Abstract
An efficient system for in vitro propagation of the endangered medicinal plant Rauvolfia serpentina has been developed. Proliferation of shoots was achieved from nodal segment explants, excised from a field grown plants on Murashige and Skoog (MS) medium supplemented with thidiazuron (TDZ) (0.1–2.5 μmol/L) although with low regeneration response and few number of shoots per explant. Greater number of shoots was achieved from nodal explants pretreated with higher concentrations of TDZ (5–100 μmol/L) in liquid MS medium for different time periods (4, 8, 12 and 16 days), followed by their transfer on a growth regulator-free medium. The highest response in terms of percent regeneration (90%), average number of shoots/explant (23.17 ± 2.15) and maximum shoot length (5.3 cm ± 0.83 cm) were achieved by pretreating the nodal explants with 50 μmol/L TDZ for 8 days. Rooting was achieved in MS medium supplemented with 0.5 μmol/L indole-3-butyric acid with an average of 5.50 ± 0.92 roots per microshoot. The rooted plantlets were transferred to soil, where 90% of them grew well and exhibited a normal development. Chlorophyll a and b, carotenoids, net photosynthetic rate and antioxidative enzymes, viz., superoxide dismutase and catalase activities were measured in leaf tissues during ex vitro acclimatization at 0, 7, 14, 21 and 28 days. Most of the activities showed a decreasing trend but subsequently increased after 7 days of acclimatization. These changes suggested the adjustment of the in vitro propagated plantlets to ex vitro conditions.
Introduction
Biotechnology has offered an immense potential for plant improvement and has become increasingly important worldwide. It offers new technologies for sustainable development and utilization of natural resources since many prized medicinal plants are facing the threat of extinction due to overexploitation and unsustainable utilization. The application of biotechnological approaches to medicinal plants would also benefit from the development of cell, tissue and organ culture systems for in vitro growth and regeneration of medicinal plants.[Citation1,Citation2] Tissue culture technologies could be a cost-effective means of high volume production of the elite planting material throughout the year without any seasonal constraints. This technology may also play an imperative role in preservation and micropropagation of germplasm that is rare, endangered or on the edge of extinction. In vitro propagation offers a viable tool for mass breeding and germplasm preservation of many medicinal and aromatic plants.[Citation3–10]
Thidiazuron (TDZ) (N-phenyl-N′-1,2,3-thidiazol-5-yl-urea), a substituted phenyl urea derivative, has a non-purine structure but has a strong cytokinin-like activity. It induces diverse morphogenic responses, ranging from tissue proliferation to induction of shoot buds and somatic embryos.[Citation11–13] Apart from its cytokinin-like activity, TDZ has been suggested to modulate the endogenous auxin levels.[Citation14] However, it remains to be resolved whether it has an auxin activity or if it is involved in the auxin metabolism.[Citation15]
Tissue culture plantlets grow heterotrophically in vitro, because of the low light intensity and the provided carbohydrates in the medium. When the transplants are removed from the culture vessels, they must acclimatize to higher light intensity, fluctuating temperatures, lower relative humidity and availability of some mineral nutrients. These conditions may result in the formation of plantlets with abnormal morphology, anatomy and physiology, which may lead to a higher percentage of plantlets' death because of the sudden changes after the ex vitro transfer.[Citation16–20] The physiological status of the in vitro grown plantlets during acclimatization is an important factor, which determines the success rates.[Citation21–23] After the ex vitro transplantation, plantlets usually need several weeks for acclimatization under shade with gradually lowering relative humidity [Citation24,Citation25] to avoid desiccation and photoinhibition and to repair the abnormalities.[Citation26,Citation27] Acclimatization of tissue culture plants to the ex vitro environment is a critical step for many species, requiring time and expensive systems that hamper the commercial application of the in vitro technique.[Citation28] Sudden changes in the environmental conditions during the acclimatization of tissue culture raised plants may generate stress through the formation of reactive oxygen species (ROS).[Citation29–31] In order to combat the danger posed by the generation of ROS, plant cells have evolved both enzymatic and non-enzymatic mechanisms.[Citation32] Superoxide dismutase (SOD) and catalase (CAT) are efficient antioxidant enzymes and are a part of the enzymatic mechanisms.[Citation33] Their combined action prevents cellular damage caused by O2−, H2O2 and OH−.
Rauvolfia serpentina (L.) Benth. ex Kurz., (Apocynaceae) is an endangered medicinal plant recognized worldwide. Due to the presence of indole alkaloids and its usage as an antihypertensive drug, the demand for this plant has increased manifold in the global pharmaceutical industry. There have been few previous reports on micropropagation of R. serpentina via callus, shoot tips and nodal segments,[Citation34–40] but none of these reports have used TDZ for in vitro propagation. The aim of the present study was to assess the effect of TDZ on the multiple shoot induction by culturing nodal segments of R. serpentina in liquid medium followed by their transfer to medium without TDZ and to optimize the physiological and biochemical activities during acclimatization of micropropagated plants.
