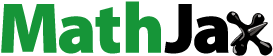
Abstract
Vitamin D and its analogues that are often used in the treatment of osteoporosis have also been shown to affect the healing of bone fractures. To avoid the high-dose systemic treatments, needed to achieve faster healing of fractured bone, the approach of local application of these drugs has become very attractive. The aim of our study was to examine the influence of cholecalciferol and alfacalcidol, locally applied with bovine bone mineral matrix (Bio-Oss), supplemented with platelet-rich plasma on femur defect healing in ovariectomized rats. Experimental osteoporosis was induced by bilateral ovariectomy. To estimate the healing of defects, the formation of the new bone tissue and the resorption of Bio-Oss particles after two and eight weeks of defect healing, we performed biochemical, histological, immunohistochemical, histomorphometric and scanning electron microscopy analyses. Our results showed that locally applied cholecalciferol and alfacalcidol delay early bone healing of defect in ovariectomized rat model. Both of the examined vitamins, particularly alfacalcidol, decreased the resorption of Bio-Oss particles in defects. Only alfacalcidol affected the formation of histologically normal new bone tissue with a good integration into the surrounding osteoporotic bone. Cholecalciferol allowed callus mineralization and led to the formation of new bone with high quantity, with characteristics, similar to those of the control group. Our results suggested that alfacalcidol and cholecalciferol may be promising tools for the local treatment of osteoporotic fractures, but the goal of application should specify the choice of vitamin D form, as well as the composition of scaffolds, with which they are applied.
Introduction
Osteoporosis, a disease characterized by reduced bone mineral density (BMD), strength and quality, is a great problem in stomatology and orthopaedics, because of the bone loss and the reduced capacity of the osteoporotic bone to provide the necessary support for an implant. The major threats for patients, suffering from osteoporosis, are fractures that are very difficult to heal in osteoporotic conditions. Therefore, it is favourable to use drugs and bone substitutes that will improve the bone formation and strengthen the site of the fracture.
Local drug delivery has become an attractive approach in recent years, because, in this way, it is possible to avoid the side effects of the high-dose systemic treatments, needed to achieve faster healing of the fractured bone.[Citation1] Vitamin D, often used in the treatment of osteoporosis, has been shown to improve the healing of fractures in non-osteoporotic,[Citation2,Citation3] as well as in ovariectomized rats model,[Citation4,Citation5] when applied orally. Anyway, its local application, in combination with bone substitutes, is poorly investigated in osteoporotic bone repair. It has been shown that cholecalciferol exerts positive impact on osteogenesis and callus characteristics when delivered locally with hydroxyapatite (HAp) nanoparticles.[Citation6] Local effect of vitamin D precursors seems possible, due to their local conversion to the active metabolite 1,25-dihydroxyvitamin D3 in osteoblasts.[Citation7,Citation8] Biomaterials based on HAp are often used as bone substitutes alone, or as a component of implants, showing among other properties a high capacity to absorb vitamin D.[Citation9] In regenerative medicine, supplementation with platelet-rich plasma (PRP) enhances the osteoinductivity of bone substitute biomaterials and improves bone regeneration.[Citation10] It is also known that co-treatment with PRP and 1,25-dihydroxyvitamin D3 stimulate osteogenic differentiation in vitro.[Citation11]
The aim of our study was to examine the influence of cholecalciferol and alfacalcidol, locally applied in combination with bovine bone mineral matrix, supplemented with PRP, on femur defect healing in ovariectomized rats. Additionally, we examined the influence of these two vitamin D forms on the characteristics of the new bone tissue formed under the osteoporotic conditions. These are our preliminary results of a wider study that is still in progress.
Materials and methods
Chemicals and materials
Alfacalcidol and cholecalciferol were purchased from Sigma (Sigma-Aldrich, St. Louis, MO) and were dissolved, according to the manufacturer's recommendations. Bio-Oss® granules, size S (Geistlich, Switzerland), were used as a bone mineral matrix.
