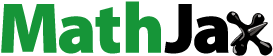
Abstract
Nitrite is known to accumulate in wastewater treatment plants and play an important role during the biological denitrogenation process. In this study, wastewater with high nitrite concentration was effectively treated, and the possible material transformation of nitrite and carbon by microorganisms is discussed herein. While inoculating pre-acclimatized floccular sludge, nitrite-denitrifying granular sludge was obtained after approximately 15 days of cultivation in a denitrifying upflow anaerobic sludge blanket (UASB) reactor. The nitrite removal efficiency ( (%)) was always greater than 98%. At the same time, the nitrite removal rate (
) reached 0.46 g N g VSS−1 d−1. The granular sludge taken from the reactor was characterized. The fluorescence in situ hybridization (FISH) result showed that the microbial community structure was also changed during the granular sludge formation process and that the nitrite denitrifying bacteria increased in population size. Based on the carbon balance, the stoichiometric equation of nitrite denitrification was obtained and a biomass yield value was calculated. The practical ratio of chemical oxygen demand (COD) and nitrite was 2:1, which was higher than the theoretical value. The measured effluent pH was negligibly different from the stoichiometric value.
Introduction
Nitrogen compounds, including ammonia, nitrite and nitrate, are becoming a major environmental concern. When they are discharged in the environment, serious problems are caused, such as eutrophication of rivers and deterioration of water sources, as well as hazards to human health.[Citation1–3] Nitrite is known to cause severe human health issues.[Citation4] Exposure to high nitrite levels through water and feed consumption can also lead to reduced vitality and increased stillbirth rates, low birthweight and slow weight gain in livestock.[Citation5] Therefore, it is necessary to remove nitrite during wastewater treatment in such cases.[Citation6] Denitrification is regarded as an efficient, natural, microbe-mediated nitrogen removal process for soil and water treatment.[Citation7–9] The upflow anaerobic sludge blanket (UASB) reactor technology has been successfully applied to the treatment of various types of wastewater.[Citation10,Citation11] Moreover, it is also widely used specifically in the denitrification field.[Citation12,Citation13] UASB was specially designed not only to retain a high carbon level via nitrite denitrification for sludge, but also to ensure the desired condition of granular sludge formation in order to perform partial nitrification.[Citation14] Most works focus on nitrate denitrification in UASB.[Citation15–18] Consequently, there are a few reports about nitrite denitrification in UASB and the role of material transformation.
Heterotrophic denitrifying bacteria require an organic carbon source for respiration and growth. When biomass yield is not taken into consideration, the heterotrophic denitrifiers remove nitrite by the following stoichiometric equations, Equation (1) [Citation19] and Equation (2) [Citation20]:(1)
(1)
(2)
(2)
The different reactions can be carried out with different carbon sources, such as methanol and acetic acid. As a result, the different carbon sources have different metabolic stoichiometric equations and characteristics of granular sludge, and the denitrification bacteria grow faster than other anaerobic bacteria. If the growth of microorganisms is considered, the stoichiometric equation would be more reasonable.
Therefore, the equation including microorganism growth was amended using nitrogen, carbon and electron balances based upon the reactor's stable operation in this study. The aim of this study was to: (1) evaluate the nitrogen removal feasibility for wastewater with high nitrite concentration in a UASB reactor; (2) investigate the granular sludge formation process and the growth of denitrifying bacteria and (3) determine the transformation stoichiometric equation of the nitrite denitrifying process.
Materials and methods
UASB reactor
Experiments were conducted in a UASB reactor made of transparent poly(methyl methacrylate), as shown in . The inner diameter, height and total effective working volume of the UASB reactor were 80 mm, 700 mm and 3.5 L, respectively. Sampling ports were installed along the height of the column at 150 mm intervals. The gas produced was collected by three-phase separators in the head of the reactor, and the production volume was measured by a wet gas flowmeter. All experiments were carried out at 35 °C.