Materials and methods
Explants source and surface sterilization
Nodal segments of healthy plants were excised and used as explants. The explants were thoroughly washed under running tap water for half an hour, followed by soaking with 5% (v/v) Labolene detergent solution for 5 min. After several washes with sterile distilled water, the explants were surface sterilized with 0.1% (w/v) mercuric chloride solution for 5 min. After a thorough washing with sterile distilled water, the explants were cut into 0.5–1.0 cm sized pieces and cultured on sterile Murashige and Skoog (MS) medium.[Citation41]
Growth medium and growth conditions
MS growth medium with 3% (w/v) sucrose and 0.8% (w/v) agar was used throughout the experiment. Plant growth regulators and their combinations were added to the medium, as specified below. The pH of the medium was adjusted to 5.8 using 1 mol/L NaOH or 1 mol/L HCl prior to autoclaving at 1.1 kg/cm2 pressure at 121 °C for 20 min. Cultures were maintained at 24°C ± 2 °C with a 16-h photoperiod under a photon flux density of 50 µmol m−2 s−1 provided by cool white fluorescent tubes.
Multiple shoot induction and proliferation
In the present study two sets of experiments were carried out on multiple shoot induction and proliferation from nodal explants of R. serpentina. In the primary experiment, the nodal explants were employed onto MS medium augmented with different concentrations of TDZ (0.1, 0.3, 0.5, 0.8, 1.0 or 2.5 µmol/L). Data for shoot induction and multiplication were recorded after 4 weeks of culturing. MS medium, lacking TDZ, served as a control. After 4 weeks the shoots, induced from TDZ containing media, were transferred into growth medium devoid of TDZ. The shoots were subcultured every 3 weeks onto a fresh growth medium. The regeneration ability was tested up to the fifth subculture and the maximum number of shoots and shoot length were recorded at each passage of subculturing.
The second experiment was planned to see the effect of TDZ supplemented liquid MS medium on shoot multiplication from nodal explants. The explants were cultured into liquid MS medium supplemented with TDZ at different concentrations (5, 25, 50, 75 and 100 µmol/L) on a rotary shaker at 100 rpm for 4, 8, 12 and 16 days. After a distinct period, the explants were transferred onto MS basal medium for further growth and proliferation. All cultures were transferred onto fresh medium every 3 weeks. The percentage of explants forming shoot, shoot numbers and shoot length were recorded after 8 weeks of transfer.
Rooting and acclimatization
Elongated healthy shoots measuring from about 4 to 5 cm were excised and transferred onto rooting medium. The rooting medium comprised MS medium augmented with indole-3-butyric acid (IBA) at different concentrations (0.1, 0.5, 1.0, 1.5 or 2.0 µmol/L). Data on percentage of rooting and root numbers and length were recorded after 4 weeks of culturing. Plantlets with well-developed shoots and roots were transferred to plastic pots containing sterile soilrite after thorough washing in running tap water. The transferred plants were maintained under diffuse light conditions (16/8 h photoperiod) and covered with transparent plastic bags to ensure high humidity. The plants were watered every 3 days with half-strength MS salt solution for 2 weeks. After 4 weeks, the acclimatized plants were transferred to pots containing normal garden soil and maintained in greenhouse under normal day length conditions. Leaf samples were collected at different time points, including 0, 7, 14, 21 and 28 days during acclimatization and frozen in liquid nitrogen until needed for physiological and biochemical studies.
Leaf gas exchange measurement
Net photosynthetic rate (PN) was measured on fully expanded leaves using portable infrared gas analyzer (IRGA, LI-COR 6400, Lincoln, USA) on the basis of net exchange of CO2 between leaf and atmosphere by enclosing the leaf in the leaf chamber and monitoring the rate at which the CO2 concentration changed over short time intervals. The net photosynthetic rate was expressed as µmol CO2 m−2 s−1.
Chlorophyll and carotenoid measurement
The Chlorophyll (Chl) a and b and the carotenoid contents were estimated by the method of Mckinney [Citation42] and McLachlan and Zalik,[Citation43] respectively. About 500 mg of fresh leaf tissue was ground in 5 mL acetone (80%), and the suspension was filtered with Whatman filter paper no 1. The absorbance of the filtered solution was then measured at 645 and 663 nm for chlorophyll a and b, respectively, and at 480 and 663 nm for carotenoid content, using a UV-vis spectrophotometer (UV 1700 PharmaSpec, Shimadzu, Tokyo, Japan). Chlorophyll and carotenoid contents were expressed in mg g−1 fresh weight (FW).