Animals
Forty female Wistar rats, three month old, weighting 180–200 g at the beginning of the experiment, were used in this study. The rats were kept under standard conditions, with a 12-hour light–dark cycle, free access to water and food and at a temperature of 22 °C–24 °C. Animal procedures were approved by the Local Ethical Committee of the Faculty of Medicine, University of Niš, Serbia (No. 01-6481-18), in accordance with the Directive 2010/63/EU of the European Parliament and of the Council of 22 September 2010 on the protection of animals used for scientific purposes.[Citation12]
Induction of osteoporosis
Experimental osteoporosis was induced by bilateral ovariectomy, made by two dorsolateral incisions, according to the previously described method [Citation13] under an intraperitoneal anaesthesia (10% ketamine, 0.1 mL/100 g of body weight). Osteoporosis was confirmed three months after the ovariectomy, by using computed tomography analysis and measuring the levels of estrogen (data not shown).
Experimental design
All 40 ovariectomized animals were subjected to the creation of full depth defects in the distal part of both of the femoral diaphysis, according to Tielinen et al.[Citation14] After the animals received anaesthesia, the defects were made with a 2 mm in diameter drill. There were four experimental groups, two control and two treatment groups, with 10 animals per group. One half of the animals from each group was sacrificed after two weeks of healing (2W) and the other half after the eight-week healing period (8W). Negative control group consisted of animals with empty defects and spontaneous regeneration (SR group). Scaffold control group (SC group) consisted of animals with defects, filled with Bio-Oss, supplemented with PRP. The animals in the treatment groups (vitamin D groups) had defects, filled with implants, made of cholecalciferol (CC group) or alfacalcidol (AC group) carried on scaffold (Bio-Oss + PRP).
PRP was prepared according to the method, described by Intini et al. [Citation15] in about six fold higher thrombocyte concentration, when compared to the physiological one.[Citation16] The mixture of liquid components (vitamin D solution and PRP suspension) was allowed to adsorb on Bio-Oss particles at room temperature for about half an hour prior to implantation. In implants, Bio-Oss and liquid components were mixed in a ratio of 1:1, whereas the ratio vitamin D/PRP was 3:1 with 2 pg of alfacalcidol per implant in the AC group and 2 ng of cholecalciferol per implant in the CC group. After the surgical procedure was finished, we applied local injections of 200 µL alfacalcidol or cholecalciferol solution at concentrations of 5 × 10−7 mol/L or 5 × 10−4 mol/L, respectively, into the defect area to refill the implants and to ensure high concentration of vitamins at the site of defects in the first three days of healing. The treatment with vitamin D, used in our study, was slightly modified in comparison to the previously described treatment, which included the subcutaneous application of the vitamin.[Citation17] SR and SC groups obtained the same amount of saline solution to achieve the same experimental conditions.
Biochemical analysis
Serum levels of alkaline phosphatase (ALP), acid phosphatase (AP), calcium (Ca) and phosphorus (P) were determined by using a spectrophotometric method on an automatic chemistry analyzer (Erba XL-600, Mannheim, Germany).
Histological, histomorphometrical and immunohistochemical analysis
Both femora of each rat were dissected and their distal parts, containing the defects, were fixed in periodate–lysine–paraformaldehyde fixative, decalcified in ethylenediaminetetraacetic acid-glycerol (EDTA-G) solution and processed to obtain paraffin embedded tissue. Paraffin blocks were cut at 4 µm on a microtome (LKB Bromma 2218 HistoRange Microtome). Hematoxylin and eosin (H&E) and Masson's trichrome staining were performed. Tissue sections were then analysed on Leica microscope (Leica DC300, Germany).
Histomorphometrical analysis was performed on H&E stained slides (× 50) in NIS-Elements software version 3.2 (Nikon, Tokyo, Japan). By using ‘Annotations and Measurements’ tool in the NIS-Elements software, we measured the total defect area, total area of the material granules and total area of the newly formed bone. Per cent of the residual material and per cent of the newly formed bone were calculated, according to the following equations:
The expression level of osteocalcin (OC) was determined by using anti-osteocalcin antibody (1:200, ab93876, Abcam, Burlingame, CA, USA). HRP/DAB detection IHC kit for visualization (ab64261, Abcam) was used, according to the manufacturer's protocol.
