Abstract
Although protein disulphide isomerase (PDI) is a well-known multifunctional protein, the precise mechanism of its response to viral infections has not been fully elucidated, especially in veterinary research. Therefore, in this study we developed a real-time polymerase chain reaction (PCR) method for the simultaneous detection of PDI expression in porcine cell lines and tissues. Several primers were designed to amplify a specific nucleotide sequence of PDI and to develop a PCR-based biotechnology platform in macrophages. The sensitivity of the assay for detecting PDI was determined to be in the range of 108–101 genomic units. Our results demonstrated that this method could be used to accurately quantify PDI expression and to examine its effects on infection responses to classical swine fever virus (CSFV) in porcine tissue. PDI expression was found to be inhibited in the heart, liver, spleen, lung, kidney and mesenteric lymph node tissue from a CSFV-positive pig. These data contribute to an improved understanding of the mechanism of CSFV pathogenesis.
Introduction
Protein disulphide isomerase (PDI) is a multifunctional protein expressed mainly in the endoplasmic reticulum (ER) of eukaryotic cells, which consists of 517 amino acids and includes four thioredoxin-like domains (termed as a, b, b′ and a′), a linker (x) and a C-terminal extension domain (c), which are arranged in the order abb′xa′c.[Citation1] The domains a and a′ contain a -Cys-Gly-His-Cys- sequence similar to that of thioredoxin, and this sequence is the active site of the isomerase.[Citation2] The individual catalytic domains a and a' have a very low isomerase activity.[Citation3] The domains b and b' bind the substrate through hydrophobic interactions.[Citation4] PDI's structure determines its protein function.[Citation5,Citation6] PDI acts as an oxidase and forms disulphide bonds in nascent secretory proteins in the ER.[Citation7] In endosomes and on the cell surface, PDI acts primarily as a reductase to cleave disulphide bonds in proteins.[Citation8]
Most of the protein folding and maturation processes that occur in the lumen of the ER require the participation of PDI.[Citation9,Citation10] High expression of PDI can protect the cells and tissues from damage by reducing stress and inhibiting apoptosis.[Citation11] Recent studies have shown that PDI is likely indispensable as a molecular chaperone to assist in the correct folding of viral proteins in virus-infected cells.[Citation12,Citation13] In addition, PDI plays an important role in the host cell invasion process by HIV-1 and the dengue virus.[Citation14,Citation15] For example, during the entry of HIV-1 into the host cells, the fusion of HIV-1 capsule membrane and the cell membrane depends on the PDI functional sites.[Citation16] During the virus–cell interaction period, PDI inhibitors could prevent decomposition of disulphide bonds and prevent the entry of HIV-1.[Citation17]
As PDI attracts increasing research interest, future projects may focus on the molecular regulation of PDI. Thus, an analytic method for rapid and accurate detection of PDI will facilitate research into the relationship between PDI and various diseases. In the present study, a real-time polymerase chain reaction (PCR) method was developed for the detection of PDI at a transcription level, using porcine tissues and cell lines. Interestingly, PDI expression was found to be suppressed in various pig tissues when they were infected by the Shimen strain of the classical swine fever virus (CSFV).
Materials and methods
Cell lines, viral strains and pig tissues
Macrophages (CRL-2845) were obtained from the American Type Culture Collection (ATCC, Rockville, MD, USA). The Shimen strain of CSFV was obtained from the Control Institute of Veterinary Bioproducts and Pharmaceuticals (China).
The experimental pigs were randomly divided into two groups: a group that was challenged with the Shimen strain of CSFV and a negative control group that was administered aseptic physiological saline.
Cell culture
Macrophages were cultured in RPMI 1640 medium (Gibco Life Technologies, Carlsbad, CA, USA) containing 2 mmol/L L-glutamine, 1.5 g/L sodium bicarbonate, 4.5 g/L glucose, 10 mmol/L N-2-hydroxyethylpiperazine-N-ethanesulphonic acid (HEPES), 1.0 mmol/L sodium pyruvate, 0.1 mmol/L non-essential amino acids and 10% fetal bovine serum (FBS). The macrophages were maintained at 37 °C in a humidified atmosphere with 5% CO2. The culture medium was changed every 1 or 2 days, and when the cell proliferation reached 70% confluence, the cells were homogenized in TRIzol (Takara, Dalian, China).