Superoxide dismutase activity measurement
The SOD activity was measured by the method of Dhinsa et al.[Citation44] SOD activity in the supernatant was assayed by its ability to inhibit the photochemical reduction. The assay mixture comprised 1.5 mL reaction buffer, 0.2 mL methionine, 0.1 mL enzyme extract with equal amount of 1 mol/L Na2CO3, 2.25 mmol/L NBT solution, 3 mmol/L ethylenediaminetetraacetic acid (EDTA), 60 µmol/L riboflavin and 1.0 mL double distilled water and was taken in test tubes, which were incubated under the light of 15 W fluorescent lamp for 10 min at 25/28°C. Blank A, containing all the above substances of the reaction mixture, along with the enzyme extract, was placed in the dark. Blank B, containing all the above substances of the reaction mixture, except the enzyme extract, was placed in light, along with the sample. The reaction was stopped by switching off the light, and the tubes were covered with a black cloth. The non-irradiated reaction mixture, containing the enzyme extract, did not develop a light blue colour. The absorbance of the samples, along with blank B, was read at 560 nm against the blank A. The difference of colour reduction between blank B and sample was then calculated. We considered 50% reduction in colour as one unit of enzyme activity and the activity was expressed in enzyme units (EU) mg−1 protein.
Catalase activity measurement
CAT activity in the leaf tissues was determined by the method of Aebi [Citation45] with minor modifications. The reaction was carried out in a 2.0 mL volume of reaction mixture comprising of reaction buffer with 0.1 mL 3 mmol/L EDTA, 0.1 mL enzyme extract and 0.1 mL 3 mmol/L H2O2. The reaction was allowed to run for 5 min. The catalase activity was determined by monitoring the disappearance of H2O2 by measuring a decrease in the absorbance at 240 nm using spectrophotometer (UV-Pharma Spec 1700, Shimadzu, Japan). The activity was calculated by using extinction coefficient (∑) 0.036 mmol/L cm−1 and expressed in EU mg−1 protein. One unit of enzyme determines the amount necessary to decompose 1 µmoL of H2O2 per min at 25 °C.
Statistical analysis
All the experiments were repeated thrice with a minimum of 10 replicates per treatment. The data was analysed statistically using SPSS Ver. 14 (SPSS Inc., Chicago, USA) and the significance of differences among the means were carried out using Duncan's multiple range test at P ≤ 0.05 probability level. The results were expressed as means ± SE of the three repeated experiments.
Results and discussion
Multiplication of plants from enhanced axillary buds or shoots is the most appropriate and consistent method of true-to-type in vitro propagation.[Citation46,Citation47] In vitro proliferation of shoots is usually promoted by incorporating growth regulators into the culture medium.[Citation48] TDZ is being selected for in vitro propagation of many plant species because of its tremendous ability to stimulate shoot proliferation.[Citation49–51] Compared to most of the other active compounds added to the media, extremely low concentrations of TDZ stimulate axillary shoot proliferation of many plant species. The morphogenetic response of R. serpentina nodal segment explants to different concentrations of TDZ (0.1, 0.3, 0.5, 0.8, 1.0 and 2.5 µmol/L) is summarized in . All concentrations of TDZ facilitated shoot bud induction and their subsequent proliferation. Of the various tested levels of TDZ, 0.8 µmol/L proved to be the most effective for inducing a maximum percentage of regeneration (77%), numbers of shoots (5.7 ± 0.28) and shoot length (3.4 cm ± 0.43 cm) after 4 weeks of culturing. On increasing the concentration of TDZ from 0.8–2.5 µmol/L, the number of shoots per explant was reduced. Similarly, at lower concentration (0.1, 0.3 and 0.5 µmol/L), the percentages of regeneration as well as the number of shoots were drastically reduced. The effectiveness of TDZ in shoot bud differentiation has been documented in wide number of plants.[Citation8,Citation10,Citation52–56]
Table 1. Effect of TDZ on shoot regeneration from nodal segment explants of R. serpentina in MS medium after 4 weeks of culturing.
After an induction period of 4 weeks, the shoot multiplication rate was increased when each responsive explant was transferred to a TDZ free MS basal medium. However, fasciated or distorted shoots were observed in cultures, continuously grown on TDZ augmented media.[Citation12,Citation57,Citation58] Subculturing had a significant effect on shoot proliferation. The numbers of shoots and shoot length increased after each subculture and showed no sign of decline until the fifth subculture passage (). The highest shoot multiplication (9.6 ± 0.84) and shoot length (5.5 ± 0.35 cm) were recorded at the fifth subculture passage. Similar observation was also documented in Aegle marmelos.[Citation59] This is probably due to the capacity of TDZ in stimulating endogenous cytokinin biosynthesis or in altering cytokinin metabolism.[Citation60–64]
Figure 1. Evaluation of morphogenetic potential of shoot culture from nodal explants of R. serpentina obtained from TDZ (0.8 µmol/L) after being tested for five subculture passages on growth regulator free MS medium.
Note: Bars represent the means ± standard error (SE). Bars denoted by the same letter within response variables are not significantly different (P ≤ 0.05) using Duncan's multiple range test.
