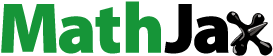
Abstract
In this study, the orthogonal experimental design was used to determine the optimum conditions for the effect of thermal-alkaline pretreatment on the anaerobic digestion of penicillin bacterial residue. The biodegradability of the penicillin bacterial residue was evaluated by biochemical methane potential tests in laboratory. The optimum values of temperature, alkali concentration, pretreatment time and moisture content for the thermal-alkaline pretreatment were determined as 70 °C, 6% (w/v), 30 min, and 85%, respectively. Thermal-alkaline pretreatment could significantly enhance the soluble chemical oxygen demand solubilization, the suspended solid solubilization and the biodegradability. Biogas production was enhanced by the thermal-alkaline pretreatment, probably as a result of the breakdown of cell walls and membranes of micro-organisms, which may facilitate the contact between organic molecules and anaerobic microorganisms.
Abbreviations
BMP | = | biochemical methane potential; |
SCOD | = | soluble chemical oxygen demand; |
SCODf | = | final soluble chemical oxygen demand; |
SCODi | = | initial soluble chemical oxygen demand; |
SS | = | suspended solid; |
SSf | = | final suspended solid; |
SSi | = | initial suspended solid; |
TAN | = | total ammonia nitrogen; |
TCOD | = | total chemical oxygen demand; |
TNK | = | total nitrogen Kjeldahl; |
TSS | = | total suspended solid; |
VFA | = | volatile fatty acid; |
TS | = | total solid; |
VS | = | volatile solid; |
VSS | = | volatile suspended solid; |
Introduction
At present, China has become the world's largest producer and exporter of antibiotics. Data show that China's production of all kinds of antibiotics was 1.21 million tons in 2007. With the increasing pressure of the environmental issues in recent years, dealing with antibiotic bacterial residue in a safe, reasonable and effective way is a serious problem, which needs an urgent solution.[Citation1–4]
Until 2008, most fresh bacterial residues were directly used as feed or feed additives after drying. However, nowadays this practice has been banned. The burning of bacterial residues can quickly reduce the amount of antibiotics and remove the harmful material, but the prices of the special incineration equipment are too high.[Citation1,Citation5] Many antibiotic bacterial residues, which can be used for biogas formation by anaerobic digestion [Citation2,Citation6] are rich in easily degradable material. The feasibility of the anaerobic digestion of various bacterial residues has been investigated by many researchers.[Citation4,Citation7,Citation8]
The hydrolysis process is the rate-limiting step in the process of anaerobic digestion. Therefore, the increase in hydrolysis rate of yeast is becoming the primary problem for improving the efficiency of the anaerobic digestion. Many studies [Citation9,Citation10] have shown that the anaerobic biogas production from biomass is impeded by the hard cell wall of the bacterial residues. To improve the overall substrate degradability, pretreatment or disintegration of the penicillin bacterial residues is required. Various disintegration techniques, including thermal, ultrasonic, microwave, mechanical, ozone, alkali and enzyme pretreatments have been successfully applied as methods for the enhancement of the anaerobic digestibility.[Citation10–15] Thermal pretreatment destroys the microbial cell walls and releases the intracellular organic matter. This method maximizes the conversion of insoluble organic matter into soluble organic matter. At the same time, it also shortens the duration of the hydrolysis process and accelerates the hydrolysis acidification and gas formation. In this way, the anaerobic fermentation process becomes more efficient and increases the biogas production. Alkaline pretreatment is probably the best known efficient method for enhancing the biodegradation of complex materials.[Citation3,Citation16–21] NaOH has been found to be the most effective for lignin removal and biogas production.[Citation22–24] Thermal-alkaline pretreatment could promote the dissolving and decomposition of microbial cells and organic materials so as to improve the anaerobic digestibility.[Citation3,Citation25,Citation26] Our previous study results [Citation1,Citation3] showed that the optimal conditions of the thermal-alkaline pretreatment of the streptomycin bacterial residue were 0.10 NaOH/ total solid (TS) ratio (g/g) at 70 °C for 2 h. The quantity of the solid-phase bacterial residue was reduced and the soluble organic material in the liquid was increased; therefore, the thermal-alkaline pretreatment method could be used as an effective method for dealing with bacterial residues.