Inoculum and culture medium
The reactor was inoculated with 32.7 g mixed liquor volatile suspended solids (MLVSS)-activated sludge from the urban sewage treatment plant of Shijiazhuang (Shijiazhuang city, China). Denitrifying sludge was acclimated in this reactor by 50 mg L−1 NO3-N. The composition of the acclimated synthetic wastewater [Citation21] in the reactor was as follows: 1.591 g L−1 C4H4Na2O4 (sodium succinate), 0.543 g L−1 NaNO2, 0.065 g L−1 KH2PO4 and 2 mL L−1 trace element solution. The trace element solution contained 63.68 mg L−1 ethylenediaminetetraacetic acid (EDTA), 2.2 mg L−1 ZnSO4·7H2O, 5.5 mg L−1 CaCl2, 1.61 mg L−1 CoCl2·6H2O, 4.32 mg L−1 MnSO4, 5.00 mg L−1 FeSO4·7H2O, 1.57 mg L−1 CuSO4·5H2O, 2.11 mg L−1 Na2MoO4·2H2O, 1.85 mg L−1 NiCl2·6H2O, 2.50 mg L−1 Na2SeO4·10H2O, 1.45 mg L−1 H3BO4 and 1.35 mg L−1 Na2WO4·2H2O. The operating conditions of the UASB reactor were pH 7.0–7.5 and 35 °C.
Feed composition and reactor operation
Approximately 15.1 g of volatile suspended solids (VSS) pre-acclimatized sludge was added to the UASB reactor, and the mean VSS was 4.33 g L−1 in the set-up period. The synthetic wastewater included NaNO2, C4H4Na2O4 (chemical oxygen demand (COD)/NO2-N in the set-up and steady processes were 3 and 2, respectively) and KH2PO4 (P/NO2-N = 0.1) in deionized water. Because the inoculum sludge was acclimated, the initial nitrite concentration of the influent was 600 mg L−1. Subsequently, the influent NO2-N was increased to 700 and 800 mg L−1 on the 11th and 19th day, respectively, with a flow rate of 1.08 L h−1, a hydraulic retention time (HRT) of 3.24 h and an upflow velocity of 0.215 m h−1.
During the set-up and steady-state processes, the change in the denitrifying bacteria population was evaluated by fluorescence in situ hybridization (FISH). Influent and effluent COD, nitrite concentration, pH and gas were monitored daily, while alkalinity and gas composition were measured weekly. Total organic carbon (TOC) was measured every three days. The concentration of CO32− and HCO3− was detected for the determination of alkalinity, which was determined every five days.
FISH assays
The EUB338 probe (Takara Biotechnology (Dalian) Co., Ltd.) used for granular sludge hybridization had the following sequence: 5′-CGG GAG GCA GCA GT-3′. This probe target position was 338–355 in the 16S rRNA of most bacteria. The probe was labelled at the end with carboxyfluorescein (HEX).
FISH steps were as follows [Citation21]: (1) the defined amount of nitrite denitrification granular sludge was placed in a 1.5 mL centrifuge tube and soaked with 4% paraformaldehyde for 4 h at 4 °C; (2) the granular sludge sample was washed three times by 1× PBS (phosphate buffered saline); (3) the sample was spotted on a gelatin-coated slide, treated with 50%, 80% and 100% ethanol, as a process of dehydration, for 3 min, respectively, and allowed to air dry as previously described; (4) the sludge sample was covered with 10 μL of hybridization buffer (0.9 mol L−1 NaCl, 0.01% sodium dodecyl sulphate (SDS), 20 mmol L−1 Tris–HCl (pH 7.2), 20% deionized formamide) containing 10 nmol L−1 of EUB338 probe; (5) the hybridization was performed for 3 h at 46 °C; then the sludge sample was washed in pre-warmed washing buffer (20 mmol L−1 Tris–HCl (pH 7.2), 0.01% SDS, 2.5 mmol L−1 NaCl) at 46 °C and then washed three times in deionized water; (6) the slides were mounted and visualized by microscopy (Leica SFL4000, Leica Microsystems (Shanghai), Co., Ltd.) at wavelengths of 460–490 nm.
Material balance methods
The UASB reactor showed high and stable treatment efficiency after the denitrification sludge became acclimated. In this condition, TOC and alkalinity were determined, and the gas was detected by gas chromatography (GC) (Tianmei, GC 7900, China). The material transformation relationship was considered by mathematical calculation. The removal rates of NO2−-N and COD were calculated as an average number. The concentrations of CO32− and HCO3− were detected for the determination of alkalinity. A nitrite denitrification stoichiometric equation was obtained by mass balance, from which the relationship between carbon and nitrogen was estimated, and the high pH of the effluent was well explained.