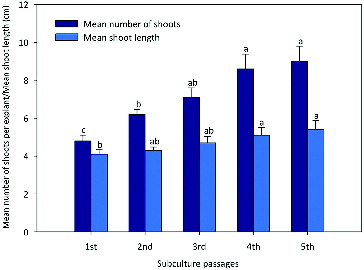
Effect of TDZ dosage and duration in liquid MS medium
This experiment was conducted in order to see the effect of nodal explants to TDZ supplemented liquid MS medium. The explants were cultured in liquid MS medium supplemented with TDZ at different concentrations (5, 25, 50, 75 and 100 µmol/L) on a rotary shaker at 100 rpm for 4, 8, 12 and 16 days (). After a defined duration, the explants were cultured on MS basal medium for further growth and proliferation. The nodal segment failed to produce shoots on MS medium without TDZ. The explants cultured on the media containing different concentrations of TDZ for different duration of incubation showed initial bud break after one week. The range of percentage of shoot regenerating explants was from 56% to 90% and the number of shoots formed per explant varied considerably and showed significant difference at different concentrations and duration of exposure to liquid MS medium, followed by their transfer to MS semi-solid medium. Among the various tested concentrations and culturing durations, the highest shoot regeneration frequency (90%), mean number of shoots (23.1 ± 2.15) and maximum shoot length (5.3 ± 0.83 cm) were recorded after 8 weeks at 50 µmol/L TDZ for 8 days culture duration (A)). The effectiveness of short term exposure and stimulating effect of TDZ on bud break and multiple shoot formation has been reported earlier for several medicinal and aromatic plant species including Ocimum basilicum,[Citation10] Arachis correntina,[Citation65] Curcuma longa,[Citation66] Curculigo orchioides [Citation67] and Mentha arvensis.[Citation68] It is suggested that TDZ may be needed as a trigger for initiating the proliferation of shoot meristems. Incubation on TDZ free MS medium led to further development of the explants.[Citation69] The explants, which were cultured on TDZ supplemented liquid MS medium for 12 and 16 days, showed reduction in all of the evaluated parameters and the shoots appeared stunted and deformed.
Table 2. Effect of different concentrations and duration of culture on TDZ supplemented liquid MS medium followed by their transfer to MS basal semi-solid medium on shoot formation from nodal explants of R. serpentina after 8 weeks of culturing.
Rooting and acclimatization
In vitro regenerated shoots measuring from about 4 to 5 cm in length were transferred to MS medium supplemented with IBA at different concentrations (0.1, 0.5, 1.0, 1.5 and 2.0 µmol/L) for rooting (). The rooting frequency varied from 60% to 87%, depending upon the auxin used. IBA with 0.1 µmol/L concentration induced 2.9 ± 0.34 roots in 60% of the shoots. On increasing its concentration, the rooting frequency was also increased. The maximum frequency of root formation (87%), number of roots (6.9 ± 0.34) and root length (5.2 cm ± 0.40 cm) was attained in a medium containing 1.0 µmol/L IBA after 4 weeks of transfer. The effectiveness of IBA in root induction has been reported in Tylophora indica,[Citation4] M. arvensis,[Citation69] Sansevieria cylindrical [Citation70] and Cyamopsis tetragonoloba.[Citation71] Rooted plants with 6–8 fully expanded leaves and well-developed roots (B)) were transferred into pots containing sterile soilrite and covered with transparent polybags to maintain high humidity. After one month the plants were transferred to normal garden soil and maintained in field conditions with 90% survival rate (C)).
Table 3. Effect of different IBA concentrations on root induction from in vitro raised microshoots of R. serpentina in MS medium after 4 weeks of culturing.
Physiological and biochemical studies
Photosynthetic parameters, viz., Chl a, Chl b, carotenoid and net photosynthetic rate (PN) were assessed in regenerated plantlets of R. serpentina at 0 (control), 7, 14, 21 and 28 days of acclimatization (). Contents of Chl a and Chl b showed an increasing trend over the control plantlets. Chl a increased from 0.18 ± 0.031 to 0.29 ± 0.026 mg g−1 FW, whereas Chl b was increased from 0.08 ± 0.017 to 0.13 ± 0.019 mg g−1 FW after 28 days of acclimatization. The carotenoid content significantly dropped from 0 to 14 days and thereafter showed an improvement from 0.12 ± 0.011 to 0.14 ± 0.015 mg g−1 FW after 28 days of acclimatization. Improvement in the chlorophyll content after ex vitro transfer was also observed in Oil palm [Citation72] and tobacco [Citation73] plants. The carotenoid plays an important role in the protection of the chlorophyll pigments under stress conditions that might be generated during acclimatization.[Citation74] The carotenoid content was increased during subsequent acclimatization periods. Net photosynthetic rate (PN) decreased to 1.5 ± 0.27 μmol CO2 m−2 s−1 after seven days of transplantation against 1.8 ± 0.23 μmol CO2 m−2 s−1 in 0 day plantlets. Later on, PN was significantly increased in the newly formed leaves and the highest photosynthetic rate of 3.2 ± 0.25 μmol CO2 m−2 s−1 was observed after 28 days of acclimatization. Photosynthetically active in vitro leaves have also been observed in Spathiphyllum floribundum plantlets.[Citation75] After one week, PN showed increasing trend and was found to be associated with the formation of new leaves.[Citation76,Citation77]
Figure 3. Changes in photosynthetic pigments and net photosynthetic rate (PN) of micropropagated plantlets of R. serpentina during different days of acclimatization.
