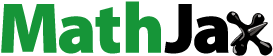
Abstract
Today, environmental protection is one of the main goals in the strive to preserve the human existence. Development in this area requires invention of new materials, which can reduce the levels of pollution. Hybrid materials are suitable for this purpose, because they combine different desirable properties existing in separate sources into one unique and accessible structure. Most of the commonly used materials for the degradation of different kind of pollutants are based on titanium dioxide, because of its photocatalytic activity under UV irradiation. Innovative silica hybrid materials, containing an organic component (chitosan) and titanium nanoparticles, were successfully synthesized via the sol–gel method and tetraethyl orthosilicate was used as a silica source and network former. Interaction between the chitosan and titanium units, and their influence on the structure of final material, were observed and discussed. A homogeneous structure with an even distribution of titanium and chitosan particles was visible from scanning electron microscopy (SEM) micrographs and the particle size varied between 50 and 150 nm. The formed silica network, characteristic peaks of chitosan and titanium groups and possible interactions between them are observed from Fourier transform infrared (FTIR) spectroscopy spectra and nuclear magnetic resonance (NMR) spectroscopy results. The behaviour of the synthesized silica hybrids after thermal treatment was investigated via differential thermal/thermo-gravimetric analysis (DTA/TG) analysis and the sorption and degradation activities of the obtained hybrid materials were investigated using a solution of methyl orange as model pollutant. The structure and properties of the synthesized silica hybrid materials assert their potential application in environmental remediation due to their photocatalytic degradation and sorption activities against pollutants.
Introduction
Environmental pollution problems are intensively discussed these days. Wastewater pollutants cause the biggest damages on natural environment. Water pollutants (organic components, heavy metals and other) are toxic, hazardous to humans, stable and resistant in different media materials. The deactivation process of these pollutants needs to be enforced before they damage the environment and adsorption is an efficient technique, which can be used to this cause. Reduction and prevention of further distribution of pollutants require an improvement on existing sorbents or invention of new ones.[Citation1]
Traditional purification materials based on ceramic membranes (for example, zeolites) or activated carbon exhibit high sorption activities, but limitations due to pollutant variety, purifying and reuse effects have led to the invention of innovative structures and hybrid materials with higher sorption activity and stability and these are highly sought. Preparation of hybrid materials allows for the variation of used components, final structure and properties with respect to the intended application.[Citation2] It is necessary to obtain a sorbent that exhibits sorption and deactivation activity against more than one pollutant.[Citation3] In this case, the type of preparation, nature of initial components and characteristics of the final hybrid structures are determining factors in the decision to utilize a material or synthesis method.
Sol–gel synthesis is one of the easiest and most useful techniques for the preparation of hybrid materials for reducing the environmental pollution, as it allows for the control of the process steps, the variation of the quality and quantity of the used components and their physical properties (humidity, temperature, pH, pressure and others).[Citation4]
Hybrid materials to be utilized in environmental pollution control are developed to possess improved qualities. One of these developments is the synthesis of a material, containing two highly reactive components, which exhibit sorption and photocatalytic activities.[Citation5] Chitosan is proven to be a highly reactive adsorbent, which can remove heavy metal ions, dye and organic pollutants from wastewater with high efficiency.[Citation6] This natural polymer is produced from marine shrimps, containing amino/amide and hydroxyl-free reactive groups. In acidic conditions, chitosan is transformed into a cationic polymer, which can easily interact with an anionic pollutant.[Citation7] Chitosan is characterized with hydrophilic, non-toxic, biocompatible and biodegradable properties. Solubility and specific behaviour in different media reduces the potential application of pure chitosan.