To our knowledge, there have been no reports about an anaerobic digestion of penicillin bacterial residue. It is recognized that the rate-limiting step of anaerobic digestion processes for the antibiotics bacterial residue with high mycelium and solid contents is the hydrolysis of the complex organic matter to soluble compounds.[Citation3] To increase the efficiency of the anaerobic digestion of the penicillin bacterial residue, thermal-alkaline pretreatment methods were used. The soluble chemical oxygen demand (SCOD) and suspended solid (SS) solubilizations efficiencies of thermal and thermal-alkaline pretreatments of the penicillin bacterial residues were studied. This study was aimed at providing preliminary information on biogas yields from the anaerobic digestion of the filamentous penicillin bacterial residues. An additional aim of this work was to study the effect of the thermal-alkaline pretreatment of penicillin bacterial residue on the anaerobic digestion for methane production.
Materials and methods
Feedstock
The penicillin bacterial residue was obtained from the North China Pharmaceutical Company, Hebei, China, which was stored in a refrigerator at 4 °C prior to use. The anaerobic sludge, which was used as inoculum, was taken from an anaerobic digester with 5.0 L working volume, which had been cultivated and used in a previous digestion process of penicillin bacterial residue at 35 °C, with 10 days retention time. The characteristics of the penicillin bacterial residue and the anaerobic sludge were analysed before the experiment and are listed in .
Table 1. Main properties of the inoculated anaerobic granular sludge and the penicillin bacterial residue.
Pretreatment
This experiment mainly considered four factors and three levels, using L9 (43) orthogonal table to screen the best pretreatment method. The orthogonal test factors and levels are shown in and the test conditions are shown in . The thermal-alkaline pretreatment method was as follows: 57.45, 38.3 and 19.15 g of the penicillin bacterial residue were taken into three 150 mL serum bottles. In each bottle, the moisture content was 85%, 90% and 95%, respectively. After this, 2.0 g (2%), 4.0 g (4%) and 6.0 g (6%) of NaOH were added into the serum bottles, respectively. Distilled water was added up to a working volume of 100 mL. Then, each of the serum bottles was placed at 25, 70 and 100 °C and each of them had a reaction time of 30, 60 and 120 min. An unpretreated penicillin bacterial residue (38.3 g) was also put into two 150 mL serum bottles, as blank controls.
Table 2. Orthogonal test factors and levels.
Table 3. Scientific experimental conditions obtained from nine representative sample sets.
The effectiveness of the thermal-alkaline pretreatment on the solubilization of the samples was evaluated based on the solubilization degree, i.e. the quantity of the soluble organic matter expressed as SCOD solubilization and SS solubilization, which were calculated as follows:where SCODf is the final soluble chemical oxygen demand (mg/L) and SCODi is the initial soluble chemical oxygen demand (mg/L) [Citation3];
where SSi is the initial suspended solid (mg/L) and SSf is the final suspended solid (mg/L).
Biochemical methane potential test
BMP test was performed to determine the ultimate CH4 yield of the organic substrate and to monitor the biochemical methane potential. During the BMP experiment (anaerobic digestion to produce methane potential), each sample was mixed in a 150 mL serum bottle with 20 mL of pretreated penicillin bacterial residue, 70 mL of mesophilic microbial inoculum and 30 mL of deionized water. Then, the bottles were gassed with 0.5 L/min N2 for 10 min, which was added to ensure anaerobic conditions. The CH4 gas was collected through the serum bottles' liquid displacement system. The methane displaced a measurable volume of NaOH solution from the reservoir, which was the methane volume. The BMP test lasted for 30 days and was repeated in triplicate.
Analytical methods
The pH value was directly measured with type FE20 laboratory pH meter. TS, volatile solid (VS), volatile suspended solid (VSS), SCOD, total chemical oxygen demand (TCOD) and total nitrogen Kjeldahl were determined based on the Chinese Standard. Volatile fatty acid analysis using gas chromatography was performed as previously described.[Citation4] Total ammonia nitrogen was measured in triplicate, according to the American Public Health Association standard methods.[Citation27] Each analytical result was the mean value of at least three measurements.
Results and discussion
Effect of thermal-alkaline pretreatment on disintegration of penicillin bacterial residues
It can be seen from that the pH value of the penicillin antibiotics residue without thermal-alkaline pretreatment was about 13. On the other hand, the pH value of the penicillin antibiotics residue with thermal-alkaline pretreatment was lower than the one without the pretreatment. The reason for this could be that the thermal-alkaline pretreatment could promote the dissolving and decomposition of microbial cells, which may release intracellular acidic substances.
Figure 1. Change of SCOD solubilization and pH.
