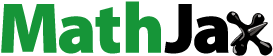
Abstract
Diosgenin is a valuable precursor for the synthesis of steroid compounds and is widely used in the pharmaceutical industry. The production of diosgenin from plant tubers by using microbial transformation is a cheap and environmentally–friendly method. However, the low efficiency of the existing fermentation protocols prevents the large–scale production required to meet the industrial demand. In this work, a solid state fermentation of Dioscorea zingiberensis was performed by using a mixed culture of Trichoderma reesei and Aspergillus fumigatus to increase the bioconversion efficiency. We optimized several parameters of the fermentation process of T. reesei and A. fumigatus monocultures using a response surface methodology. Next, we tested fermentation strategies and different ratios of T. reesei:A. fumigatus (T:A) inoculum. A maximum yield (95.82%) resulted from using a T:A ratio of 1:2 (7.5 × 106 T. reesei spores/g pretreated DZW) and a fermentation strategy, in which T. reesei was added at the start of the fermentation and A. fumigatus was added after 24 hours.
Introduction
Diosgenin (CAS number 512-04-09), an important plant steroidal sapogenin, is widely used as a precursor for partial synthesis of androstene, cortisone, androstane, progesterone and other steroids in the pharmaceutical industry.[Citation1,Citation2] The production of diosgenin shows a great market potential. More than 3000 tons of diosgenin are produced worldwide per year.
In China, Dioscorea zingiberensis (DZW) is the main plant used to produce diosgenin due to the high content of saponins in its tubers.[Citation3] Traditionally, acid hydrolysis of DZW tubers has been used to release diosgenin from saponins. However, this method poses significant environmental consequences by producing acid wastewater with a high level of chemical oxygen demand (COD; >60,000 mg/L) and total nitrogen (>650 mg/L).[Citation4,Citation5] Compared to acid hydrolysis, the microbial transformation is an environmentally-friendly method because of the mild reaction conditions, which are required.[Citation6] However, microbial transformation techniques have not yet been utilized in a large-scale production due to the low yield of diosgenin and the necessity for submerged fermentation (SmF), which is a high energy requiring process that offers a low production yield.
In our previous researches, we used Trichoderma reesei to convert saponins from DZW into diosgenin.[Citation7–9] We found that about 90% of the diosgenin was released from saponins and the main intermediate was diosgenin-glucoside (trillin).[Citation10] We also found that another fungus, Aspergillus fumigatus, which yields a high level of special β-glucosidase, is able to transform diosgenin-glucoside effectively.[Citation11] Thus, we wanted to test if co-culturing of T. reesei and A. fumigatus could increase the efficiency of microbial transformation.
Solid state fermentation (SSF) has many practical advantages over the SmF, such as lower capital cost, lower energy requirements, less water usage and wastewater generation and a higher concentration of the product.[Citation12] Compared with SmF, SSF provides a better opportunity for a large-scale production. In general, fungal strains are the preferred microorganisms for SSF due to their low water activity requirements.[Citation13]
To test the large-scale production of diosgenin by using a mixed culture SSF with T. reesei and A. fumigatus, we first optimized the pure strain SSF conditions by using a response surface methodology. Second, we optimized the parameters for mixed culture SSF for a large-scale operation.
Materials and methods
Materials
DZW tubers were obtained from Yunxi City, Hubei province, China. Cellulase (10,000 IU/g) and α-amylase (2,000 IU/g) were supplied by Shandong Longda Bio-product Co., Ltd., Shangdong, China, DZW was pretreated with the method that we demonstrated previously [Citation7] and therefore pretreated DZW (PDZW) was obtained. p-Nitrophenyl-β-D-glucopyranoside (pNPG), p-nitrophenyl-α-L-rhamnopyranoside (pNPR) and the standard of diosgenin were procured from Sigma Company, Shanghai, China. The standard of diosgenin-3-O-[β-D-glucopyranosyl(1→4)]-α-L-rhamnopynosyl (diosgenin-glucoside-rhamnoaside) was purchased from Nantong Kanmaike Co., Ltd (Jiangsu, China). The standard of diosgenin-3-O-[β-D-glucopyranosyl(1→4)]-β-D-glucopyranside (diosgenin-diglucoside) and the standard of trillin (diosgenin-glucoside) were purchased from Zhongkangweiye Co., Ltd (Shanghai, China).