[Citation8] In the past decades, photodegradation of organic pollutants was an extensively applied technique. Some oxides, which exhibit photocatalytic activities are ZnO, TiO2 and SnO2, and investigations proved that TiO2 exhibits excellent degradation activity against organic pollutants under UV radiation.[Citation9,Citation10] Photocatalytic activity is associated with formation of electrons and holes. In contact with water or air, the electron/hole pairs lead to formation of hydroxyl (–OH), superoxide O2− and hydroperoxyl (–OOH) radicals,[Citation11] which degrade organic pollutants. Application of pure TiO2 units for environmental purification is reduced, because of the quantity of the used material, as well as further recombination.[Citation12]
Combination between TiO2 and chitosan leads to the formation of an adsorption–photocatalytic hybrid with improved capacities. Zubieta et al. [Citation13] tested the behaviour between these two components and found that chitosan can enhance the adsorption process due to charge interactions with pollutants. On the other hand, when the free reactive centres are blocked, the pollutants can be photodegraded by TiO2 units under UV irradiation.[Citation14,Citation15] Incorporation of these two components into a suitable carrier allows the formation of a useful hybrid with improved sorption activity and stability [Citation16] and it has been proven that the suitable carriers of hybrid structures are silica materials.[Citation17] High mechanical, chemical and thermal stabilities of silica networks ensure the long-term stability of the final hybrid.[Citation18]
There have been a lot of studies focused on environmental remediation using silica–chitosan, silica–TiO2 and TiO2–chitosan hybrid materials as adsorbents.[Citation19–23] Preparation of hybrid materials, which combine silica, TiO2 and chitosan components into one structure are less investigated. By using sol–gel technique, it is possible to obtain hybrid materials with high sorption activities. In the process of synthesis, stable silica networks with evenly distributed TiO2 and chitosan were formed. It is also proven that, silica components can form strong bonds with chitosan and can enhance the photocatalytic activity of TiO2 even after the removal of the UV source.[Citation24,Citation25] The novel hybrid materials can be successfully applied as adsorbents of pollutants in wastewater.
The aim of the present study was sol–gel synthesis of innovative silica hybrid materials, by using chitosan and titanium moieties. Compatibility between the used components and their influence on the final hybrids were observed and discussed. The structural characteristics and properties of the obtained materials were investigated using X-ray diffraction (XRD), Fourier transform infrared (FTIR) spectroscopy, scanning electron microscopy (SEM), nuclear magnetic resonance (NMR) spectroscopy and differential thermal/thermo-gravimetric analysis (DTA/TG) analysis. Sorption and degradation activities were evaluated using a solution of methyl orange (MO) as a model of organic pollutant and UV radiation. Reuse of the synthesized hybrid materials for recombination and observation of the previously exhibited properties was done.
Materials and methods
The following components were used for the sol–gel synthesis of the silica hybrid materials: tetraethyl orthosilicate (TEOS, 98% Sigma Aldrich), titanium n-butoxide (TBOT, 99% Alfa Aesar), chitosan (CS, LW, Fluka), acetic acid, absolute ethanol (Alkaloid Skopje), distilled water (dH2O), HCl (37%, Merck) and methyl orange indicator.
Synthesis method
The first step in the preparation of the silica hybrid material was the fabrication of the silica carrier, and for that purpose TEOS was mixed with dH2O and 1 mol L−1 HCl in the ratio 1:0.4:0.4, respectively, and the resultant solution was vigorously stirred for an hour. The solution's composition was kept constant for all the synthesized hybrids. Chitosan (1 g L−1) was also separately dissolved in 1 mol L−1 acetic acid. TBOT rate of hydrolysis is faster than that of the silica precursor. For preparation of homogeneous mixture and hydrolysis of Ti–O–C groups, the titanium precursor was initially dissolved in ethanol (TBOT/ethanol; 1:1 ratio). After hydrolysis of TEOS, a calculated amount of CS and TBOT was added to the obtained silica sol. To determine the influence of the quantities of CS and TBOT components to the structure and properties of the final hybrids, the component ratio varied – TEOS/CS/TBOT = 1:0.1:0.1 (SiCSTi1); 1:0.2:0.2 (SiCSTi2); 1:0.3:0.3 (SiCSTi3); 1:0.4:0.4 (SiCSTi4); 1:0.5:0.5 (SiCSTi5).