RNA extraction and reverse transcription
Macrophagocytes were homogenized in TRIzol, and 200 µL chloroform was added to the mixture. Shaking tubes were vigorously carted out for 15 s and then incubated at room temperature for 5 min. Samples were centrifuged at 15,000 × g for 15 min at 4 °C. After the upper transparent liquid was carefully removed, an equal volume of isopropanol was added. The resulting mixture was transferred into new tubes, inverted and kept at room temperature for 10 min. The samples were centrifuged at 11,500 × g for 10 min at 4 °C and the supernatant was removed. The RNA pellet was washed once with 75% ethanol, after this 1 mL of 75% ethanol was added, and the samples were centrifuged at 11,500 × g for 5 minutes at 4 °C. The purified RNA was dissolved in RNase-free water. Messenger RNA was transcribed using the Superscript II enzyme (Invitrogen, Life Technologies, Carlsbad, CA, USA), according to the manufacturer's instructions.
Design of primers for amplification of the PDI gene
The swine PDI sequence published in the GenBank database (NM_001195112) was used to select a 224-bp region with high homology as a target for real-time PCR amplification. Primer Premier 5.0 (Premier Biosoft International, Palo Alto, CA, USA) was used for primer design. The PDI-specific primers, PDI-F (5′-GCAGCCAGCAACTTGAGGGA-3′) and PDI-R (5′-TGTCATGTGAGGGCAGATACCA-3′) were used to obtain 224-bp amplicons.
Amplification of the target gene
The PDI sequence was amplified by a conventional PCR. The conditions for the PCR were as follows: 94 °C for 4 min, 35 cycles at 94 °C for 30 s, 57 °C for 30 s, 72 °C for 30 s and a final extension at 72 °C for 10 min. The PCR reaction was performed in a total volume of 25 μL, containing 4 μL PDI DNA preparation, 12.5 μL 2 × Taq MasterMix (Beijing ComWin Biotech Co., Ltd) and 2 μL PDI sequence primer solution. The purified PCR product was subjected to electrophoresis, according to the DNA gel extraction kit instructions. The DNA marker was Trans2K Plus DNA Marker (TransGen Biotech, Beijing, China).
Plasmid construction
The amplified fragment was cloned into a pMD19-T Simple Vector (TaKaRa, Dalian, China) and then transformed into DH-5α competent cells (TaKaRa, Dalian, China). The positive clones were screened using ampicillin and expanding culture. Ten microliters of the extracted plasmid was sent to Beijing Kuni Genomics Services Limited for sequencing. The copy number was calculated according to the formula, after detecting the standard concentration of the plasmid using a UV spectrophotometer (Thermo Spectronic, Madison, WI, USA).
Real-time PCR assays
Real-time PCR was performed in a final volume of 20 μL, containing 1 μL of the plasmid, 10 μL of TransStart Tip Green qPCR SuperMix (Tiandz, Beijing, China), 0.5 μL of primer solution and 8 μL of water. The amplification conditions were as follows: 95 °C for 30 s, 40 cycles at 95 °C for 10 s, 60 °C for 30 s and a final extension at 72 °C for 5 min. The real-time PCR reactions were performed using a BIO-RAD iQ5 multicolor real-time detection system (Bio-Rad, Hercules, CA, USA).
Sensitivity and reproducibility
To determine the detection sensitivity, the experiment was run in triplicate for each dilution in the range of 108–101 genomic units for obtaining the lowest copy number. To evaluate the intra-assay and inter-assay reproducibility of the real-time PCR assay, amplifications were carried out in fast reaction tubes (eight tubes/strip) (Applied Biosystems, Forster City, CA, USA) and tests were performed using two sets of plasmid standards, eight high concentrated and eight low concentrated controls (106 copies/µL and 104 copies/µL). The intra-assay coefficient of variation (CV) reported in the present study was an average value calculated from the individual CVs for all of the duplicates () in which the cycle threshold (Ct) value of each plasmid sample was detected twice – as Result 1 and as Result 2. The inter-assay CV was an expression of consistency that was calculated from the mean values for the high-concentrated and low-concentrated controls of plasmid samples, as illustrated in .
Table 1. Intra-assay statistical results of plasmid standard detection by real-time PCR.
Table 2. Inter-assay statistical results of plasmid standard detection by real-time PCR.