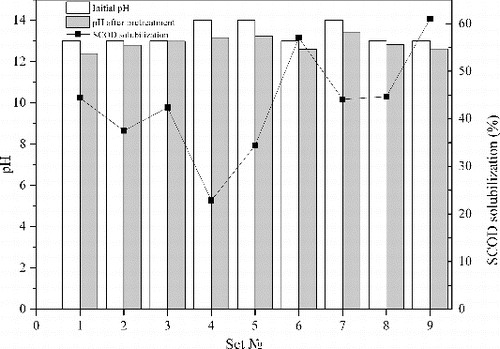
and show the SCOD and SS solubilizations after the thermal-alkaline pretreatment, respectively. It can clearly be seen that the ninth test (see ) reached the highest SCOD solubilization–61.5%. The results also showed that during the sixth test, the solubilization rate of SCOD was 58.3%. The conditions of the sixth test were 70 °C reaction temperature, 6% (w/v) alkali concentration, 30 min pretreatment time and an initial pH value of 13. We can also see from that the SCOD solubilization of the third, the sixth and the ninth sets was 42.4%, 58.3% and 61.5%, respectively, and the SS solubilization () of these sets was 54.6%, 60.9% and 57.5%, respectively. The pH played a very important role in the thermal-alkaline pretreatment.
Figure 2. Change of SS solubilization during the nine experiments with different conditions.
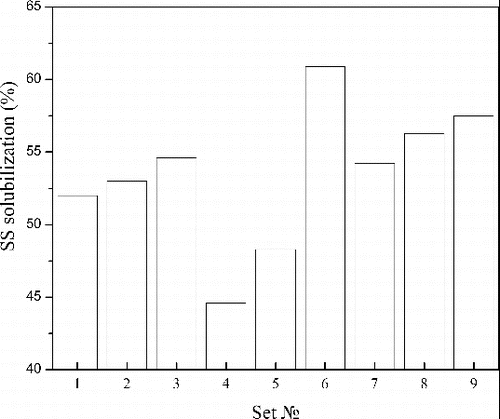
The first purpose of the penicillin bacterial residue pretreatment was the solid volume reduction, which decreased the solid content of the penicillin bacterial residue. Combined alkali thermal process with the addition of different concentrations of sodium hydroxide can abate the bacterial cell walls of slag, which are resistant to high temperatures. This makes the cell walls more susceptible to damage and the intracellular substances more dissolvable in water. On the other hand, this process can stabilize, modulate and reduce the size of the penicillin bacterial residue.
According to these results, it could be concluded that the thermal-alkaline pretreatment significantly enhanced the amount of the soluble organic matter from the penicillin bacterial residue. The SCOD and SS solubilizations of the penicillin bacterial residue with thermal-alkaline pretreatment were almost 4.1– 7.9 times higher than the solubilization with thermal pretreatment only.[Citation3]
Effects of thermal-alkaline pretreatment on biogas production yields in the anaerobic digestion of penicillin bacterial residues
The cumulative biogas production of the untreated and thermal-alkaline pretreated samples during the BMP test is shown in . As it can be seen, the biogas production was significantly increased immediately in all set groups. The daily biogas production gradually decreased after 15 days of digestion, and most of the biogas was produced before the 15th day of digestion. Biogas production of the thermal-alkaline pretreated penicillin bacterial residue started earlier and lasted longer time, whereas the biogas production of the penicillin bacterial residue with no pretreatment started later and lasted less time. This could be due to the destruction of lignin and cellulose of the penicillin bacterial residue due to the thermal-alkaline pretreatment. This may enable the pretreated penicillin bacterial residue to exhibit a good process stability and a high conversion efficiency for the anaerobic digestion. The biogas energy was determined by both biogas volume and methane content. The methane content for all groups was measured during the whole digestion period.
Figure 3. Accumulated biogas production for 30 days at different pretreatment conditions and at conditions without pretreatment.
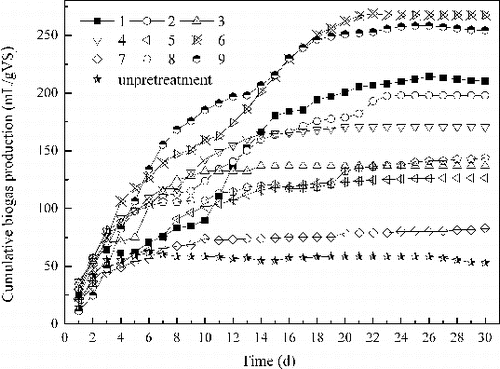
Biogas production rate is an important indicator that reflects the anaerobic digestion performance. The greater the biogas production rate, the more biomass could be recycled. As shown in , at different heating temperatures during the thermal-alkaline pretreatment, the production rate of biogas changed. shows that during the process of anaerobic digestion in the BMP test, after pretreatment of the penicillin bacterial residue, the biogas and methane production rate became higher than that of the control group, especially in the sixth and in the ninth test groups. After 30 days in the sixth and in the ninth test groups, the biogas production rate reached 267.5 and 249.6 mL/gVS, respectively, and the methane production rate reached 201.2 mLCH4/gVS and 193.6 mL CH4/gVS, respectively. Compared with the biogas production rate of the non-pretreated penicillin bacterial residue (52.9 mL/gVS), the sixth and the ninth test groups had increased their biogas production rate by 5.06 and 4.72 times, respectively. Compared with the methane production rate of the non-pretreated penicillin bacterial residue (22.1 mLCH4/gVS), the sixth and the ninth test groups had increased their methane production rate by 9.10 and 8.76 times, respectively.