Structural characterization
The following analytical methods were used to evaluate the structural characteristics of the synthesized hybrid materials: XRD (Brucker D8 Advance, X-ray diffractometer, Cu-Kα, in the range 10°–80° 2θ with scan rate 3° 2θ min−1), FTIR (Mattson 7000, Brucker Tensor 27 Spectrometer, 4000–400 cm−1), SEM (Hitachi SU-70), NMR (Bruker MSL-400 NMR spectromet), DTA/TG (Setaram LabsysTm TG-DSC16, temperature range 0 °С–1000 °С, heating rate 10 °С min−1).
Sorption and degradation experiments
The sorption and degradation activities of the synthesized silica hybrid materials were investigated via batch method using water solution of MO as an organic pollutant. One gram of the synthesized sample was immersed into 100 mL MO solution with concentration of 80 mg L−1 in a beaker. An ultraviolet lamp (8 W, wavelength 368) was used as the UV source. The samples were irradiated for 4 h and the distance between the UV lamp and the beaker was 10 cm. The materials were reused in the same conditions for evidence of properties recombination. The change of MO concentration for each test was measured by ultraviolet–visible (UV–Vis) spectrophotometer.
The percentage of removal (%R) of MO is defined as
(1)
(1) where Co and Ce are the liquid phase initial and equilibrium dye concentrations (mg L−1), respectively.[Citation26]
Results and discussion
XRD results
Investigations on the structure of the synthesized hybrid materials were done using XRD analysis and the obtained results are shown in . Halos around 23° and 12° were presented on XRD patterns.
The amorphous halo around 23° showed that the synthesized silica hybrid materials are in an amorphous state. The formed amorphous structures are results of formed Si–O–Si network, obtained via sol–gel synthesis at room temperature. Song et al. [Citation27] established that chitosan has three forms – noncrystalline (11.7°), hydrated crystalline (14.2°) and anhydrous crystalline (23°). The presence of a peak around 12 2θ showed that the CS additive in the hybrid materials is in amorphous state. The peak proved the compatibility between the used acetic acid and the polymer. On the other hand, peak associated with anhydrous crystalline is overlapped with silica network peak. In comparison with XRD pattern of pure silica material (not shown), there was no increased intensity around 23° and this proved that CS in the obtained hybrids was in an amorphous state. The absence of other intensive peaks can be associated with good distribution of TBOT component without formation of aggregates.
FTIR spectra
Characteristic groups of used components, as well as potential interactions between them, are investigated via FTIR analysis and the results are presented in .
As a result of hydrolysis and polycondensation reactions, TEOS formed two specific bonds, Si–O–Si and Si–OH. The symmetric vibrations of Si–O–Si bonds are associated with the presence of peaks at 450 cm−1, 560 cm−1 and 790 cm−1. Asymmetric vibrations of silica network is ascribed with an intensive peak around 1080 cm−1. The presence of Si–OH bonds are due to partial polycondensation of TEOS and are associated with peaks at 950 cm−1. The new materials’ synthesis and drying at room temperature produce residual or adsorbed water in the structures. The peaks at 1640 cm−1 and 3400 cm−1 showed the presence of H–OH groups.
The existence of chitosan component can be established with the presence of peaks, corresponding to carbon structural units, N–H and –OH groups. The polymer structural units, as well as the oxygen bridge between them, are presented via peaks in the range of 1100–1200 cm−1. C–H bonds are attributed with peak around 2300 cm−1. Small intensive peaks in the range of 1400–1500 cm−1 ascribed the existence of N–H groups in the hybrid structures. The peak of chitosan –OH groups is overlapped with the peak of water bonds at 1640 cm−1.[Citation28]
The peaks at 560 cm−1 and around 1450 cm−1 can be associated with the presence of Ti–O–Ti bonds. Potential interactions between silica and titanium sources and the formation of Si–O–Ti bonds can be associated with the peak at 950 cm−1.[Citation29] On the other hand, small intensive peaks connected with free reactive groups of chitosan can be attributed to a possible interaction between the polymer and other components.[Citation3] FTIR spectra of synthesized hybrid materials showed that the obtained structures are characterized with stable silica network with existig chitosan and TiO2 components.