Pig tissues sample assays
Total RNA was extracted from the heart, liver, spleen, lung, kidney and mesenteric lymph node tissue of CSFV-positive and CSFV-negative pig tissues and then reverse-transcribed into cDNA.[Citation18] The positive and negative tissues for CSFV infection were identified using a previously described method.[Citation19] PDI expression was detected using an established quantitative PCR method.
Results and discussion
PDI cloning and identification
The cloned PCR product of the PDI gene was subjected to electrophoresis, and bands near the expected size of 224 bp were obtained (). The extracted plasmid was sent to Beijing Kuni Genomics Services Limited to be sequenced after cloning of the target fragment. The sequencing results indicated that it was 99.9% homologous to the reference sequence (data not shown).
Sensitivity
The experiment for the sensitivity evaluation was run in the range of 108–101 genomic units for 40 cycles, using the appropriate standards. The results indicated that the assay could accurately detect the presence of PDI at a concentration of 1.0 × 101 copies/µL and showed that the assay was highly sensitive (). The real-time PCR method relies on the visual detection of the amplification products by displaying a fluorescent signal produced either by intercalating dyes, which indicate the presence of double-stranded DNA, or by specific dual-labelled probes.[Citation20,Citation21] Due to its widely recognized high sensitivity,[Citation22,Citation23] real-time PCR has become an important tool in molecular biology researches.
Figure 2. Test for sensitivity of real-time PCR for protein disulphide isomerase (PDI) detection.
Note: Representative amplification plots are shown. 1–8 represent the standard curves based on 10-fold serial dilutions of between 108 and 101 plasmid equivalents: 1.0 × 108 copies/µL (1), 1.0 × 107 copies/µL (2), 1.0 × 106 copies/µL (3), 1.0 × 105 copies/µL (4), 1.0 × 104 copies/µL (5), 1.0 × 103 copies/µL (6); 1.0 × 102 copies/µL (7); and 1.0 × 101 copies/µL (8).
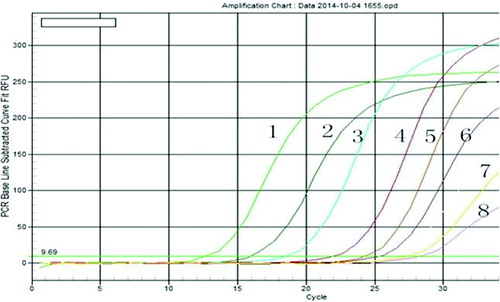
Reproducibility
Intra-assay reproducibility was verified by performing independent intra-laboratory intra-assay comparisons. The percentage CVs for each sample were calculated by determining the standard deviation of the results (). The average of the individual CVs (0.58) was reported as the intra-assay CV. To determine the inter-assay reproducibility, plasmid standards of high (1.0 × 106 copies/µL) and low (1.0 × 104 copies/µL) concentrations were used to calculate the inter-assay CVs. As shown in , the percentages of the CV value (0.60) showed acceptable inter-laboratory discordance. This study aimed to establish an accurate, rapid and efficient method for detection of PDI, such as fluorescent quantitative PCR, based on a strong linear relationship between changes in fluorescence and changes in concentration of the target gene. This method showed good intra-assay and inter-assay reproducibility.
Quantitative detection of PDI in experimental pigs
Tissue samples from pigs experimentally infected with the Shimen strain of CSFV and mock-infected pigs were quantitated using an established quantitative PCR method; heart, liver, spleen, lung, kidney and mesenteric lymph node tissues were tested. First, viral distribution in the different tissues was evaluated. As shown in , viral RNA could be detected at a low relative expression levels in the heart, liver, lung and kidney samples; the highest levels of viral RNA were detected in the spleen and mesenteric lymph node samples of all infected pigs, using a previously reported detection method.[Citation19] CSFV has been reported to show different proliferation characteristics in different host tissues, because it is known to show tissue tropism.[Citation24,Citation25] The results shown in are concordant with the previously reported results.
Figure 3. CSFV-infected field samples analysed using a real-time PCR.
Note: Low relative expression levels of viral RNA were distributed among the heart, liver, lung and kidney samples; higher relative expression levels of viral RNA were detected in the spleen and lymph node samples of all infected pigs.
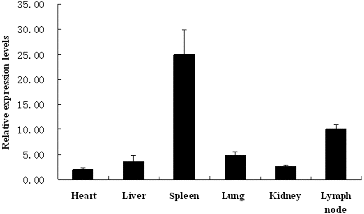
Changes in expression of PDI were detected in CSFV-positive and CSFV-negative pig tissues, using an established quantitative PCR method. Significant differences (P < 0.05) in the expression of PDI were observed among different pig tissues. Interestingly, infection with CSFV reduced the expression of PDI in the heart, liver, spleen, lung, kidney and mesenteric lymph node tissues of the pig ().