Scanning electron microscopy analysis (SEM)
Distal parts of the femora, containing the defect were fixed in 2.5% glutaraldehyde (G5882 Sigma-Aldrich), were post-fixed in 1% osmium tetroxide and dehydrated through increased series of ethanol and acetone. Samples were dried by the procedure of critical point drying, coated with gold and observed under scanning electron microscope (JEOL, JSM 5300).
Statistical analysis
Statistical analyses of data were performed in SPSS software, version 17.0 (SPSS, Chicago, IL, USA) by using Kruskal–Wallis and Mann–Whitney tests for analysing the biochemical data and analysis of variance for the histomorphometric data. Differences with P ≤ 0.05 were considered statistically significant.
Results and discussion
Drill-hole defect model, obtained according to Tielinen et al.,[Citation14] is easily reproducible, does not require complicated intervention and is the way to avoid large trauma and risk for the animal. The concentrations of vitamin D, used in our experiment, were based on the effective doses that stimulate bone formation [Citation17,Citation18] and do not cause hypercalcemia ( and ).
Table 1. Concentration of analysed serum biochemical markers in ovariectomized rats after two weeks of healing.
Table 2. Concentration of analysed serum biochemical markers in ovariectomized rats after eight weeks of healing.
Our results showed that there were differences between the experimental groups, when compared to the control groups. Hyperplasia of connective tissue cells that usually occurs as a consequence of a strong inflammatory reaction during the reparative process was observed on histological sections of the SC group ((B)), but it was not detected to that extent in the vitamin D groups ((C) and 1(D)) and in the SR group ((A)). These results indicated that the examined vitamin D forms decreased the tissue reaction to injury and to the inserted implant and, thus, prevented the accumulation of connective tissue in the defect, which was observed in the SC group after eight-week healing period ((B) and 2(F)) and was not indicated in vitamin D groups ((C) and 2(D)). It is already known that vitamin D inhibits the release of pro-inflammatory cytokines (also known as pro-resorptive cytokines, such as interleukin-1, interleukin-6 and tumour necrosis factor alpha) from macrophages [Citation19] and, thus, downregulates an inflammatory reaction, altering, at the same time, the bone resorption.[Citation20] Locally applied vitamin D at the early phase of bone healing, might be the justification for its potential clinical use, in order to avoid problems in fracture healing, such as irregular fusion and pseudarthrosis outcome.
Figure 1. Defect healing in ovariectomized rats after the two-week healing period in SR group (A) and (E), SC group (B) and (F), CC group (C) and (G) and AC group (D) and (H). Hyperplasia of connective-tissue cells (CT), bony callus (BC), implanted Bio-Oss (★), repopulated and integrated Bio-Oss particles in new bone (★), collagen (Col). Up – H&E, 200 μm scale bar; Down – Masson's trichrome, 50 μm scale bar.
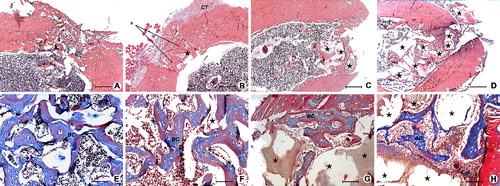
Figure 2. Defect healing in ovariectomized rats after the eight-week healing period in SR group (A) and (E), SC group (B) and (F), CC group (C) and (G) and AC group (D) and (H). Connective tissue (CT), boundary between old and new bone (Bd), mature bone (MB), immature bone incurred in remodelling (IB), implanted Bio-Oss (★), remodelation site (RS). Up – H&E, 200 μm scale bar; Down – Masson's trichrome, 50 μm scale bar.