SEM micrographs
The surface and microstructure characteristics of the obtained hybrid materials are determined for their potential application in the field of environmental remediation. SEM micrographs of synthesized hybrids are shown in . A smooth, homogeneous surface is observed on the surface micrographs for all the samples. This serves as proof of the suitability of the synthesis technique and the compatibility of the used components. The formation of nanoparticles is clearly visible on the volume micrographs with their sizes varying between 50 and 150 nm.
Figure 3. SEM micrographs on the surface (left) and in the volume (right) of the obtained hybrid structures.
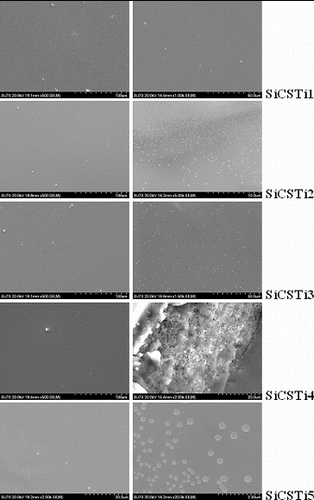
The homogeneous and smooth structures are as a result of sol–gel synthesis. The prepared silica network via two reaction processes and proper distribution of CS and TBOT components formed stable and resistant materials. The SEM proved that synthesized hybrid materials are suitable for environmental use due to their smooth surface formation, ensuring the material's resistance to aggressive agents. Furthermore, establishment of nanoparticles all over the hybrid structure proved that these materials contain reactive centres capable of interacting and degrading the pollutants.
NMR results
The silica network obtained via sol–gel reactions of TEOS is responsible for the property of newly synthesized materials and consequently their application for environmental protection. 29Si NMR analysis was used to establish the construction of the silica matrix and the degree of polymerization of SiO4 tetrahedral ions in the network ().
The presence of resonance peaks Q4 and Q3 are visible from the results. The peak Q4 (∼110 ppm) denotes that every SiO4 polyhedral is connected by an oxygen bridge to four other silica polyhedral. The intensity of the peak also decreases with increasing CS/TBOT value. Next to this peak is another assigned as Q3 which showed that some of the SiO4 polyhedral has three bridging oxygen bonds with other silica polyhedral and one with the other components. The presence of Q3 peak can be attributed to possible interactions between silica and other components and the formation of Si–O–C and Si–O–Ti bonds. From the results, a conclusion can be drawn that the intensity of the peak ∼101 ppm (Q3) increases with an increase in the Si/TBOT values.[Citation30]
Based on the investigations of the structure of synthesized hybrid materials and the literature's review, the scheme of potential interactions between the used components is presented in .[Citation31]
DTA/TG analysis
DTA/TG results of the synthesized silica hybrid materials after heating are shown in and one endothermic reaction in the region 100 °C–200 °C is observed from the DTA analysis. Structure transformations of the synthesized hybrid materials around 150 °C can be connected with the evaporation of residual/adsorbed water, ethanol and organic groups. By increasing the temperature to 800 °C, possible reactions, such as the chitosan degradation and polymerization of titanium and silica units, may occur.[Citation32] The preparation of crystal phase TiO2 from TBOT via sol–gel synthesis showed that, in the temperature range 350 °C–1000 °C, it is possible to obtain anatase or rutile phases.[Citation33]
The heating of the obtained silica hybrid material showed that at 1000 °C, the material experienced a 25% loss in mass. The reduction in mass is due to endothermic reaction at around 150 °C. Fifteen percent weight loss proved the evaporation of water, solvents and part of the organic residual groups. The further 10% weight loss with increasing temperature can be associated with polymerization of inorganic parts, and degradation of chitosan units. The DTA/TG results showed that the synthesized hybrid materials are thermally stable due to one intensive transformation and the 25% weight loss. Observation of the individual components during the heating showed that their stability increased. This observation proves the compatibility of the used initial materials, their proper synthesis and improved property of the final hybrids.