Microorganisms
T. reesei (ACCC 30597) was obtained from the Agricultural Culture Collection of China (Beijing, China). A. fumigatus was isolated from naturally fermented DZW tubers in our laboratory and identified by Sichuan Microbiological Resources Infrastructure & Culture Collection Center, China.[Citation11]
Solid state fermentation in a flask
The experiment was conducted in 50 mL Erlenmyer flasks. One gram of PDZW in each flask was adjusted to different moisture contents with a medium containing 2.67% peptone and 0.27% K2HPO4, w/v, pH 5.8. The contents were mixed thoroughly and were sterilized at 121 °C for 30 min. Each flask was inoculated with 1 mL of T. reesei or A. fumigatus spore suspension, containing 107 spores. The flasks were incubated in a water bath shaker, at a shaking speed of 150 rpm for 168 hours. Every 12 hours, one set of flasks was assayed to determine the concentration of diosgenin in the microbial transformation products.
Optimization of the pure strain fermentation conditions was carried out using the response surface methodology (RSM). A three-factor and five-level central composite design, consisting of 20 experimental trials was employed. The design contained three independent variables -- Tween 80 (x1), temperature (x2) and moisture content (x3). The response variable was diosgenin yield (%), resulting from the T. reesei (y1) and A. fumigatus (y2) biotransformation.
The independent variables were coded as −1.68, −1, 0, 1 and 1.68 (). The experimental data from 20 runs were analysed with RSM algorithm of Design Expert 8.0.7.1 and fitted to a second-order polynomial (EquationEquation (1)(1)
(1) ):
(1)
(1)
Table 1. Central composite design (CCD) matrix for Tween 80, temperature and moisture content and the experimental responses.
In this equation, y is the predicted diosgenin yield, β0 is the offset term, xi is the coded value of independent variable, and βi, βii and βij are the linear, quadratic and interaction coefficients, respectively.
Analysis of variance (ANOVA) was conducted using the Analysis package of Design Expert 7.1.8.0. The optimization of the three independent variables for a maximum diosgenin yield was carried out using a numerical optimization package of Design Expert 7.1.8.0. The values of the independent variables were optimized with a maximization of both y1 and y2.
The optimized result, obtained from RSM, was used for the production of diosgenin from mixed cultures of T. reesei and A. fumigatus. Before the optimization of the mixed culture fermentation conditions, the changes in saponin concentrations and enzyme activities in the pure culture transformation process were determined. Based on these results, a mixed culture fermentation was conducted. The ratio of T. reesei:A. fumigatus (T:A), inoculum level and fermentation strategies were optimized using one-factor-at-a-time method.
α-Rhamnase and β-glucosidase activities
The total fermentation broth in one flask was mixed with 4 mL water and centrifuged at 4 °C and 10,000 rpm for 15 min. The residue was suspended in 3 mL water and centrifuged again. The supernatant was combined and adjusted with distilled water to 10 mL. α-Rhamnase or β-glucosidase activity in the solution was determined using pNPR or pNPG as substrate with the method that we have described previously.[Citation7] One unit (IU) of α-rhamnase (or β-glucosidase) activity was defined as the amount of enzyme releasing 1 μmol of p-nitrophenol in 1 min.
Results and discussion
Optimization of pure culture solid state fermentation
Optimization of SSF parameters (Tween 80, temperature and moisture content) to maximize the diosgenin production in pure culture fermentation was carried out using RSM. The results are shown in .
For T. reesei, the overall second order polynomial equation for diosgenin was
(2)
(2) where y1 is the predicted diosgenin yield obtained by T. reesei SSF, and x1, x2 and x3 are code values of Tween 80, temperature and moisture content, respectively.
The calculated R2 (0.9727) indicated a high agreement between the measured and the predicted results. ANOVA implied that the quadratic model was statistically valid with an F value of 39.62 (P < 0.001) and a lack of fit value of 2.85 (P > 0.05) (). The regression results of EquationEquation (2)(2)
(2) showed that the linear terms of x3 and quadratic terms of x1 and x3 demonstrated the most significant impact on diosgenin production (P < 0.001). While the linear terms of x1 and cross products of x2x3 showed significant effects on diosgenin yield (P < 0.05), the influences of the other terms were insignificant (P > 0.05).