Figure 4. Biogas production and methane production after 30 days at different pretreatment conditions.
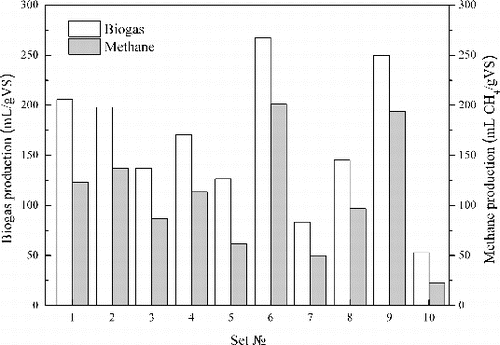
As it can be seen from , the sixth group had the best technology conditions, because its biogas production reached 267.5 mL/gVS. We selected the biogas production as an observation value. The results from the orthogonal experiment () were obtained from the nine representative sample sets showed in . From K value analysis, the A2 = 564.7, B3 = 654.0, C1 = 618.4; D1 = 581.8 showed in are all factors under the maximum. So, the best experimental scheme was: 70 °C temperature, 6% (w/v) alkali concentration, 30 min pretreatment time and 85% moisture content.
Table 4. Performance data during the anaerobic process of the penicillin bacterial residue as feedstock at different pretreatment conditions.
The different factors had distinct influence degree on the digestive process. The extreme value R was used to express the influence degree. The greater the R values, the bigger the influence degree. It can be seen from that RA was only 86.5, which was lower than RB, RC and RD. It illustrated that the influence of the temperature change was small, which showed that raising the temperature did not change significantly the anaerobic process. On the other hand, RC showed the opposite result– the longer the pretreatment time, the less the biogas production. As a result from the analysis above, we can conclude that the influence degree of the factors can be ordered as follows: pretreatment time > alkali concentration > moisture content > temperature.
Conclusions
Together with the previous findings, the results from this study helped to identify that the thermal-alkaline pretreatment could significantly enhance not only SCOD and SS solubilizations, but also the biodegradability. Thermal-alkaline pretreatment may enhance the breakdown of cell walls and membranes of micro-organisms in order to facilitate the contact between the organic molecules and the anaerobic micro-organisms. In this way, the biogas yield can be increased. At the sixth group (70 °C temperature, 6% alkali concentration, 30 min pretreatment time and 85% moisture content) and the ninth group (100 °C temperature, 6% alkali concentration, 60 min reaction time and 90% moisture content), the methane production rate reached 201.2 and 193.6 mLCH4/gVS, respectively. Compared with the methane production rate of the non-pretreated penicillin bacterial residue, it increased by 9.10 and 8.76 times, respectively.
Disclosure statement
No potential conflict of interest was reported by the authors.
Additional information
Funding
References
- Li ZX, Tian BK, Zuo JE, Yu X, Shen H, Wang Y, Zhao X. Progress in treatment and disposal technology of antibiotic bacterial residues. Environ Eng. 2012;30(2):72–75.
- Guo B, Gong L, Duan E, Liu R, Ren A, Han J, Zhao W. Characteristics of penicillin bacterial residue. J Air Waste Manage Assoc. 2012;62(4):485–488.
- Li ZX, Zuo JE, Tian BK, Yang JL, Yu X, Chen P, Zhao Y. Thermal-alkaline pretreatment on the decomposition of the streptomycin bacterial residue. Biotechnol Biotechnol Equip. 2012;26(3):2971–2975.
- Zhong WZ, Li ZX, Yang JL, Liu C, Tian BK, Wang Y, Chen P. Effect of thermal–alkaline pretreatment on the anaerobic digestion of streptomycin bacterial residues for methane production. Bioresour Technol. 2014;151:436–440.
- Cassone CG, Vongphachan V, Chiu S, Williams KL, Letcher RJ, Pelletier E, Crump D, Kennedy SW. In ovo effects of perfluorohexane sulfonate and perfluorohexanoate on pipping success, development, mRNA expression, and thyroid hormone levels in chicken embryos. Toxicol Sci. 2012;127(1):216–224.