Sorption and degradation of MO
Test of sorption and degradation activities of synthesized hybrid materials were made using MO solution. UV irradiation was employed for 4 h to activate the sorption and photocatalytic activities of the hybrids (). The UV–Vis analysis of the test solution shows two absorption peaks around 270 and 470 nm. The intensity of these two peaks reduced after the addition of a calculated amount of synthesized hybrids and UV irradiation ((a)). This change can be associated with the adsorption or degradation of MO molecules on the hybrid surface. The change of the wavelength after 500 nm presented the favourable effect of the hybrid structure. The UV–Vis results of the reused materials, using the same initial MO concentration and irradiation time, showed that their absorption capacity and wavelength remained unchanged when compared to the first step of the experiment ((b)). MO is dissolved in water with dissociation of SO3Na and MO is converted into anionic dye ions. The exact concentration of MO is 79.2% and the reduction of MO after UV irradiation was established. The tendency of reduction follows the increasing step of chitosan and TiO2 additives in the structure. The presence of chitosan in the obtained structures (on the surface) allowed contact between free hydroxyl and amino/amide groups of chitosan and organic dye. From UV–Vis analysis, it was established that the pH of initial MO solution was 5 and after treatment with hybrid materials and UV irradiation the pH became reduced (pH = 3–5). The acidic media favour conversion of NH2 groups into NH3+, which accelerated the attraction of MO units. The interaction between amino groups of chitosan and MO can be denoted with the following reaction:
Figure 7. UV–Vis analysis of absorption capacity of synthesized hybrid materials after 4 h of UV irradiation (a) and reuse test (b).
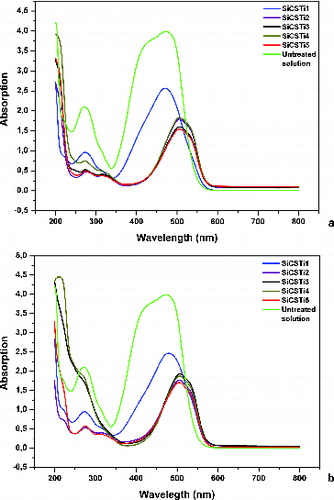
The mechanism of sorption and degradation activities of TiO2 is activated due to the presence of UV light and water molecules. The photocatalytic process is activated as a result of transformation of light into chemical energy. As a result of light activation of TiO2 particles, the oxygen ion of water molecules is transforming in a anionic reactive center, which can easily interact with cationic dye pollutant and degrade it. The results of the calculated equilibrium adsorption concentration of MO showed that with increasing the content of CS/Ti components, the activity of materials increased after 4 h of irradiation.
The free reactive groups, as well as potential contact mechanism between MO particles and hybrid structures, are represented in .
The removed amount of MO in percent after addition of hybrid materials (first cycle) and their reuse (second cycle) were calculated and the results are shown in . The exact concentration of the untreated MO is 79.2%. The results for treated solutions showed that with increasing quantity of CS/Ti components, the MO concentration was reduced after 4 h of irradiation. The SiCSTi5 sample, where silica and CS/TBOT components are equal, showed the highest adsorption capacity of MO. The percentage of adsorbed/degraded MO increased up to 82 for the same sample. Li et al. [Citation34] investigated the adsorption and photocatalytic activities of CS/TiO2 materials and established that a 90% removal of MO can be reached if the TiO2 is both in a crystal phase and over 40% of the final mass. The synthesized materials possess the similar removal efficiency of pollutant, when the quantity of TiO2 is reduced to 25% and the used semiconductor is in an amorphous state.