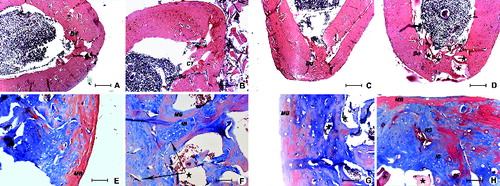
Results that show the influence of the examined vitamins on bone resorption were based on the significant decrease in the serum levels of AP, which remained low during the observation period in the AC group ( and ) and the large amount of remaining Bio-Oss material, noticed in both vitamin D groups ((D), (G) and (A)). Bio-Oss particles were also used in our study, as a tool for assessing the influence of cholecalciferol and alfacalcidol on resorption. The low percentage of the newly formed bone, found in the AC-8W group, compared to the CC-8W group ((B)), taken together with the higher percentage of residual material in AC-8W group ((A)) indicated that alfacalcidol has more pronounced antiresorptive potential than cholecalciferol in the case of the applied scaffold. It seems that high inability in Bio-Oss resorption was a drawback for the new bone formation in the AC group. Results of strong antiresorptive properties of alfacalcidol were confirmed in many other studies.[Citation18,Citation21] This also represents the advantage of alfacalcidol over the cholecalciferol in BMD preservation.[Citation22] The quantity of the newly formed bone in the defect area was the highest in the CC-8W group. This could mean that the cholecalciferol enhanced callus formation, when compared to the alfacalcidol receiving group and the two control groups during the later observation period ((B)). The assessment of the treatment efficacy in healing process of the applied vitamin D forms obviously depends on the parameters, based on which the assessment is performed. It should also be taken into account that a scaffold with different resorptive characteristics than Bio-Oss + PRP might give different results.
Figure 3. Per cent of the residual material (A) and per cent of the newly formed bone (B) in the defects after two-week and eight-week period of healing. *P < 0.05, **P < 0.01.
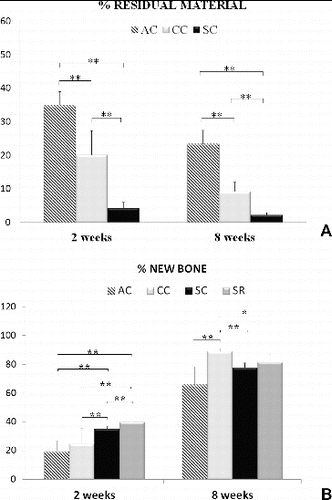
At the two-week time point, a small amount of the bony callus was noticed in vitamin D groups ((C), 1(D), (B), (C) and 4(D)) in comparison with the control groups ((A), (B), (A) and (B)). These results point out the delay in bone healing in both of the vitamin D groups, in comparison with the control groups. This means that cholecalciferol and alfacalcidol impeded the early bone regeneration process. Postponed bone formation was also noticed by using non-steroidal anti-inflammatory drugs.[Citation23] This could indicate that the key of the delayed healing may be exactly the impact on the initial inflammatory phase.
Figure 4. SEM micrographs of the defect area in SR group (A) and (E), SC group (B) and (F), CC group (C) and (G) and AC group (D) and (H) two weeks (up) and eight weeks (down) after the defect was made. Immature bony callus (BC), old bone (OB), new bone (NB), connective tissue (CT), residual material (BO).
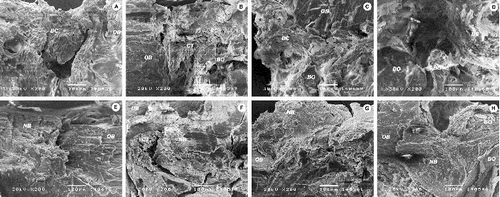
Osteocalcin positive ‘osteocyte-like’ cells that were found in the newly formed bone in CC-2W group ((C)) and CC-8W group ((G)), indicated a wider distribution of this calcium binding matrix protein, which contributed to the mineralization of the bone in CC group in contrast to the other groups ((A), 5(B), 5(D)–(F) and 5(H)). Unlike cholecalciferol that allowed callus maturation with accompanying mineralization ((G) and (G)), alfacalcidol led to weaker or delayed mineralization, but higher production of ‘collagen-rich bone’ ((H) and (H)). Only treatment with alfacalcidol enhanced the organization of collagenous fibres in parallel bundles, which can be noticed clearly on the SEM micrograph of AC group ((H)) but cannot be noticed on micrographs of other groups ((E)–(G)). Positive alfacalcidol impact on the quality of collagen was also obtained in earlier studies.[Citation5] Considering OC negative areas, observed in the callus of the SR-2W group ((A)), both vitamins showed to accelerate the peak of OC expression under the osteoporotic condition, which is consistent with previously published study.[Citation24]
Figure 5. Osteocalcin expression after the two-week (up) and eight-week (down) defect healing period in SR group (A) and (E), SC group (B) and (F), CC group (C) and (G) and AC group (D) and (H) of ovariectomized rats. Arrow – osteocalcin positive “osteocyte-like” cells, 25 μm scale bar.