Note: Fresh weight (FW). Points represent means ± standard error (SE). Points denoted by the same letter within response variables are not significantly different (P ≤ 0.05) using Duncan's multiple range test.
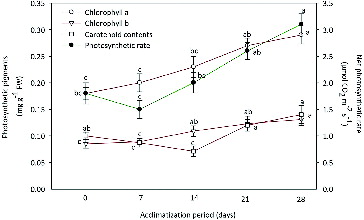
Changes in enzymatic activity
A differential pattern of SOD and CAT activity was observed in regenerated plantlets at 0 (control), 7, 14, 21 and 28 days of acclimatization (). SOD activity was increased significantly (23.0 ± 1.53 Unit mg−1 protein) at day 7, when compared to the control plantlets (16.2 ± 1.10 Unit mg−1 protein) and thereafter showed a decreasing trend. The SOD activity after 28 days of transplantation was recorded 17.6 ± 0.87 Unit mg−1 protein. CAT activity showed an increasing trend during the whole experiment, compared to the control plants. The quantitative analysis revealed 2.5, 3.9, 4.1, 4.8 and 5.1 μmol min−1 mg−1 protein at 0, 7, 14, 21 and 28 days of acclimatization, respectively. The increased activity of SOD during the acclimatization resembles with the earlier reports of Chai et al. [Citation31] and Van Huylenbroeck et al.[Citation75] The catalase activity increased during the acclimatization period. This observation suggested an up-regulation of the plant protective mechanisms against oxidative stress through scavenging H2O2 by converting it into O2 and H2O in peroxisomes.[Citation78,Citation79] These results corroborate the earlier reports in Rauvolfia tetraphylla, [Citation27], Tecomella undulate [Citation80] and Cassia alata.[Citation81]
Figure 4. Changes in superoxide dismutase and catalase activity of micropropagated plantlets of R. serpentina during different days of acclimatization.
Note: Points represent means ± standard error (SE). Points denoted by the same letter within response variables are not significantly different (P ≤ 0.05) using Duncan's multiple range test.
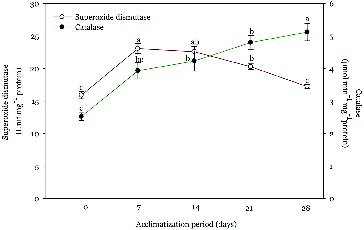
Conclusion
The present study described an efficient protocol for in vitro axillary shoot multiplication using thidiazuron, which revealed a definite advantage over the earlier reports for regeneration of R. serpentina – a potent antihypertensive drug producing plant. Various physiological and biochemical parameters were evaluated for the first time during an ex vitro acclimatization of plantlets. The developed protocol could be helpful to explore the possibility of ex vitro conservation and improvement of active constituents by genetic engineering of this endangered medicinal plant.
Acknowledgements
The author is thankful to the King Saud University, Deanship of Scientific Research, College of Science Research Centre for financial assistance.
Disclosure statement
No potential conflict of interest was reported by the author.
Additional information
Funding
References
- Briskin DP. Medicinal Plants and Phytomedicines. Linking plant biochemistry and physiology to human health. Plant Physiol. 2000;124(2):507–514.
- Esmaeili AK. Micropropagation, antioxidant and antimicrobial activities of Asparagus officinalis Cv. Mary washington in vivo and in vitro [dissertation]. Kuala Lumpur: University of Malaya; 2012.
- Sudha CG, Seeni S. In vitro multiplication and field establishment of Adhotoda beddomei GB, Clarke, a rare medicinal plant. Plant Cell Rep. 1994;13:203–207.
- Pattnaik S, Chand PK. In vitro propagation of the medicinal herbs Ocimum americanum L. Syn. O. canum Sims. (Hoary bsil) and Ocimum sanctum L. (Holy basil). Plant Cell Rep. 1996;15:846–850.
- Amo-Marco JB, Ibanez MR. Micropropagation of Limonium cavanillesii Erben, a threatened statice, from inflorescence stems. Plant Growth Regul. 1998;24:49–54.
- Faisal M, Anis M. Rapid mass propagation of Tylophora indica Merill via leaf callus culture. Plant Cell Tissue Organ Cult. 2003;75:125–129.
- Anis M, Faisal M. In vitro regeneration and mass propagation of Psoralea corylifolia – an endangered medicinal plant. Indian J Biotechnol. 2005;4:261–264.
- Faisal M, Siddique I, Anis M. An efficient plant regeneration system for Mucuna pruriens L. (DC.) using cotyledonary node explants. In Vitro Cell Dev Biol – Plant. 2006;42:59–64.
- Siddique I, Anis M. In vitro shoot multiplication and plantlet regeneration from nodal explants of Cassia angustifolia (Vahl.): a medicinal plant. Acta Physiol Plant. 2007;29:233–238.
- Bacila I, Coste A, Halmagyi A, Deliu C. Micropropagation of Hypericum maculatum Cranz an important medicinal plant. Rom Biotechnol Lett. 2010;15:86–99.
- Huetteman CA, Preece JE. Thidiazuron: a potent cytokinin for woody plant tissue culture. Plant Cell Tissue Organ Cult. 1993;33:105–119.