Analytical methods
COD, mixed liquor suspended solids (MLSS), VSS and nitrite concentration were determined according to standard methods.[Citation22] TOC was also analyzed according to standard methods [Citation22] by a total organic carbon instrument (Shimadzu, TOC-VCPN, Japan). The pH was determined by a digital pH meter (Delta-320, China). The gas produced in the reactor was detected and characterized by GC (Tianmei, GC 7900, China).
In this study, the efficiency of nitrite removal and the rate of nitrite removal were described by Equations (3) and (4), respectively:(3)
(3)
(4)
(4)
where is the initial concentration of NO2-N (g L−1);
is the NO2-N concentration (g L−1) at time t; V is the volume of wastewater in the reactor (L); VSS is the VSS in the reactor (g L−1).
Results and discussion
Nitrite denitrification characteristics of UASB
Removal rate of NO2−-N
In the first 10 days, the bulk of flocculant sludge and dispersed particles were washed out of the reactor with the effluent. However, (%) still stabilized over 98%, which suggests that the nitrite denitrification sludge was highly active. Subsequently, the nitrite loading rate increased to 2.4 g NO2−-N L−1 d−1 (), accelerating the formation of granular sludge. After the nitrite-loading rate increased from 2.4 to 3.6 g NO2−-N L−1 d−1,
(%) stabilized over 98% with an effluent nitrite concentration decreasing to 10 mg L−1. The effluent was clear, and the interface of solid and liquid was distinct.
Figure 2. Nitrite removal performance in UASB: (a) NO2−-N concentration and removal rate change; (b) nitrite loading and sludge bed height change.
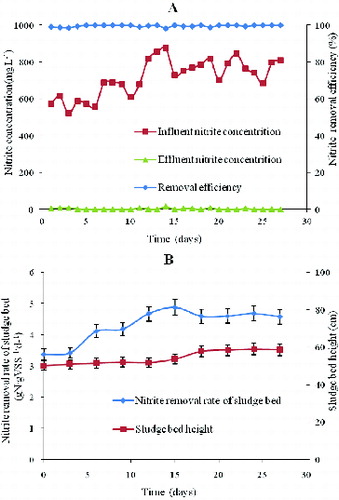
reported in this study was compared with previous published values (). In these experiments, aerobic granules were used to remove high nitrite concentration, and the nitrite-denitrifying activity had been previously promoted. However, in the present study,
was 0.46 g N g VSS−1 d−1, which is higher than other reported rates. It was revealed that nitrite-denitrifying granular sludge could treat nitrite wastewater effectively under a relatively high loading rate.
Table 1. Removal rates of nitrite and nitrite volumetric loading rates reported in literature.
Adav et al. [Citation23] reported that high nitrite concentration had a toxic effect on the microorganisms and of granular sludge decreased from 0.22 to 0.08 g N g VSS−1 d−1. Beccari et al. [Citation24] found that the biomass concentration regulated the inhibitory effect on denitrification together with the pH and nitrite concentration. At a pH of 8.0–8.9, nitrite concentration reached 2000 mg NO2−-N L−1, which showed no inhibitory effect on the denitrifying biofilm.[Citation25] In this study, there was no inhibitory effect when
of granular sludge was 0.46 g N g VSS−1 d−1. Based on the high pH (approximately 10.45), the free nitrous acid concentration (1.75 × 10−5 mg HNO2-N L−1) in the UASB reactor was far below the range of 0.01–0.025 mg HNO2-N L−1.[Citation26,Citation27] Thus, the nitrous acid inside the cells might be very low (no data) and therefore would not inhibit the nitrite denitrifiers.
COD removal rate and TOC change during the set-up process
Organic carbon is an important factor for heterotrophic denitrification, which influences the degradation of nitrite. (a) shows the COD change between influent and effluent. Based upon a high nitrite removal rate, the effluent COD was always less than 200 mg L−1. During the denitrification period, COD was three times the stoichiometric concentration needed to reduce nitrite. After 20 days, the effluent COD stabilized at approximately 50 mg L−1. The result indicated that the COD was sufficient to complete aerobic denitrification.