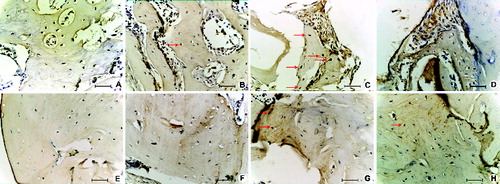
Based on the visibility of boundaries between the old bone and the new bone ((A)–(D)), as well as between the immature bone, incurred in remodelling and the surrounding new bone ((E)–(H)), alfacalcidol showed to improve the integration processes in AC-8W group. Callus in AC-8W group seemed to be remodelled in a histologically normal bone tissue with a good compactness and integration into the existing osteoporotic bone ((D) and 2(H)). In earlier researches, orally administered vitamin D forms also showed a positive effect on fracture healing, including mechanical strength, integrity, remodellation (remodelling) and microstructure restoration.[Citation3,Citation4]
Conclusions
Locally applied cholecalciferol and alfacalcidol differ in their action on healing of femur defects, filled with bone mineral matrix and PRP in ovariectomized rats, both between themselves and compared with control groups. The examined vitamin D forms showed antiresorptive properties, impeded the early bone regeneration process and prevented accumulation of connective tissue in the defect. Applied alfacalcidol had more pronounced antiresorptive potential than cholecalciferol and promoted the formation of histologically normal bone tissue with а well organised collagen matrix and good integration into the osteoporotic bone. The ultimate outcome of the applied cholecalciferol was the generation of higher amount of better calcified new bone. Considering the diverse effects of the examined forms of vitamin D in combination with the Bio-OSS + PRP scaffolds, the goal of the application should specify the choice of vitamin D form.
Acknowledgements
The authors would like to express their gratitude to Marija Vojvodić, the proofreader.
Disclosure statement
No potential conflict of interest was reported by the authors.
Additional information
Funding
References
- Kyllönen L, D'Este M, Alini M, Eglin D. Local drug delivery for enhancing fracture healing in osteoporotic bone. Acta Biomater. 2015;11:412–434.
- Brumbaugh PF, Speer DP, Pitt MJ. 1 alpha, 25-dihydroxyvitamin D3 a metabolite of vitamin D that promotes bone repair. Am J Pathol. 1982;106(2):171–179.
- Delgado‐Martínez A, Martinez M, Carrascal M, et al. Effect of 25‐OH‐vitamin D on fracture healing in elderly rats. J Orthop Res. 1998;16(6):650–653.
- Fu L, Tang T, Miao Y, et al. Effect of 1,25-dihydroxy vitamin D3 on fracture healing and bone remodeling in ovariectomized rat femora. Bone. 2009;44(5):893–898.
- Saito M, Shiraishi A, Ito M, et al. Comparison of effects of alfacalcidol and alendronate on mechanical properties and bone collagen cross-links of callus in the fracture repair rat model. Bone. 2010;46(4):1170–1179.
- Ignjatović N, Uskoković V, Ajduković Z, Uskoković D. Multifunctional hydroxyapatite and poly (D, L-lactide-co-glycolide) nanoparticles for the local delivery of cholecalciferol. Mater Sci Eng C. 2013;33(2):943–950.