Table 2. The fit statistics of the two models.
For A. fumigatus, applying a multiple regression analysis on the coded experimental data, a polynomial equation for diosgenin yield (y2) was as follows:
(3)
(3) where y2 is the predicted diosgenin yield, obtained from A. fumigatus SSF, and x1, x2 and x3 are code values of Tween 80, temperature and moisture content, respectively.
ANOVA indicated that the regression model that we proposed was statistically good with a R2 value of 0.9415. The F value of 17.89 (P < 0.001) and lack of fit value of 2.41 (P > 0.05) also implied the fitness of model. The coefficient values of the terms in EquationEquation (3)(3)
(3) were evaluated and are demonstrated in . The P values of x1, x3 and
were lower than 0.001, indicating that they were the most significant factors influencing the diosgenin production. x1x2 and
were less significant factors with P value < 0.05.
The results showed that Tween 80 and moisture content influenced the biotransformation efficiency of both T. reesei and A. fumigatus significantly.
Although determination of the conditions required for a maximum diosgenin yield from pure cultures was informative, we predicted that intermediate culturing conditions would be required to maximize the production in mixed cultures. Thus, using Design Expert 7.1.8.0 numerical optimization design space, the optimal conditions were determined with the setting of ‘maximum of both y1 and y2.’ We selected these conditions to be 1.3% Tween 80, 30 °C and 75% moisture content, as operational parameters for the production of diosgenin from mixed cultures. Under these conditions, y1 of 82.01% and y2 of 71.48% were obtained.
Optimization of mixed culture solid state fermentation
Three condition parameters -- the ratio of T. reesei:A. fumigatus (T:A); the inoculum level and the fermentation strategies were optimized for the production of diosgenin in mixed culture SSF.
The composition of the mixed culture may influence the efficiency of the microbial transformation because of changes in the proportions of the enzymes produced by the two species.[Citation14,Citation15] In this research, five ratios of T. reesei and A. fumigatus (1:0.25, 1:0.5, 1:1, 1:2, 1:4) were studied (). We found that an inoculum ratio of 1:2, after 156 hour of incubation, resulted in a maximum diosgenin yield of 93.66%. The diosgenin yield decreased at 1:0.25, 1:0.5 and 1:1 ratios, with values of 86.92%, 88.35% and 91.54%. The production of diosgenin at 1:4 ratio was similar to that at 1:2 ratio. So, the ratio of 1:2 was chosen for the co-culturing conditions.
Figure 1. Effects of T:A ratio (a), inoculum level (b) and fermentation strategies (c) on diosgenin yield.
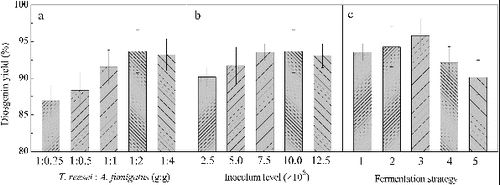
From the prospective of enzyme production, the inoculum level of the co-culture is a critical factor for determining the microbial transformation efficiency.[Citation16] The inoculum level can be optimized to maximize the diosgenin yield and to reduce the operation cost. The biotransformation efficiency was investigated by using an inoculation level of 2.5–12.5 × 106 T. reesei spores/g PDZW. The results are shown in (b). When the inoculum level was increased from 2.5 × 106 to 7.5 × 106, the maximum diosgenin yield increased from 90.17% to 93.57%. When the inoculum level was increased from 7.5 × 106 to 12.5 × 106, the diosgenin yield did not improve, therefore the suitable inoculation level under these mixed culture conditions was 7.5 × 106 T. reesei spores/g PDZW and 1.5 × 107 A. fumigatus spores/g PDZW.