Figure 4. Quantification of protein disulphide isomerase (PDI) by real-time PCR in classical swine fever virus (CSFV)-positive and CSFV-negative tissues.
Note: Relative expression levels of PDI in CSFV-positive pig tissues were lower than those in CSFV-negative pig tissues, with regard to heart, liver, spleen, lung, kidney and lymph node tissue of the pig.
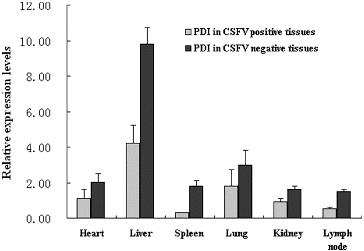
The liver is known to be the primary organ involved in metabolic function and to play a key role in the synthesis of secreted proteins.[Citation26] In this study, PDI expression was found to be the highest in the liver tissues of CSFV-negative swine, consistent with its biological function.
PDI expression in the tissues of CSFV-positive swine was found to be significantly lower than that in the tissues of CSFV-negative swine, indicating that CSFV could downregulate the expression of PDI. Viral proteins can disturb the correct folding of proteins in host cells.[Citation27] As a molecular chaperone protein, PDI may assist in correct protein folding.[Citation28] Thus, CSFV may affect the expression of PDI as a part of its mechanism of pathogenesis.
Conclusion
Currently, emergent viral strains are a subject of great public concern, and researches into the mechanism by which viral and host cell proteins interact are attracting greater interest. PDI plays an important role in the process of virion fusion with host cells. However, little attention has been focused on veterinary research, especially on CSFV. Research in this field may help build an effective approach for detection of PDI. In the present study, a real-time PCR method was established that offered an analytical tool to investigate a novel mechanism underlying the pathogenesis of classical swine fever via a study of the molecular chaperone protein PDI.
Disclosure statement
The authors declare that they have no competing interests.
Additional information
Funding
References
- Gruber CW, Cemazar M, Heras B, Martin JL, Craik DJ. Protein disulfide isomerase: the structure of oxidative folding. Trends Biochem Sci. 2006;31(8):455–464.
- Vuori K, Myllylä R, Pihlajaniemi T, Kivirikko KI. Expression and site-directed mutagenesis of human protein disulfide isomerase in Escherichia coli. This multifunctional polypeptide has two independently acting catalytic sites for the isomerase activity. J Biol Chem. 1992;267(11):7211–7214.
- Darby NJ, Penka E, Vincentelli R. The multi-domain structure of protein disulfide isomerase is essential for high catalytic efficiency. J Mol Biol. 1998;276(1):239–247.
- Xiao R, Solovyov A, Gilbert HF, Holmgren A, Lundström-Ljung J. Combinations of protein-disulfide isomerase domains show that there is little correlation between isomerase activity and wild-type growth. J Biol Chem. 2001;276(30):27975–27980.
- Hoffstrom BG, Kaplan A, Letso R, Schmid R, Turmel GJ, Lo DC, Stockwell BR. Inhibitors of protein disulfide isomerase suppress apoptosis induced by misfolded proteins. Nat Chem Biol. 2010;6(12):900–906.
- Ellerman DA, Myles DG, Primakoff P. A role for sperm surface protein disulfide isomerase activity in gamete fusion: evidence for the participation of ERp57. Dev Cell. 2006;10(6):831–837.
- Muller C, Bandemer J, Vindis C, Camare C, Mucher E. Protein disulfide isomerase modification and inhibition contribute to ER stress and apoptosis induced by oxidized low density lipoproteins. Antioxid Redox Signal. 2013;18:731–742.
- Xu S, Butkevich AN, Yamada R, Zhou Y, Debnath B. Discovery of an orally active small-molecule irreversible inhibitor of protein disulfide isomerase for ovarian cancer treatment. Proc Natl Acad Sci USA. 2012;109:16348–16353.
- Vekich JA, Belmont PJ, Thuerauf DJ, Glembotski CC. Protein disulfide isomerase-associated 6 is an ATF6-inducible ER stress response protein that protects cardiac myocytes from ischemia/reperfusion-mediated cell death. J Mol Cell Cardiol. 2012;53:259–267.