- van Driel M, Koedam M, Buurman CJ, et al. Evidence for auto/paracrine actions of vitamin D in bone: 1α-hydroxylase expression and activity in human bone cells. FASEB J. 2006;20(13):2417–2419.
- Ichikawa FSK, Nanjo M, Nishii Y, et al. Mouse primary osteoblasts express vitamin D3 25-hydroxylase mRNA and convert 1 alpha-hydroxyvitamin D3 into 1 alpha,25-dihydroxyvitamin D3. Bone. 1995;16:129–135.
- Ozeki K, Aoki H, Fukui Y. The effect of adsorbed vitamin D and K to hydroxyapatite on ALP activity of MC3T3-E1 cell. J Mater Sci. 2008;19(4):1753–1757.
- Hanna R, Trejo PM, Weltman RL. Treatment of intrabony defects with bovine-derived xenograft alone and in combination with platelet-rich plasma: a randomized clinical trial. J Periodontol. 2004;75(12):1668–1677.
- Feng Y, Sun Y, Jia W, Zhang C. Platelet-rich plasma and 1, 25 (OH) 2 vitamin D3 synergistically stimulate osteogenic differentiation of adult human mesenchymal stem cells. Biotechnol Lett. 2010;32(5):635–642.
- Directive 2010/63/EU of the European Parliament and of the council of 22 September 2010 on the protection of animals used for scientific purposes. Official Journal of the European Union. 2010;L276:33–79. Available from: http://eur-lex.europa.eu/legal-content/EN/TXT/PDF/?uri=CELEX:32010L0063&from=EN
- Lasota A, Danowska-Klonowska D. Experimental osteoporosis-different methods of ovariectomy in female white rats. Rocz Akad Med Bialymst. 2004;49(Suppl 1):129–131.
- Tielinen L, Manninen M, Puolakkainen P, et al. Inability of transforming growth factor-β1, combined with a bioabsorbable polymer paste, to promote healing of bone defects in the rat distal femur. Arch Orthop Trauma Surg. 2001;121(4):191–196.
- Intini G, Andreana S, Intini FE, et al. Calcium sulfate and platelet-rich plasma make a novel osteoinductive biomaterial for bone regeneration. J Translational Med. 2007;5:1–13.
- Marx RE. Platelet-rich plasma: evidence to support its use. J Oral Maxillofac Surg. 2004;62(4):489–496.
- Erben RG, Scutt AM, Miao D, et al. Short-term treatment of rats with high dose 1, 25-dihydroxyvitamin D3 stimulates bone formation and increases the number of osteoblast precursor cells in bone marrow. Endocrinology. 1997;138(11):4629–4635.
- Shiraishi A, Takeda S, Masaki T, et al. Alfacalcidol inhibits bone resorption and stimulates formation in an ovariectomized rat model of osteoporosis: distinct actions from estrogen. J Bone Mineral Res. 2000;15(4):770–779.
- Zhang Y, Leung DY, Richers BN, et al. Vitamin D inhibits monocyte/macrophage proinflammatory cytokine production by targeting MAPK phosphatase-1. J Immunol. 2012;188(5):2127–2135.
- Schett G. Effects of inflammatory and anti‐inflammatory cytokines on the bone. Eur J Clin Investig. 2011;41(12):1361–1366.
- Li M, Li Y, Healy D, et al. Alfacalcidol restores cancellous bone in ovariectomized rats. J Musculoskelet Neuronal Interactions. 2003;3(1):39–46.
- Shiraishi A, Higashi S, Ohkawa H, et al. The advantage of alfacalcidol over vitamin D in the treatment of osteoporosis. Calcified Tissue Int. 1999;65(4):311–316.
- Butcher C, Marsh D. Nonsteroidal anti-inflammatory drugs delay tibial fracture union. Injury. 1996;27(5):375–375.
- Bellows C, Reimers S, Heersche J. Expression of mRNAs for type-I collagen, bone sialoprotein, osteocalcin, and osteopontin at different stages of osteoblastic differentiation and their regulation by 1, 25 dihydroxyvitamin D3. Cell Tissue Res. 1999;297(2):249–259.