In the mixed culture fermentation process, the feeding strategy may influence on the efficiency of biotransformation.[Citation17] In the present work, five culture strategies were investigated. Because T. reesei is a good producer of glucosidases, which are essential to convert saponins to diosgenin-glucoside and A. fumigatus allows an effective conversion of diosgenin-glucoside to diosgenin, we reasoned that it might make sense to add T. reesei first and then add the A. fumigatus. To test the effect of adding A. fumigatus to the cultures at different times, we added T. reesei at the start of the SSF and A. fumigatus was added at the same time (strategy 1), after 12 hours (strategy 2), after 24 hours (strategy 3), after 36 hours (strategy 4) or after 48 hours (strategy 5). The results are presented in (c). The highest transformation efficiency was achieved during strategy 3, with a diosgenin yield of 95.82%.
Based on the above analysis, conditions with T:A ratio of 1:2, an inoculation level of 7.5 × 106 T. reesei spores/g PDZW and fermentation strategy 3 were selected for the mixed culture SSF. The results of the enzymes production and saponins changes as a function of time in mixed culture are presented in . As shown in the figure, β-glucosidase production was enhanced by the mixed culture. The enzyme activity reached a maximum value of 92.7 IU/g PDZW on day 4.5, which was significantly higher than that of the monocultures, which was 81.9 IU/g PDZW for T. reesei and 77.3 IU/g PDZW for A. fumigatus. The α-rhamnase from the mixed culture was similar to that from T. reesei, but the enzyme was more stable in the mixed culture than in T. reesei's culture. The highest diosgenin concentration of 68.21 μmol/g was obtained on the sixth day in the mixed culture, which was 15.69% higher than that in T. reesei. On the sixth day, the concentrations of diosgenin-diglucoside, diosgenin-glucoside-rhamnoside and diosgenin-gluoside in the mixed culture were 0 μmol/g, 2.04 μmol/g and 1.16 μmol/g, respectively, which were significantly lower than those in the monocultures. Lower concentrations of those compounds in the residue mean that more of them were transformed into diosgenin and therefore the diosgenin yield was increased.
Figure 2. Profiles of α-rhamnase (○) and β-glucosidase (■) activities obtained from T. reesei (a), A. fumigatus (b) and mixed culture (c) SSF. Profiles of concentrations of diosgenin-diglucoside (□), diosgenin-glucoside-rhamnoside (•), diosgenin-glucoside (△) and diosgenin (▾) obtained from T. reesei (d), A. fumigatus (e) and mixed culture (f) SSF.
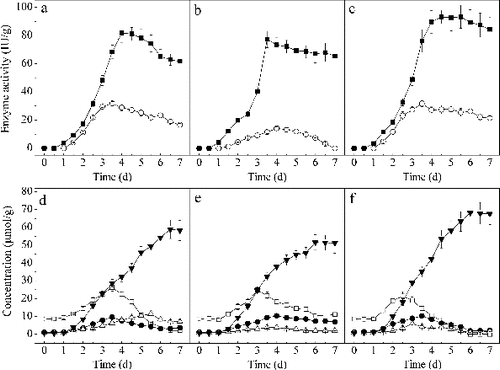
This may be due to a synergism of the two fungi. A complete enzyme complex was produced in the mixed culture system.[Citation18,Citation19] Saponins in PDZW were transformed into diosgenin-glucoside with α-rhamnase and β-glucosidase produced by T. reesei.[Citation10] Diosgenin-glucoside was then transformed into diosgenin with β-glucosidase from A. fumigatus.[Citation11] Those may be the reasons why diosgenin yield was increased by the mixed culture of T. reesei and A. fumigatus.
Conclusions
In the present study we demonstrated the suitability of a mixed culture SSF in the production of diosgenin from DZW. With RSM, the single strain SSF conditions were first optimized. Tween 80 of 1.3%, 30 °C and 75% moisture content were chosen for operational parameters of the mixed culture SSF. The conditions of co-culture SSF were then optimized. The highest diosgenin yield of 95.82% was obtained using mixed culture fermentation at 1:2 ratio, inoculum level of 7.5 × 106 T. reesei spores/g PDZW and fermentation strategy 3.
Disclosure statement
No potential conflict of interest was reported by the authors.
Additional information
Funding
References
- Lepage C, Léger DY, Bertrand J, Martin F, Beneytout JL, Liagre B. Diosgenin induces death receptor-5 through activation of p38 pathway and promotes TRAIL-induced apoptosis in colon cancer cells. Cancer Lett. 2011;301:193–202.