(b) shows the influent and effluent TOC change in UASB with a high carbon concentration and a high nitrogen loading rate. Sodium succinate [Citation12,Citation28] was the only carbon source in the denitrification process, and the detected gas did not contain carbon dioxide, which might be produced in the reactor. The average TOC difference between influent and effluent was 72.02 mg L−1, which can be used by denitrification organisms under anoxic conditions in UASB. TOC played an important role for microbial growth and provided the basis for the calculation of microbial growth (see Section ‘Material balance’).
pH change during the set-up process of UASB
The pH change between influent and effluent for the reactor performance is shown in . The effluent pH depended mainly on the organic carbon resource and NO2−-N concentrations. The influent pH ranged between 6.0 and 8.0; however, the effluent pH was maintained at approximately 10.45, which shows that the reactor worked well. Furthermore, the pH value of the effluent was different from that obtained by Jin et al. [Citation20]. A reasonable explanation for this difference would be that bicarbonate was discovered in the effluent (for further, more detailed discussion, see Section ‘Material balance’).
Characteristics of nitrite denitrification granular sludge
Three pictures in illustrate the change of sludge morphology in the UASB reactor. In the first picture ((a)), the denitrification sludge was allowed to acclimate for one month in a small anaerobic reactor (2 L), which efficiently removed nitrite, and the sludge was spread and blackened due to an effective sludge setting. After 15 days ((b)), the sludge in the UASB reactor became granular, and a milky secretion appeared in one half of the reactor bottom. When the reactor had operated stably for 27 days ((c)), the fine granules in the reactor turned yellowish brown in colour and became bigger and irregular in shape. In this process, (%) remained above 98%.
The formation of granular sludge in the UASB reactor was the key factor for the reduction of high nitrite and carbon concentrations. Consequently, the microorganisms were able to adapt to the denitrification environment, the high nitrite concentration and hydraulic shear forces. The high nitrite removal rate and hydraulic shear force were important factors in the process of formation of denitrification granular sludge.
FISH of denitrification granular sludge in the UASB reactor
The denitrification granular sludge was cultivated in synthetic wastewater with nitrite. The effect of high nitrite concentration on microbial populations was evaluated by FISH over different treatment times. shows that the abundance of the microbial population in the denitrification granular sludge was different at different points during treatment (1st, 15th and 27th day). The microbial population was minimal during the initial period (1st day; (a)), likely because the nitrite denitrification sludge was cultured under the condition of lower nitrite concentration. Then, the microbial population size increased after the 15th day of treatment ((b)). After the initial environmental stress due to the increased nitrite concentration in the influent, the microbial community adapted to the denitrification environment with high nitrite (after the 27th day), which most likely caused the subsequent increase in microbe abundance ((c)). The relative abundances were 10%, 18% and 30% during the three different periods, respectively. The results showed that nitrite denitrifying bacteria were widely present in the denitrifying environment. The denitrifying granular sludge could reduce nitrite with sodium succinate when the carbon source was in conjunction with a higher nitrite concentration and greater hydraulic shear forces.
Material balance
The low nitrite concentration (lower than 10 mg L−1) and high COD (100 mg L−1) of effluent suggested that the carbon source was sufficient and denitrification was fully achieved.
(a) shows the main theoretical gas compositions for denitrification in the UASB reactor. Nitrogen, methane and carbon dioxide are distinguished by different time of peak outflow. (b) shows the gas composition of nitrite denitrification in this study and that nitrogen was detected as the only component. The absence of methane was most probably caused by the high nitrite concentration, which inhibits the activity of methanogens, and COD was mainly used to reduce nitrite during the denitrification process.[Citation29] Carbon dioxide was also undetected in the gas mixture. This could be due to the fact that carbon dioxide might dissolve in the effluent in the form of CO32− and HCO3−. To check this possibility, the effluent TOC and alkalinity in the UASB reactor were measured. The results presented in confirmed the presence of CO32− and HCO3− in the effluent.
Table 2. Effluent TOC and alkalinity in UASB reactor.
shows the nitrite concentration and COD of influent and effluent, and the calculated NO2−-N removal rate together with the average COD and TOC consumption. As calculated, the average NO2−-N consumption was 652.33 mg L−1. The average COD was 1326.72 mg L−1, which had eliminated the interference of nitrite. Assuming that the difference in TOC between influent and effluent was used for the growth of denitrifying bacteria (C5H7NO2 was used instead of biomass), the biomass concentration was 0.001 mol L−1. The mean mass concentration of HCO3− was 185.8 mg L−1 and the molar concentration was 0.0155 mol L−1, while the mean mass concentration of CO32− was 370 mg L−1 and the molar concentration was 0.0308 mol L−1.
Table 3. Operational parameters and performance of UASB (average of all data).