- Faisal M, Ahmad N, Anis M. Shoot multiplication in Rauvolfia tetraphylla L. using thidiazuron. Plant Cell Tissue Organ Cult. 2005;80:187–190.
- Siddique I, Anis M. Rapid micropropagation of Ocimum basilicum using shoot tip explants pre-cultured in thidiazuron supplemented liquid medium. Biol Plant. 2007;51:787–790.
- Petrić M, Subotić A, Jevremović S, Trifunović M. Somatic embryogenesis and bulblet regeneration in snakehead fritillary (Fritillaria meleagris L.). Afr J Biotechnol. 2011;10(72):16181–16188.
- Yi C. The use of thidiazuron in tissue culture. In Vitro Cell Dev Biol – Plant. 1993;29:92–96.
- Kozai T, Fujiwara K, Hayashi M, Aitken-Christie J. The in vitro environment and its control in micropropagation. In: Kurata K, Kozai T, editors. Transplant production systems. London: Kluwer Academic Publishers; 1992. p. 247–282.
- Buddendorf-Joosten JMC, Woltering EJ. Components of the gaseous environment and their effects on plant growth and development in vitro. Plant Growth Regul. 1994;15:1–16.
- Desjardins Y. Photosynthesis in vitro-on the factors regulating CO2 assimilation in micropropagation systems. Acta Hort. 1995;393:45–61.
- Pospisilova J, Haisel D, Synkova H, Catsky J, Wilhelmova N, Plzakova S, Prochazkova D, Sramek F. Photosynthetic pigments and gas exchange during ex vitro acclimation of tobacco plants as affected by CO2 supply and abscisic acid. Plant Cell Tissue Organ Cult. 2000;61:125–133.
- Faisal M, Anis M. Effect of light irradiations on photosynthetic machinery and antioxidative enzymes during ex vitro acclimatization of Tylophora indica plantlets. J Plant Interaction. 2009;5:21–27.
- Debergh PC. Acclimatization techniques of plants from in vitro. Acta Hort. 1991;289:291–300.
- Van Huylenbroeck JM, Debergh PC. Impact of sugar concentration in vitro on photosynthesis and carbon metabolism during ex vitro acclimatization of Spathiphyllum plantlets. Physiol Plant. 1996;96:298–304.
- Rival A, Triques K, Beule T, Aberlenc-Bertossi F, Morcillo F, Huet C, Grosdemange F, Hocher V, Verdeil JL, Duval Y, Hamon S. The zygotic embryo: a model for physiological studies in coconut. Curr Plant Sci Biotechnol Agri. 1999;35:355–369.
- Preece JE, Sutter EG. Acclimatization of micropropagated plants to the greenhouse and field. In: Debergh PC, Zimmerman RH, editors. Micropropagation: technology and application. London: Kluwer Academic Publishers; 1991.p. 71–93.
- Kadlecek P. Effect of pretreatment by irradiance and exogenous saccharose under in vitro conditions on photosynthesis and growth of tobacco (Nicotiana tabacum L.) plants during acclimatization after transfer to soil. [dissertation]. Praha: Department of Plant Physiology, Charles University; 1997.
- Bag N, Chandra S, Palni LMS, Nandi SK. Micropropagation of Dev-ringal [Thamnocalamus spathiflorus (Trin.) Munro] – a temperate bamboo, and comparison between in vitro propagated plants and seedlings. Plant Sci. 2000;156:125–135.
- Faisal M, Anis M. Changes in photosynthetic activity, pigment composition, electrolyte leakage, lipid peroxidation, and antioxidant enzymes during ex vitro establishment of micropropagated Rauvolfia tetraphylla plantlets. Plant Cell Tissue Organ Cult. 2009;99:125–132.
- Ahmad N, Faisal M, Fatima N, Anis M. Encapsulation of microcuttings for propagation and short-term preservation in Ruta graveolens L.: a plant with high medicinal value. Acta Physiol Plant. 2012;34:2303–2310.
- Santamaria JM, Davies WJ, Atkinson CJ. Stomata from micropropagated Delphinium plants responded to ABA, CO2, water potential and light but fail to close fully. J Exp Bot. 1993;44:99–107.
- Van Huylenbroeck JM, Piqueras A, Debergh PC. The evolution of photosynthetic capacity and the antioxidant enzymatic system during acclimatization of micropropagated Calathea plant. Plant Sci. 2000;155:59–66.
- Chai TT, Fadzillah NM, Kusnan M, Mahmood M. Water stress-induced oxidative damage and antioxidant responses in micropropagated banana plantlets. Biol. Plant. 2005;49:153–156.
- Inze D, Van Montagu M. Oxidative stress in plants. Curr. Opin Biotechnol. 1995;6:153–158.
- Scandalios JG. Oxygen stress and superoxide dismutase. Plant Physiol. 1993;101:7–12.
- Stockgit J, Pfitzner A, Firl J. Indole alkaloids from cell suspension cultures of Ruawolfia serpentina Benth. Plant Cell Rep. 1981;1:36–39.