- Mu SM, Tian XS, Ruan YM, Liu YT, Bian DZ, Ma CY, Yu CX, Feng M, Wang FR, Gao L, Zhao JJ. Diosgenin induces apoptosis in IGF-1-stimulated human thyrocytes through two caspase-dependent pathways. Biochem Biophys Res Commun. 2012;418:347–352.
- Huang W, Zhao HZ, Ni JR, Zuo H, Qiu LL, Li H. The best utilization of D. zingiberensis C. H. Wright by an eco-friendly process. Bioresour Technol. 2008;99:7407–7411.
- Zhao HZ, Cheng P, Zhao B, Ni JR. Yellow ginger processing wastewater treatment by a hybrid biological process. Process Biochem. 2008;43:1427–1431.
- Cheng P, Zhao HZ, Zhao B, Ni JR. Pilot treatment of wastewater from Dioscorea zingiberensis C.H. Wright production by anaerobic digestion combined with a biological aerated filter. Bioresour Technol. 2009;100:2918–2925.
- Fernandes P, Cruz A, Angelova B, Pinheiro HM, Cabral JMS. Microbial conversion of steroid compounds: recent developments. Enzyme Microb Technol. 2003;32:688–705.
- Zhu YL, Huang W, Ni JR, Liu W, Li H. Production of diosgenin from Dioscorea zingiberensis tubers through enzymatic saccharification and microbial transformation. Appl Microbiol Biotecnol. 2010;85:1409–1416.
- Zhu YL, Huang W, Ni JR. A promising clean process for production of diosgenin from Dioscorea zingiberensis C. H. Wright. J Clean Prod. 2010;18:242–247.
- Zheng TX, Yu LD, Zhu YL, Zhao B. Evaluation of different pretreatments on microbial transformation of saponins in Dioscorea zingiberensis for diosgenin production. Biotechnol Biotechnol Equip. 2014;28(4):740–746.
- Zhu YL, Zhu HC, Qiu MQ, Zhu TT, Ni JR. Investigation on the mechanisms for biotransformation of saponins to diosgenin. World J Microbiol Biotechnol. 2014;30:143–152.
- Lei J, Niu H, Li TH, Huang W. A novel β-glucosidase from Aspergillus fumigates releases diosgenin from spirostanosides of Dioscorea zingiberensis C. H. Wright (DZW). World J Microbiol Biotechnol. 2012;28(3):1309–1314.
- Pandey A. Solid-state fermentation. Biochem Eng J. 2003;13:81–84
- Singhania RR, Patel AK, Soccol CR, Pandey A. Recent advances in solid-state fermentation. Biochem Eng J. 2009;44:13–18.
- Kedia G, Wang RH, Patel H, Pandiella SS. Use of mixed cultures for the fermentation of cereal-base substrates with potential probiotic properties. Process Biochem. 2007;42:65–70.
- Viana F, Gil JV, Vallés S, Manzanares P. Increasing the levels of 2-phenylethyl acetate in wine through the use of a mixed culture of Hanseniaspora osmophila and Saccharomyces cerevisiae. Int J Food Microbiol. 2009;135:68–74.
- Ahamed A, Vermette P. Enhanced enzyme production from mixed cultures of Trichoderma reesei RUT-C30 and Aspergillus niger LMA as fed batch in a stirred tank bioreactor. Biochem Eng J. 2008;42:41–46.
- Fang H, Zhao C, Song XY. Optimization of enzymatic hydrolysis of steam-exploded corn stover by two approaches: response surface methodology or using cellulase from mixed cultures of Trichoderma reesei RUT-C30 and Aspergillus niger NL02. Bioresour Technol. 2010;101:4111–4119.
- Ahamed A, Vermette P. Culture-based strategies to enhance cellulase enzyme production from Trichoderma reesei RUT-C30 in bioreactor culture conditions. Biochem Eng J. 2008;40:399–407.
- Dhillon GS, Oberoi HS, Kaur S, Bansal S, Brar SK. Value-addition of agricultural wastes for augmented cellulase and xylanase production through solid-state tray fermentation employing mixed-culture of fungi. Ind Crops Prod. 2011;34:1160–1167.