoresis, gels were stained with staining solution (10% acetic acid, 25% methanol, 0.25 g·L−1 CBB G-250) overnight and destained in 30%–10% methanol and acetic acid overnight. Standard proteins served as markers for molecular mass (Fermentas). The molecular mass standards were BSA (66.2 kDa), ovalbumin (45 kDa), lactate dehydrogenase (35 kDa), REase Bsp98 (25 kDa), α-lactalbumin (18.4 kDa) and lysozyme (14.4 kDa).
Characterization of protease enzyme
pH stability
The activity of the crude protease was measured at different pH values. The pH was adjusted using the following buffers (0.05 mol·L−1): phosphate (pH 5.0–7.0), Tris-HCl (pH 8.5) and sodium acetate (pH 4.0–6.0). Crude protease (100 µL) was mixed with 2 mL of the buffer and incubated at 37 °C for 30 min, and the activity of the enzyme was measured.
Thermal stability
Thermal stability of the crude enzyme was determined by incubating the enzyme at different temperatures (37, 50, 60, 70 and 80 °C) for 30 min at the optimum pH (8.5) and then the activity of the enzyme was determined.
Assay of antifungal activity of the purified enzyme
The antifungal activity of the purified enzyme was tested by the disc diffusion method. The tested fungi were precultured on PDA medium and adjusted at 105 cfu·mL−1, then added to PDA plates before solidification. Filter-paper discs (7 mm in diameter) impregnated with the purified enzyme solution were placed on plates inoculated with the test organism. Three replicates were used. The activity was determined after 72 h of incubation at 28 °C. The diameters (mm) of the inhibition zones were measured.
Results and discussion
Assay of antifungal activity
In the present study, S. griseorubens E44G inhibited the growth of R. solani with an inhibition zone of 25 mm, indicating its antifungal potential (data not shown). This result is in agreement with that of Al-Askar et al. [Citation4], who assayed the antifungal activity of 40 actinomycete isolates against R. solani. The antifungal activity of streptomycetes is commonly attributed to the production of antifungal compounds [Citation22,Citation23] and/or extracellular hydrolytic enzymes.[Citation24,Citation25]
Screening for proteolytic activity
The result obtained from the proteolytic assay revealed that protease activity contributed to the aggressive nature of the tested isolate of S. griseorubens E44G. A clear zone was observed around the disc of the antagonist, which is indicative of casein hydrolysis (). Actinomycetes, particularly Streptomyces spp., are known to be good protease producers.[Citation26,Citation27] The protein substrate and the composition of the medium could considerably influence the extracellular protease production.[Citation28]
Purification of protease enzyme
An extracellular alkaline protease was partially purified from the culture filtrate of S. griseorubens E44G, using the ammonium sulphate precipitation method. Maximum precipitation of protease content was reached at saturation of 70% of ammonium sulphate, giving the highest proteolytic and specific activities compared with the crude protease and other concentrations (). Therefore, precipitation of protease with this percentage of saturation was adopted as standard precipitation. By using the ammonium sulphate method for protease precipitation, proteases from S. albidoflavus are precipitated by 45% saturation [Citation29] and proteases from S. alboniger, by 40% saturation.[Citation30]
Table 1. Purification of protease from S. griseorubens E44G.
After ammonium sulphate precipitation, the pellet was dialyzed and loaded onto a Sephadex G-100 column. The fractions were tested for protease activity and the active fractions (nos. 10 and 11) were pooled (). The elution profile of the enzyme solution is shown in . Reports on the purification of proteases by different affinity chromatographic methods show that Sephadex is commonly the method of choice for separation of proteases.[Citation31–33] For example, Hatanaka et al. [Citation34] reported that Sephadex G-75 was used for the purification of proteases from S. albidoflavus.
Figure 2. Elution profile of alkaline protease of S. griseorubens E44G via Sephadex G-100 column chromatography.
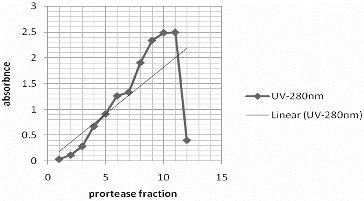
SDS-PAGE analysis showed four bands with a relative molecular mass of approximately 40, 35, 25 and 18 kDa (data not shown), while the purified protease showed a single band. This result suggested that several proteases were present in the culture filtrate of S. griseorubens E44G. The pooled fraction was re-purified again using column chromatography (Sephadex G-100) and one fraction was positive for protease activity. The purified enzyme showed a single band in SDS-PAGE, indicating that the preparation was homogeneous (). The molecular mass of the purified protease was 35 kDa. On the basis of these data, protease recovery and specific activity were calculated. gives a summary of these data from a representative run.
pH stability
The effect of pH on the protease activity was determined in the pH range of 6–11. The enzyme activity increased gradually with increasing the initial pH and reached an optimum at pH 8.5 (). Further increase in pH led to a reduction in the enzyme activity. The effect of acidic pH (< 6) could not be determined, since casein precipitates at acidic conditions. This fact made it difficult to explore the enzyme activity over the whole pH range, and the presented data do not exclude the possibility that the enzyme could be most active at pH values lower than 6. Many Streptomyces spp. have been reported to produce high levels of alkaline protease in culture media with pH ranging between 7 and 8.5.[Citation33,Citation35]
Table 2. Effect of pH on protease enzyme activity.