The results obtained for the activities of the hybrid materials after 4 h of UV irradiation showed that the synthesized materials can be successfully applied as agents for water purification. Reuse experiments were made to investigate the recombination of the same samples and the results showed that the activities were slightly reduced (for example, %R SiCSTi5 after the first cycle was 82.7%, and after the second cycle was 79.9%).
The reuse experiment and obtained results showed that the synthesized silica hybrid materials can be used many times.
Conclusions
Innovative hybrid materials involving chitosan and TiO2 components were successfully synthesized. The obtained hybrid materials represented a combination of high adsorption and photocatalytic activities into a suitable carrier. The results from structural analysis proved formation of homogeneous structures with free reactive centres. This was achieved by incorporating the components into a silica network by using a sol–gel synthesis and tetraethyl orthosilicate as the silica source. Adsorption/degradation activities against MO were tested and the results showed that the obtained hybrid materials are applicable for environmental remediation purposes and also showed favourable recombination properties.
Disclosure statement
No potential conflict of interest was reported by the authors.
Additional information
Funding
References
- Reddy D, Lee S. Application of magnetic chitosan composites for the removal of toxic metal and dyes from aqueous solutions. Adv Colloid Interface Sci. 2013;201–202:68–93.
- Lee K, Morad N, Teng T, et al. Characterization and the application of hybrid materials in coagulation/flocculation of wastewater: a review. Chem Eng J. 2012;203:370–386.
- Yang D, Li J, Jiang Z, et al. Chitosan/TiO2 nanocomposite pervaporation membranes for ethanol dehydration. Chem Eng Sci. 2009;64:3130–3137.
- Kumar A, Gaurav, Malik A, et al. A review on development of solid phase microextraction fibers by sol–gel methods and their applications. Anal Chim Acta. 2008;610:1–14.
- Singhon R, Husson J, Knorr M, et al. Adsorption of Ni(II) ions on colloidal hybrid organic–inorganic silica composites. Colloids Surf B. 2012;93:1–7.
- Li Q, Su H, Tan T. Synthesis of ion-imprinted chitosan–TiO2 adsorbent and its multi-functional performances. Biochem Eng J. 2008;38:212–218.
- Pillai C, Paul W, Sharma C. Chitin and chitosan polymers: chemistry, solubility and fiber formation. Progr Polym Sci. 2009;34:641–678.
- Liu Y, Su Y, Lee K, et al. Crosslinked organic–inorganic hybrid chitosan membranes for pervaporation dehydration of isopropanol–water mixtures with a long-term stability. J Membr Sci. 2005;251:233–238.
- Gegova R, Dimitriev Y, Bachvarova-Nedelcheva A, et al. Combustion gel method for synthesis of nanosized ZnO/TiO2 powders. J Chem Technol Metal. 2013;48:147–153.
- Xing Y, Li X, Zhang L, et al. Effect of TiO2 nanoparticles on the antibacterial and physical properties of polyethylene-based film. Progr Org Coat. 2012;73:219–224.
- Chen C, Lu CS, Chung C, et al. UV light induced photodegradation of malachite green on TiO2 nanoparticles. J Hazard Mater. 2007;141:520–528.
- Jin R, Wu Z, Liu Y, et al. Photocatalytic reduction of NO with NH3 using Si-doped TiO2 prepared by hydrothermal method. J Hazard Mater. 2009;161;42–48.
- Zubieta CE, Messina PV, Luengo C, et al. Reactive dyes remotion by porous TiO2–chitosan materials. J Hazard Mater. 2008;152:765–777.
- Jawad AH, Nawi MA. Fabrication, optimization and application of an immobilized layer-by-layer TiO2/Chitosan system for the removal of phenol and its intermediates under 45-W fluorescent lamp. React Kinet Mech Catal. 2012;106:49–65.
- Al-Sagheer FA, Merchant S. Visco-elastic properties of chitosan–titania nano-composites. Carbohydr Polym. 2011;85:356–362.
