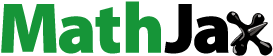
Abstract
In this study, the functional polymer biocarrier polyamide 6 (PA6) modified by anthraquinone-2-sulphonate (AQS) was synthesized by hydrolysis and sulphonation reactions. The optimal hydrolysis conditions of PA6 were 35 °C, 3 mol/L HCl and 72 h reaction time. The optimal sulphonation conditions of PA6 modified by AQS (PA6-AQS) were 30 °C and 6 h reaction time. The morphology and heat resistance of PA6-AQS were analysed by scanning electron microscopy, thermogravimetric analysis and differential scanning calorimetry. The AQS content of PA6-AQS, determined by an elemental analysis, was approximately 0.11 mmol/g. The denitrification efficiency was enhanced by 1.78-fold with PA6-AQS. Repeated-batch operations showed that the denitrification efficiency with PA6-AQS became stabilized above 80% of the original value and exhibited a better catalytic activity and durability.
Introduction
Functional polymers are introduced centuries ago and their characteristics are identified by the aggregate structure, chain structure and species, quantity, and distribution of functional groups on the chain. Functional polymers can meet special requirements, such as photosensitiveness,[Citation1,Citation2] electroconductibility,[Citation3,Citation4] select classification [Citation5,Citation6] and slow-release feature.[Citation7–9] Therefore, functional polymers can be applied on various fields, including wastewater treatment.
It is known that functional polymers serve a role as flocculants,[Citation10] ion exchange resins [Citation11] and ionic membranes [Citation12] on the physical/chemical treatment process of hexavalent chromium,[Citation11] ceric ion,[Citation13] azo dyes,[Citation14] nitrite, nitrate [Citation15] and cobalt.[Citation16] Meanwhile, functional polymers act as biocarriers and play an important role in biofilm processes, which is one of the important biological treatment technologies. Jurecska et al. [Citation17] studied four carriers, containing three types of polypropylene fibres and polyester fibre. It was shown that functional polymers were worth applying in further experiments in the industrial wastewater biological treatment. Recently, redox mediators immobilized on functional polymers, forming new functional polymer biocarriers, have become a research emphasis. A redox mediator, for example, the quinone redox mediator, serves as an electron donor to accelerate the microbial reduction.[Citation18] New functional polymer biocarriers are conducive to microorganism growth on the surface and accelerate the biotransformation of contaminants. However, the reported functional polymer biocarriers were not ideal for application and this is why novel functional polymer biocarriers need to be explored.
In this study, anthraquinone-2-sulphonate (AQS) was selected as the redox mediator, and polyamide 6 (PA6) was selected as the functional polymer, thanks to its good biocompatibility, stable chemical performance and large specific surface area. The functional polymer biocarrier PA6 modified by AQS (PA6-AQS) was synthesized, optimized and characterized. The catalysing effect and stability of PA6-AQS on denitrification were also studied.
Materials and methods
Materials
PA6 was obtained from Shijiazhuang Hengjia Co., Ltd. Acid red 14 (AR14) was the sulphonated azo dye used in this study and was purchased from Xiandai Ltd. Anthraquinone-2-sulphonic sodium (AQS) was purchased from Shanghai Yuan Ye Biotechnology Co., Ltd. Anthraquinone-2-sulphonyl chloride (ASC) was synthesized by using AQS.[Citation19] All of the other reagents used were of analytical grade. The dye solution was prepared by dissolving AR14 in distilled water until it reached a concentration of 150 mg/L.
Strains, media and culture conditions
The reaction temperature and time of PA6-AQS' synthesis were optimized by the decolourization efficiency, which was related to the quinone group content and the application of PA6-AQS was evaluated by denitrification. The decolourization/denitrification efficiency (η) was calculated by EquationEquation (1)(1)
(1) . C0 and Ct are the concentrations of AR14/nitrate at initial time and time t. Halomonas sp. GYW (GenBank accession number, EF188281) and Paracoccus versutus strain GW1 (GenBank accession number, GU111570) were obtained from the Hebei Key Laboratory of Environmental Biotechnology and kept at 4 °C. The culture conditions of GYW and GW1 were in a good agreement with the reported cultivation conditions in the literature.[Citation20] The denitrification experiment was conducted at an initial nitrate concentration of 160 mg/L. The GYW/GW1, AR14/nitrate and PA6 were set as blank controls in the decolourization/denitrification experiment. The PA6-AQS denitrification experiment was repeated six times.