Thermal stability
The results from the experiments on the thermal stability of the purified protease showed that considerable enzyme activity was observed in the range of 60–70 °C (). The optimum temperature for alkaline protease was found to be 60 °C and the activity decreased with further increase in the incubation temperature. The results in show that the purified protease was almost stable at 60 °C even after 30 min of incubation. Earlier, an extracellular protease from Actinomyces sp. was shown to have high optimal temperature ( ≥ 40 °C), thus indicating that it requires a heated environment for optimum activity.[Citation36] A slightly higher (50 °C) optimal temperature has also been reported for S. pseudogrisiolus NRC-15.[Citation37] The thermostability property of the enzyme purified in our study can be an advantage for use in industrial processes.
Table 3. Effect of temperature on the stability of the protease enzyme.
Antifungal activity of the purified enzyme
The results from the disc diffusion method showed a clear inhibition zone (3 mm), confirming the antifungal activity of the purified protease. The potential of the purified enzyme to inhibit R. solani growth in vitro indicates that this enzyme may play a role in the antagonistic interaction between S. griseorubens E44G and R. solani. However, other mechanisms may also contribute to the aggressive nature of S. griseorubens E44G, such as production of another inhibitory metabolite (an antibiotic/antifungal or a hydrolytic enzyme) or competition between the bacterium and the fungus for nutrients and space. Further studies on molecular characterization of the protease produced by S. griseorubens E44G, and its possible participation in the antagonistic process are underway.
Conclusions
In this study, the antifungal activity of S. griseorubens E44G against R. solani was investigated. A protease enzyme produced by S. griseorubens E44G demonstrated marked inhibitory effects against the pathogenic fungus. The purified protease was thermo-stable and retained full activity even after 1 hour of incubation at 60 °C. It showed optimum activity at pH 8.5 and 60 °C. These optimized conditions can be implemented for alkaline protease production on industrial scale.
Disclosure statement
No potential conflict of interest was reported by the authors.
Additional information
Funding
References
- Rashad YM, Abdel-Fattah GM, Hafez EE, El-Haddad SA. Diversity among some Egyptian isolates of Rhizoctonia solani based on anastomosis grouping, molecular identification and virulence on common bean. Afr J Microbiol Res. 2012;6(37):6661–6667.
- Ithurrart ME, Buttner G, Petersen J. Rhizoctonia root rot in sugar beet (Beta vulgaris ssp. altissima) – epidemiological aspects in relation to maize (Ze mays) as a host plant. J Plant Dis Protec. 2004;111:302–312.
- Arcury TA, Quandt SA. Pesticides at work and at home: exposure of migrant farmworkers. Lancet. 2003;362:2021.
- Al-Askar AA, Abdul Khair WM, Rashad, YM. In vitro antifungal activity of Streptomyces spororaveus RDS28 against some phytopathogenic fungi. Afr J Agric Res. 2011;6(12):2835–2842.
- Gnanamanickam SS. Biological control of crop diseases. New York, NY: Marcel Dekker Inc; 2002.
- Jayamurthy H, Valappil Sajna K, Dastagar SG, Pandey A. Anti-fungal potentials of extracellular metabolites of Western Ghats isolated Streptomyces sp. NII 1006 against moulds and yeasts. Indian J Exp Biol. 2014;52(11):1138–1146.
- Viterbo A, Inbar J, Hadar Y, Chet I. Plant disease biocontrol and induced resistance via fungal mycoparasites. In: Kubicek CP, Druzhinina IS, editors. Environmental and microbial relationships. Berlin: Springer Verlag; 2007. p. 325–340.
- Bhaskar N, Sudeepa ES, Rashmi HN, Selvi AT. Partial purification and characterization of protease of Bacillus proteolyticus CFR3001 isolated from fish processing waste and its antibacterial activities. Bioresour Technol. 2007;98:2758–2764.
- Jellouli K, Bougatef A, Manni L, Agrebi R, Siala R, Younes I, Nasri M. Molecular and biochemical characterization of an extracellular serine-protease from Vibrio metschnikovii. Microbiol Biotechnol. 2009;36:939–948.
- Deng A, Wu J, Zhang Y, Zhang G, Wen T. Purification and characterization of a surfactant-stable high-alkaline protease from Bacillus sp. B001. Bioresour Technol. 2010;101:7100–7116.
- Gupta R, Beg QK, Khan S, Chauhan B. An overview on fermentation, downstream processing and properties of microbial alkaline proteases. Appl Microbiol Biotechnol. 2002;60:381–395.
- Kocher GS, Mishra S. Immobilization of Bacillus circulans MTCC 7906 for enhanced production of alkaline protease under batch and packed bed fermentation conditions. Internet J Microbiol. 2009;7:359–378.
- Al-Askar AA, Abdulkhair WM, Rashad YM, Hafez EE, Ghoneem KM, Baka ZA. Streptomyces griseorubens E44G: a potent antagonist isolated from soil in Saudi Arabia. J Pure Appl Microbio. 2014;8(Spl. Edn. 2):221–230.