- Berndes G, Hoogwijk M, van den Broek R. The contribution of biomass in the future global energy supply: a review of 17 studies. Biomass Bioenerg. 2003;25:1–28.
- Li LM, Chen Y, Ling GC, Wu ZZ. Studies on anaerobic biological treatment of rifampicin mycelia. Chin J Liaoning Univ. 1992;19(3):70–74.
- Li S, He C. A study of biogas formation of distillers grains and residue of kanamycin fermentation. J Microbiol. 1988;8(2):11–15.
- Carrere H, Dumas C, Battimelli A, Batstone D, Delgenes J, Steyer J, Ferrer I. Pretreatment methods to improve sludge anaerobic degradability: a review. J Hazard Mater. 2010;183(1):1–15.
- Saha M, Eskicioglu C, Marin J. Microwave, ultrasonic and chemo-mechanical pretreatments for enhancing methane potential of pulp mill wastewater treatment sludge. Bioresour Technol. 2011;102(17):7815–7826.
- González-Fernández C, Sialve B, Bernet N, Steyer JP. Comparison of ultrasound and thermal pretreatment of Scenedesmus biomass on methaneproduction. Bioresour Technol. 2012;110:610–616.
- Kavitha S, Adish Kumar S, Yogalakshmi KN. Effect of enzyme secreting bacterial pretreatment on enhancement of aerobic digestion potential of waste activated sludge interceded through EDTA. Bioresour Technol. 2013;150:210–219.
- Maria S, Joan G, Ivet F. Biogas production from microalgae grown in wastewater: effect of microwave pretreatment. Appl Energy. 2013;108:168–170.
- Palmowski L, Simons L, Brooks R. Ultrasonic treatment to improve anaerobic digestibility of dairy waste streams. Water Sci Technol. 2006;53:281–288.
- Park KY, Lee JW, Ahn KH, Maeng SK, Hwang JH, Song KG. Ozone disintegration of excess biomass and application to nitrogen removal. Water Environ Res. 2004;76:162–167.
- Kim S, Holtzapple MT. Lime pretreatment and enzymatic hydrolysis of corn stover. Bioresour Technol. 2005;96(18):1994–2006.
- Bjerre AB, Olesen AB, Fernqvist T, Ploger A, Schmidt AS. Pre-treatment of wheat straw using combined wet oxidation and alkaline hydrolysis resulting in convertible cellulose and hemicellulose. Biotechnol Bioeng. 1996;49:568–577.
- Aiba S, Fan LT, Fiechter A, Klein J, Schügerl K, editors. Biotechnology monographs, vol. 3, cellulose hydrolysis. Berlin: Springer-Verlag; 1987.
- Lin JG, Chang CN, Chang SC. Enhancement of anaerobic digestion of waste activated sludge by alkaline solubilization. Bioresource Technol. 1997;62:85–90.
- Lopez TM, Espinosa LMC. Effect of alkaline pretreatment on anaerobic digestion of solid wastes. Waste Manag. 2008;28:2229–2234.
- Millett MA, Baker AJ, Satter LD. Physical and chemical pretreatments for enhancing cellulose saccharification. Biotechnol Bioeng Symp. 1976;6:125–153.
- Zhu J, Wan C, Li Y. Enhanced solid-state anaerobic digestion of corn stover by alkaline pretreatment. Bioresour Technol. 2010;101(19):7023–7028.
- Stamatelatou K, Antonolopoulou G, Ntaikou I, Lyberatos G. The effect of physical, chemical, and biological pretreatments of biomass on its anaerobic digestibility and biogas production. In: Mudhoo A, editor. Biogass production: pretreatment methods in anaerobic digestion, Biogas production. Hoboken (NJ): Scrivener Publishing LLC; 2012. p. 50–99.
- Yang D, Zheng Y, Zhang R, An ASABE International Meeting; Jun 21–24; Reno (NV): American Society of Agricultural and Biological Engineers; 2009. (Paper No: 095685).
- Bougrier C, Delgenès JP, Carrère H. Effects of thermal treatments on five different waste activated sludge samples solubilisation, physical properties and anaerobic digestion. Chem Eng J. 2008;139(2):236–244.
- Skiadas IV, Gavala HN, Lu J, Ahring BK. Thermal pre-treatment of primary and secondary sludge at 70 °C prior to anaerobic digestion. Water Sci Technol. 2005;52(1–2):161–166.
- American Public Health Association. Standard methods for water and wastewater examination. Washington (DC): American Public Health Association; 1998.