From and , the molar ratio of C4H6O42:HCO3−:CO32− was 1:15:30. According to the calculated result of mass and electron balance, the reaction equation describing the substrate transformation for this UASB reactor was derived as Equation (5):(5)
(5)
As observed in Equation (3), the theoretical ratio of COD and nitrogen was calculated to be 1.96:1, which was a little lower than the actual ratio (2.0:1). The COD exceeded the stoichiometric demand presented in the feed. The demand for electron donors was most probably needed to reduce any oxygen entering the anaerobic bioreactors, as reported by Atikovic et al. [Citation30].
Based on Equation (4), the effluent pH during the nitrite denitrification process was considerably lower than the theoretical pH value expected (11.38), which was calculated using the ionization constants of OH−.[Citation20] For nitrite denitrification, the effluent pH value can be calculated by Equation (6) with the different ionization constants of HCO3− and CO32−:(6)
(6)
where pKa is the HCO3− dissociation constant (10.25). According to Equation (6), the theoretical pH value of the effluent in this study was calculated to be 10.56, which was close to the measured value (approximately 10.45). This result agrees with the very high pH increase observed in a USB reactor for nitrite denitrification [Citation20] and suggests that a high effluent pH should enhance the precipitation potential of calcium carbonate as the core of the formation of granule sludge. Therefore, the features of nitrite granular sludge (i.e., high ash content, dense structure and good settleability) were attributed to the release of alkalinity and subsequently increased the pH value by nitrite denitrification. In fact, Green et al. [Citation31] showed that a high pH (close to 9.0) was a key factor in obtaining heavy granular sludge in their denitrification study using a UASB reactor.
Taken together, our results suggest that the studied UASB reactor can be considered feasible for nitrogen removal from wastewater with high nitrite concentration. The observed granular sludge formation and growth of denitrifying bacteria as well as the transformation stoichiometric equation of the nitrite denitrifying process are promising for the application of the denitrifying granular sludge. Further studies at a larger scale and with real contaminated water are needed to determine the economic viability of the proposed process.
Conclusions
To the best of our knowledge, this is the first report on the formation process of nitrite denitrification granular sludge in a UASB reactor, and reached 0.46 g N g VSS−1 d−1. In the process, the nitrite-denitrifying activity had been previously promoted due to the increase of nitrite denitrification bacteria in the microbial community. In the case of stable operation, the equation for the rate of denitrification was obtained based on the material balance, which included the biomass yield. The theoretical ratio of COD and nitrogen was calculated to be 1.96:1, which was a little lower than the practical ratio (2:1). At the same time, the high pH (10.45) of effluent was close to the stoichiometric value calculated, which contributed to the heavy granular sludge. This indicates that the material transformation in the denitrification process could be considered more effective and the conditions of each treatment performance could be optimized. This paper provides an effective technology for nitrite treatment that could assist in the future application of shortcut nitrification–denitrification processes.
Disclosure statement
No potential conflict of interest was reported by the authors.
Additional information
Funding
References
- Morita M, Uemoto H, Watanabe A. Nitrogen-removal bioreactor capable of simultaneous nitrification and denitrification for application to industrial wastewater treatment. Biochem Eng J. 2008;4:59–66.
- Fernández-Nava Y, Marañón E, Soons J, et al. Denitrification of high nitrate concentration wastewater using alternative carbon source. J Hazard Mater. 2010;173:682–688.
- Pous N, Puig S, Coma M, et al. Bioremediation of nitrate-polluted groundwater in a microbial fuel cell. J Chem Technol Biotechnol. 2013;88:1690–1696.
- Yang XP, Wang SM, Zhou LX. Effect of carbon source, C/N ratio, nitrate and dissolved oxygen concentration on nitrite and ammonium production from denitrification process by Pseudomonas stutzeri D6. Bioresour Technol. 2012;104:65–72.
- Sun YM, Nemati M. Evaluation of sulfur-based autotrophic denitrification and denitritation for biological removal of nitrate and nitrite from contaminated waters. Bioresour Technol. 2012;114:207–216.
- Sison NF, Hanaki K, Matsuo T. Denitrification with external carbon source utilizing adsorption and desorption capability of activated carbon. Water Res. 1996;30:217–227.
- Dahab MF. Treatment alternatives for nitrate contaminated groundwater supplies. J Environ Syst. 1988;17:65–75.
- Rivett MO, Buss SR, Morgan P, et al. Nitrate attenuation in groundwater: a review of biogeochemical controlling processes. Water Res. 2008;2:4215–4232.