- Domsch KW, Gams W, Anderson TH. Compendium of soil fungi. London: Academic Press; 1980.
- Buchanan RE, Gibbons NE. Bergey's Manual of Determinative bacteriology. Baltimore: Williams and Wilkins; 1974.
- Larsen MD, Kristiansen KR, Hansen TK. Characterization of the proteolytic activity of starter cultures of Penicillium roqueforti for production of blue veined cheeses. Int J Food Microbiol. 1998;43(3):215–221.
- Tsuchida O, Yamagota Y, Ishizuka J, Arai J, Yamada J, Takeuchi M, Ichishima E. An alkaline protease of an alkalophilic Bacillus sp. Curr Microbiol. 1986;14:7–12.
- Lowry OH, Rosebrough NJ, Farr AL, Randall RJ. Protein measurement with Folin phenol reagent. J Biol Chem. 1951;193:265–275.
- Tremacoldi CR, Carmona EC. Production of extracellular alkaline proteases by Aspergillus clavatus. World J Microbiol Biotechnol. 2005;21(2):169–172.
- Bradford MM. A rapid and sensitive method for the quantitation of microgram quantities of protein utilizing the principle of protein-dye binding. Anal Biochem. 1976;72:248–254.
- Laemmli UK. Cleavage of structural proteins during the assembly of the head of bacteriophage T4. Nature. 1970;227:680–685.
- Fguira LF, Fotso S, Ameur-Mehdi RB, Mellouli L, Laatsch H. Purification and structure elucidation of antifungal and antibacterial activities of newly isolated Streptomyces sp. strain US80. Res Microbiol. 2005;156:341–347.
- Atta HM. An antifungal agent produced by Streptomyces olivaceiscleroticus, AZ-SH514. World Appl Sci J. 2009;6(11):1495–1505.
- Mukherjee G, Sen SK. Purification, characterization, and antifungal activity of chitinase from Streptomyces venezuelae P10. Curr Microbiol. 2006;53:265–269.
- Prapagdee B, Kuekulvong C, Mongkolsuk S. Antifungal potential of extracellular metabolites produced by Streptomyces hygroscopicus against phytopathogenic fungi. Int J Boil Sci. 2008;4(5):330–337.
- Simkhada JR, Mander P, Cho S, Yoo JC. A novel fibrinolytic protease from Streptomyces sp. CS684. Process Biochem. 2010;45:88–93.
- Manivasagan P, Venkatesan J, Sivakumar K, Kim S. Production, characterization and antioxidant potential of protease from Streptomyces sp. MAB18 using poultry wastes. BioMed Res Int. 2013;2013:496586. doi:10.1155/2013/496586
- Rifaat HM, El-Said OH, Hassanein SM, Selim SM. Protease activity of some mesophilic streptomycetes isolated from Egyptian habitats. J Cult Collec. 2007;5:16–24.
- Kang S, Kim I, Rho Y, Lee K. Production dynamics of extracellular proteases accompanying morphological differentiation of Streptomyces albidoflavus SMF301. Microbiology. 1995;141:3095–3103.
- Lopes A, Coelho R, Meirelles M, Branquinha M, Vermelho A. Extracellular serine-proteinases isolated from Streptomyces alboniger: partial characterisation and effect of aprotinin on cellular structure. Mem Inst Oswaldo Cruz. 1999;94:763–770.
- Rifaat HM, Hassanein SM, El-Said OH, Saleh SA, Selim MSM. Purification and characterization of extracellular neutral protease from Streptomyces microflavus. Arab J Biotech. 2006;9:51–60.
- Prasad MP, Rekha S, Arthirajan J. Optimization studies of Protease enzyme in in-vitro conditions from Bacillus licheniformis. Int Res J Biological Sci. 2014;3(2):34–39.
- Abdelwahed NAM, Danial EN, El-Naggar NE, Mohamed AA. Optimization of alkaline protease production by Streptomyces ambofaciens in free and immobilized form. Am J Biochem Biotechnol. 2014;10(1):1–13.
- Hatanaka T, Yoshiko Uesugi JA, Iwabuchi M. Purification, characterisation cloning, and sequencing ofmetalloendopeptidase from Streptomycesseptatus TH-2. Arch Biochem Biophys. 2005;434:289–298.
- Azeredo LA, Freire DM, Soares RM, Leite SG, Coelho RR. Production and partial characterisation of thermophilic proteases from Streptomyces sp. isolated from Brazilian cerrado soil. Enz Microbiol Technol. 2004;34:354–358.
- Aftab S, Ahmed S, Saeed S, Rasool SA. Screening, isolation and characterization of alkaline protease producing bacteria from soil. Pak J Biol Sci. 2006;9(11):2122–2126.
- Awad HM, Mostafa EE, Saad MM, Selim MH, Hassan HM. Partial purification and characterization of extracellular protease from a halophilic and thermotolerant strain Streptomyces pseudogrisiolus NRC-15. Indian J Biochem Biophys. 2013;50(4):305–311.