- Yamamoto O, Yamada Y. Production of reserpine and its optimization in culture Ruawolfia serpentina Benth. Plant Cell Rep. 1986;5:50–53.
- Benjamin BD, Roja G, Heble MR. Agrobacterium rhizogens mediated transformation of Rauvolfia serpentina: regeneration and alkaloid synthesis. Plant Cell Tissue Organ Cult. 1993;35:253–257.
- Roja G, Heble MR. Indole alkaloids in clonal propagules of Rauwolfia serpentina benth ex kurz. Plant Cell Tissue Organ Cult. 1996;44:111–115.
- Ilahi I, Rahim F, Jabeen M. Enhaced clonal propagation and alkaloid biosynthesis in culture of Rauwolfia. Pak J Plant Sci. 2007;13:45–56.
- Bhatt R, Arif M, Gaur AK, Rao PB. Rauwolfia serpentina: protocol optimization for in vitro propagation. Afr J Biotechnol. 2008;7:4265–4268.
- Faisal M, Alatar AA, Hegazy AK. High frequency shoot regeneration and plant establishment of Rauvolfia serpentina: an endangered medicinal plant. J Med Plant Res. 2012;6:3324–3329.
- Murashige T, Skoog F. A revised medium for rapid growth and bioassays with tobacco tissue cultures. Physiol Plant. 1962;15:473–497.
- Mckinney G. Absorption of light by chlorophyll solution. J Biol Chem. 1940;140:315–322.
- McLachlan S, Zalik S. Plastid structure, chlorophyll concentration and free amino acid composition of chlorophyll mutant by barley. Canadian J Bot. 1963;1053–1062.
- Dhinsa RS, Plumb-Dhinsa P, Thorpe TA. Leaf senescence: correlated with increased levels of membrane permeability and lipid peroxidation and decreased levels of superoxide dismutase and catalase. J Exp Bot. 1981;32:93–101.
- Aebi H. Catalase in vitro. Methods Enzymol. 1984;105:121–126.
- George EF, Hall MA, De Klerk GJ. Plant propagation by tissue culture. The background. 3rd ed. Vol. 1. Dordrecht: Springer; 2008.
- Varshney A, Anis M. Trees: propagation and conservation: biotechnological approaches for propagation of a multipurpose tree, Balanites aegyptiaca. New Delhi: Springer; 2014.
- Malda G, Backhaus RA, Martin C. Alterations in growth and crassulacean acid metabolism (CAM) activity of in vitro cultured cactus. Plant Cell Tissue Organ Cult. 1999;58(1):1–9.
- Sharma V, Gupta SK, Dhiman M. Regeneration of plants from nodal and internodal segment cultures of Ephedra gerardiana using thidiazuron. Plant Tissue Cult Biotech. 2012;22(2):153–161.
- Ružić DV, Vujović TI. The effects of cytokinin types and their concentration on in vitro multiplication of sweet cherry cv. Lapins (Prunus avium L.). Hort Sci (Prague). 2008;35(1):12–21.
- Mitrofanova IV, Mitrofanova OV. Development of recipient system of woody subtropical plants in vitro. Acta Universitatis Latviensis, Biol. 2004;676:189–676.
- Malik KA, Saxena PK. Regeneration in Phaseolus vulgaris L. high frequency induction of direct shoot formation in intact seedlings by N6- benzylamino purine and thidiazuron. Planta. 1992;186:384–389.
- Faisal M, Anis M. Thidiazuron induced high frequency axillary shoot multiplication in Psoralea corylifolia. Biol Plant. 2006;50:437–440.
- Kanyand M, Peterson CM, Prakash CS. The differentiation of emergences into adventitious shoots in peanut, Arachis hypogaea (L.). Plant Sci. 1997;126:87–95.
- Tiwari V, Tiwari KN, Singh BD. Comparative studies of cytokinins on in vitro propagation of Bacopa monniera. Plant Cell Tissue Organ Cult. 2001;66:9–16.
- Siddique I, Anis M, Jahan AA. Rapid Multiplication of Nyctanthes arbor-tristis L. Through In vitro Axillary Shoot Proliferation. World J Agric. Sci. 2001;2(2):188–192.
- Subotić A, Jevremović S, Grubišić D. Influence of cytokinins on in vitro morphogenesis in root cultures of Centaurium erythraea – valuable medicinal plant. Sci Hortic. 2009;120 386–390
- Mukhtar S, Ahmad N, Khan MI, Anis M, Aref IM. Influencing micropropagation in Clitoria ternatea L. through the manipulation of TDZ levels and use of different explant types. Physiol Mol Biol Plant. 2012;18(4):381–386.
- Ajithkumar D, Seeni S. Rapid clonal multiplication through in vitro axillary shoot proliferation of Aegle marmelos (L.) Corr., a medicinal tree. Plant Cell Rep. 1998;17:422–426.
- Li H, Murch SJ, Saxena PK. Thidiazuron induced de novo shoot organogenesis on seedlings, etiolated hypocotyls and stem segments of Huang-qin. Plant Cell Tissue Organ Cult. 2000;62:169–173.