- Tanaka LY, Araújo HA, Csordas AA, Hironaka GK, Takimura CK. Role of protein disulfide isomerase during vascular repair after injury. FASEB J. 2012;26:1114–1137.
- Tanaka S, Uehara T, Nomura Y. Up-regulation of protein-disulfide isomerase in response to hypoxia/brain ischemia and its protective effect against apoptotic cell death. J Biol Chem. 2000;275:10388–10393.
- Ping Y, Lüpken T, Habekuss A, Hensel G, Steuernagel B, Kilian B, Ariyadasa R, Himmelbach A, Kumlehn J, Scholz U, Ordon F, Stein N. Protein disulfide isomerase like 5-1 is a susceptibility factor to plant viruses. Proc Natl Acad Sci USA. 2014;111:2104–2109.
- Hasegawa G, Suwa M, Ichikawa Y, Ohtsuka T, Kumagai S. A novel function of tissue-type transglutaminase: protein disulphide isomerase. Biochem J. 2003;373:793–803.
- Wan SW, Lin CF, Lu YT, Lei HY, Anderson R, Lin YS. Endothelial cell surface expression of protein disulfide isomerase activates β1 and β3 integrins and facilitates dengue virus infection. J Cell Biochem. 2012;113(5):1681–1691.
- Bi S, Hong PW, Lee B, Baum LG. Galectin-9 binding to cell surface protein disulfide isomerase regulates the redox environment to enhance T-cell migration and HIV entry. Proc Natl Acad Sci USA. 2011;108(26):10650–10655.
- Khan MM, Simizu S, Lai NS, Kawatani M, Shimizu T. Discovery of a small molecule PDI inhibitor that inhibits reduction of HIV-1 envelope glycoprotein gp120. ACS Chem Biol. 2011;6:245–251.
- Stantchev TS, Paciga M, Lankford CR, Schwartzkopff F, Broder CC, Clouse KA. Cell-type specific requirements for thiol/disulfide exchange during HIV-1 entry and infection. Retrovirology. 2012;9:97.
- Deprez RHL, Fijnvandraat AC, Ruijter JM, Moorman AF. Sensitivity and accuracy of quantitative real-time polymerase chain reaction using SYBR green I depends on cDNA synthesis conditions. Anal Biochem. 2002; 307(1): 63–69.
- Ning P, Li H, Liang W, Guo K, Tan X, Cao W, Cheng L, Zhang Y. Detection and differentiation of classical swine fever virus strains C and Shimen by high-resolution melt analysis. J Virol Methods. 2013;194(1):129–131.
- Gonzalez O, Fontanes V, Raychaudhuri S, Loo R, Loo J. The heat shock protein inhibitor Quercetin attenuates hepatitis C virus production. Hepatology. 2009;50:1756–1764.
- Omiccioli E, Amagliani G, Brandi G, Magnani M. A new platform for real-time PCR detection of Salmonella spp., Listeria monocytogenes and Escherichia coli O157 in milk. Food Microbiol. 2009;26(6):615–622.
- Pfaff MW, Horgan GW, Dempfle L. Relative expression software tool (REST) for group-wise comparison and statistical analysis of relative expression results in real-time PCR. Nucleic Acids Res. 2002;30:e36.
- Mackay IM, Arden KE, Nitsche A. Real-time PCR in virology. Nucleic Acids Res. 2002;30:1292–1305.
- Sheng C, Zhu Z, Yu J, Wan L, Wang Y. Characterization of NS3, NS5A and NS5B of classical swine fever virus through mutation and complementation analysis. Vet Microbiol. 2010;140:72–80.
- Liu J, Fan XZ, Wang Q, Xu L, Zhao QZ. Dynamic distribution and tissue tropism of classical swine fever virus in experimentally infected pigs. Virol J. 2011;8:201.
- Campbell I. Liver: metabolic functions. Anaesth Intensive Care Med. 2006;7(2): 51–54.
- Gottipati K, Ruggli N, Gerber M, Tratschin JD, Benning M, Bellamy H, Choi KH. The structure of classical swine fever virus N(pro): a novel cysteine Autoprotease and zinc-binding protein involved in subversion of type I interferon induction. PLoS Pathog. 2013;9(10):e1003704.
- Tu BP, Weissman JS. Oxidative protein folding in eukaryotes: mechanisms and consequences. J Cell Biol. 2004;164(3):341–346.