- Calleja G, Serrano PD, Sanz R, et al. Mesostructured SiO2-doped TiO2 with enhanced thermal stability prepared by a soft-templating sol–gel route. Microporous Mesoporous Mater. 2008;111:429–440.
- Ispas C, Sokolov I, Andreescu S. Enzyme-functionalized mesoporous silica for bioanalytical applications. Anal Bioanal Chem. 2009;393:543–554.
- Lin X, Ma T, Yuan Z. Titania–silica–phosphonate triconstituent hybrid mesoporous materials as adsorbents in gas and liquid phases. Chem Eng J. 2011;166:1144–1151.
- Tao Y, Pan J, Yan S, et al. Tensile strength optimization and characterization of chitosan/TiO2 hybrid film. Mat Sci Eng B. 2008;138:84–89.
- Zhang W, Zou L, Wang L. Photocatalytic TiO2/adsorbent nanocomposites prepared via wet chemical impregnation for wastewater treatment: a review. Appl Catal A. 2009;371:1–9.
- Li D, Zheng H, Wang Q, et al. A novel double-cylindrical-shell photoreactor immobilized with monolayer TiO2-coated silica gel beads for photocatalytic degradation of Rhodamine B and Methyl Orange in aqueous solution. Sep Purif Technol. 2014;123:130–138.
- Soltani R, Khatace A, Safari M, et al. Preparation of bio-silica/chitosan nanocomposite for adsorption of a textile dye in aqueous solutions. Int Biodeterior Biodegrad. 2013;85:383–391.
- Zhao J, Zhu Y, Wu J, et al. Chitosan-coated mesoporous microspheres of calcium silicate hydrate: environmentally friendly synthesis and application as a highly efficient adsorbent for heavy metal ions. J Colloid Interface Sci. 2014;418:208–215.
- Mei F, Liu C, Zhang L, et al. Microstructural study of binary TiO2:SiO2 nanocrystalline thin films. J Cryst Growth. 2006;292:87–91.
- Copello G, Mebert A, Raineri M, et al. Removal of dyes from water using chitosan hydrogel/SiO2 and chitin hydrogel/SiO2 hybrid materials obtained by the sol–gel method. J Hazard Mater. 2011;186:932–939.
- Nawi M, Sabar S, Jawad A, et al. Adsorption of Reactive Red 4 by immobilized chitosan on glass plates: towards the design of immobilized TiO2–chitosan synergistic photocatalyst–adsorption bilayer system. Biochem Eng J. 2010;49:317–325.
- Song R, Xue R, He L, et al. The structure and properties of chitosan/polyethylene glycol/silica ternary hybrid organic–inorganic films. Chin J Pol Sci. 2008;26:621–630.
- Zainal Z, Hui L, Hussein M, et al. Characterization of TiO2–chitosan/glass photocatalyst for the removal of a monoazo dye via photodegradation–adsorption process. J Hazard Mater. 2009;164:138–145.
- Gao X, Wachs IE. Titania-silica as catalysts: molecular structural characteristics and physico-chemical properties. Catal Today 1999;51:233–254.
- Dimitriev Y, Dimitrov V. Strukturen analiz: spektralni metodi [Structural analysis: spectral methods]. Sofia: UCTM; 2009. p. 117.
- Huang R, Liu Q, Huo J, et al. Adsorption of methyl orange onto protonated cross-linked chitosan. Arab J Chem. Forthcoming 2013.
- Kim Y, Shao G, Jeon S, et al. Sol–gel synthesis of sodium silicate and titanium oxychloride based TiO2–SiO2 aerogels and their photocatalytic property under UV irradiation. Chem Eng J. 2013;231:502–511.
- Yu K, Zhao J, Tian Y, et al. Preparation of nanosized titanium dioxide from titanium n-butoxide modified with tartaric acid and its influence on the phase transformation. Mater Lett. 2005;59:3563–3566.
- Li Q, Su H, Tan T. Synthesis of ion-imprinted chitosan–TiO2 adsorbent and its multi-functional performances. Biochem Eng J. 2008;38:212–218.