- Aelion CM, Warttinger U. Low sulfide concentrations affect nitrate transformations in freshwater and saline coastal retention pond sediments. Soil Biol Biochem. 2009;41:735–741.
- Zhang D, Verstraete W. The anaerobic treatment of nitrite containing wastewater using an Expanded Granular Sludge Bed (EGSB) reactor. Environ Technol. 2001;22:905–913.
- Letting G. Anaerobic digestion and wastewater treatment systems. Antonie Van Leeuwenhoek. 1995;67:3–28.
- Cuervo-López F, Martinez F, Gutiérrez-Rojas M, et al. Effect of nitrogen loading rate and carbon source on denitrification and sludge settleability in upflow anaerobic sludge blanket (UASB) reactors. Water Sci Technol. 1999;40:123–130.
- Martínez S, Cuervo-López FM, Gomez J. Toluene mineralization by denitrification in an up flow anaerobic sludge blanket (UASB) reactor. Bioresour Technol. 2007;98:1717–1723.
- Wong FS, Fang W, Moy YP, et al. The application of UASB-MBR with partial nitrification–denitrification in wastewater reclamation in warm climate. J Water Reuse Desalination. 2012;2:102–108.
- Rusten B, Hem LJ, Odegaard H. Nitrogen removal from dilute wastewater in cold climate using moving-bed biofilm reactors. Water Environ Res. 1995;67:65–74.
- Kondo M, Hozo S, Inamori Y. Simultaneous removal of BOD and nitrogen with anoxic/oxic porous biomass support systems. Water Sci Technol. 1992;26:2003–2006.
- Pagáčová P, Galbová K, Drtil M, et al. Denitrification in USB reactor with granulated biomass. Bioresour Technol. 2010;101:150–156.
- Wan CL, Yang X, Lee DJ, et al. Aerobic denitrification by novel isolated strain using NO2−-N as nitrogen source. Bioresour Technol. 2011;102:7244–7248.
- Chen C, Rena NQ, Wang AJ, et al. Enhanced performance of denitrifying sulfide removal process under micro-aerobic condition. J Hazard Mater. 2010;179:1147–1151.
- Jin XB, Wang F, Liu G, et al. Characteristics of denitrifying granular sludge grown on nitrite medium in an upflow sludge blanket (USB) reactor. Water Sci Technol. 2011;65:1420–1427.
- Li HB, Guo JB, Lian J, et al. Effective and characteristics of anthraquinone-2,6-disulfonate (AQDS) on denitrification by Paracoccus versutus sp. GW1. Environ Technol. 2013;34:2563–2570.
- APHA. Standard methods for the examination of water and wastewater. 21st ed. Washington, DC: American Public Health Association; 2005.
- Adav SS, Lee DJ, Lai JY. Enhanced biological denitrification of high concentration of nitrite with supplementary carbon source. Appl Biochem Biotechnol. 2010;85:773–778.
- Beccari M, Passino R, Ramadori R, et al. Kinetics of dissimilatory nitrate and nitrite reduction in suspended growth culture. J Water Pollut Control Fed. 1983;55:58–64.
- Chen SK, Juaw CK, Cheng SS. Nitrification and denitrification of high-strength ammonium and nitrite wastewater with biofilm reactors. Water Sci Technol. 1991;23:1417–1425.
- Zhou Y, Oehmen A, Lim M, et al. The role of nitrite and free nitrous acid (FNA) in wastewater treatment plants. Water Res. 2011;45:4672–4682.
- Abeling U, Seyfried CF. Anaerobic–aerobic treatment of high-strength ammonium wastewater – nitrogen removal via nitrite. Water Sci Technol. 1992;26:1007–1015.
- Martínez-Hernández S, Olguín EJ, Gómez J, et al. Acetate enhances the specific consumption rate of toluene under denitrifying conditions. Arch Environ Contam Toxicol. 2009;57:679–687.
- Hendriksen HV, Ahring BK. Integrated removal of nitrate and carbon in an upflow anaerobic sludge blanket (UASB) reactor: operating performance. Water Res. 1996;30:1451–1458.
- Atikovic E, Suidan MT, Maloney SW. Anaerobic treatment of army ammunition production wastewater containing perchlorate and RDX. Chemosphere. 2008;72:1643–1648.
- Green M, Tarre S, Schnizer M, et al. Groundwater denitrification using an upflow sludge blanket reactor. Water Res. 1994;28:631–637.