- Ahmad N, Siddique I, Anis M. Improved plant regeneration in Capsicum annuum L. from nodal segments. Biol Plant. 2006;50:701–704.
- Guo B, Abbasi BH, Zeb A, Xu LL, Wei YH. Thidiazuron: A multi-dimensional plant growth regulator. Afr J Biotechnol. 2011;10(45):8984–9000.
- Zhou J, Ma H, Guo F, Luo X. Effect of thidiazuron somatic embryogenesis of Cayratia japonica. Plant Cell Tissue Organ Cult. 1994;36:73–79.
- Sankhla D, Davis TD, Sankhla N. Thidiazuron induced in vitro shoot formation from roots of intact seedlings of Albizzia julibrissin. Plant Growth Regul. 1994;14:267–272.
- Mroginski E, Rey HY, Gomzalez AM, Mroginski LA. Thidiazuron promotes in vitro plant regeneration of Arachis correntina (Leguminosae) via organogenesis. Plant Growth Regul. 2004;23:129–134.
- Prathanturarug S, Soonthornchareonon N, Chauakul W, Phaidee Y, Saralamp P. Rapid micropropagation of Curcuma longa L. using bud explants pre-cultured in thidiazuron- supplemented liquid medium. Plant Cell Tissue Organ Cult. 2005;80:347–351.
- Thomas DT. Pretreatment in thidiazuron improves the in vitro shoot induction from leaves in Curculigo orchioides Gaertn., an endangered medicinal plant. Acta Physiol Plant. 2007;29:455–461.
- Faisal M, Alatar AA, Hegazy AK, Alharbi SA, El Sheikh M, Okla MK. Thidiazuron induced in vitro multiplication of Mentha arvensis and evaluation of genetic stability by flow cytometry and molecular markers. Ind Crops Prod. 2014;62:100–106.
- Khalafalla MM, Hattori K. A combination of thidiazuron and benzyladenine promotes multiple shoot production from cotyledonary node explants of faba bean (Vicia faba L.). Plant Growth Regul. 1999;27:145–148.
- Shahzad A, Ahmad N, Rather MA, Husain, MK, Anis, M. Improved shootregeneration system through leaf derived callus and nodule culture of Sanse-vieria cylindrica. Biol Plant. 2009;53,745–749.
- Ahmad N, Faisal M, Anis M. Role of PGR on in vitro shoot propagation in Cyamopsis tetragonoloba L. (Taub.): a drought tolerant grain legume Rend. Fis Acc Lincei. 2013;24,7–12.
- Rival A, Beule T, Lavergue D, Nato A, Havaux M, Puard M. Development of photosynthetic characteristics in oil palm during in vitro micropropagation. J Plant Physiol. 1997;150:520–527.
- Pospisilova J, Wilhelmova N, Synkova H, Catsky J, Krebs D, Ticha I, Hanackova B, Snopek J. Acclimation of tobacco plantlets to ex vitro conditions as affected by application of abscisic acid. J Exp Bot. 1998;49:863–869.
- Farnese FS, Oliveira JA, Farnese MS, Gusman GS, Silveira NM, Siman LI. Uptake arsenic by plants: Effects on mineral nutrition, growth and antioxidant capacity. IDESIA (Chile) Enero-Febrero 2014;32(1):99–106.
- Van Huylenbroeck JM, Van Laere IMB, Piqueras A, Debergh PC, Bueno P. Time course of catalase and superoxide dismutase during acclimatization and growth of micropropagated Calathea and Spathiphyllum plants. Plant Growth Regul. 1998;26:7–14.
- Diaz-Perez JC, Sutter EG, Shackel KA. Acclimatization and subsequent gas exchange water relations, survival and growth of microcultured apple plantlets after transplanting them in soil. Physiol. Plant. 1995;95:225–232.
- Estrada-Luna AA, Davies Jr FT, Egilla JN. Physiological changes and growth of micropropagated Chile and pepper plantlets during acclimatization and post acclimatization. Plant Cell Tissue Organ Cult. 2001;66:17–24.
- Dat J, Vandenabeele S, Vranová E, Van Montagu M, Inzé D, Van Breusegem F. Dual action of the active oxygen species during plant stress responses. Cell Mol Life Sci. 2000;57:779–795.
- Mitrović T, Stamenković S, Cvetković V, Nikolić M, Basosić R, Mutić J, Andelković A, Bojić A. Epiphytic lichen Flavoparmelia caperata as a sentinel for trace metal pollution. J Serbian Chem Soc. 2012;77(9):1301–1310.
- Varshney A, Anis M. Improvement of shoot morphogenesis in vitro and assessment of changes of the activity of antioxidant enzymes during acclimation of micropropagated plants of desert teak. Acta Physiol Plant 2012;34:859–867.
- Ahmed MR, Anis M. Changes in activity of antioxidant enzymes and photosynthetic machinery during acclimatization of micropropagated Cassia alata L. plantlets. In Vitro Cell Dev Biol-Plant. 2014;50:601–609.