(1)
(1)
Preparation of PA6-AQS
PA6-AQS was obtained by a two-step procedure: hydrolysis and sulphonation reaction. An acid amide bond in PA6 was produced by polymerization with a ring-opening reaction of ϵ-caprolactam.[Citation21] The hydrolysis product of PA6 was ϵ-caprolactam, which underwent an intramolecular back-biting process and a hydrogen transfer reaction, leading to the scission of the C–N bond β to the amide group.[Citation22] As shown in Equation (2), PA6 reacted with HCl. Many amino functional groups were exposed in this process. The hydrolysate was PA6-N, which was rinsed with distilled water three times at room temperature. The HCl concentration, reaction temperature and reaction time were the main factors in the hydrolysis and were optimized by an orthogonal experiment. The hydrolysis conditions were optimized by using the orthogonal experiment of three factors and four levels (). The three factors were HCl concentration (factor A), hydrolysis temperature (factor B) and hydrolysis time (factor C). The mean values of K (Kji) and the range value (Rj) were calculated by the mathematical calculation of the orthogonal experiment in
Table 1. Levels and factors affecting the content of the amino groups.
Table 2. Hydrolysis of PA6 in orthogonal experiment and range analysis data of the amino group content.
As shown in Equation (3), HCl was produced during the synthesis process of PA6-AQS. In accordance with the theory of chemical equilibrium, PA6-AQS was easily synthesized under alkaline conditions, because HCl was commendably neutralized in the reaction system.
The sulphonation process was as follows: PA6-N was put into 50 mL of 2 mol/L NaOH. Meanwhile, 0.33 mmol ASC was dissolved in 30 mL dimethyl sulphoxide and was added in a dropwise fashion over a 30 min period. PA6-N reacted with ASC under different conditions and then the sulphonation product (PA6-AQS) was washed with distilled water three times at room temperature. Then, PA6-AQS was dried at 60 °C and used in the following decolourization and denitrification experiments.
The reaction conditions were optimized by the decolourization efficiency, which was related to the quinone group content. The reaction time was set at 2, 4, 6, 8 and 10 h. The reaction temperature was set at 20, 30 and 40 °C. The reaction time and reaction temperature were optimized by single variable analysis in the PA6-AQS synthesis.
Analytical methods
The morphologies of PA6 and PA6-AQS were examined by scanning electron microscopy (SEM, KYKY-AMRAY-1000B, China). The hydrolysing amine concentration of PA6-N was examined by the ninhydrin colouration method.[Citation23] The elemental composition and sulphur element concentration of PA6-AQS were gained by an elemental analysis instrument (Elementar Vario EL III, Germany). The sulphur difference value (S%) between PA6-AQS and PA6-N, and the quinone content of PA6-AQS were also calculated by this method.
The thermal analyses of the obtained products were characterized by thermogravimetric analysis (TGA, STARe, Mettler Toledo) and differential scanning calorimetry (DSC, Q-200), which was calibrated with indium. A stream of nitrogen was used as an inert atmosphere and the temperature was 20 °C for 120 s and then increased to 500 °C at a rate of 10 °C/min during the TGA experiment. The weight loss (mg) of PA6, PA6-N and PA6-AQS was calculated. PA6 and PA6-AQS samples were heated from 35 to 100 °C and then cooled at a rate of 10 °C/min in the DSC experiment. The heat flow (w/g) was examined before the experiment. The glass transition temperature (Tg) was obtained in the DSC experiment, based on the turning points of the heat flow curves of PA6, PA6-N and PA6-AQS.
The azo dye concentration was determined by UV-visible spectrophotometry (Tianmei, UV-2600, China) according to standard methods.[Citation24] Duplicates of all of the experiments were performed to ensure reproducibility and the results were expressed as the mean of these determinations.
Results and discussion
The orthogonal experiment of hydrolysis
The purchased PA6, which has few terminal amino groups, was used in the experiment. Therefore, the hydrolysis reaction was conducted to increase the amino groups in PA6 by rupturing the amide bond.
According to the orthogonal experiment in , 16 experiments were carried out to complete the optimization process. The concentration of the amino group is shown in . The entire amino group content increased during the hydrolysis process of PA6. For the sake of mechanical strength, it was not desirable for the surface of PA6 to be apparently shedding. The highest concentration of the amino group for each level was distinguished at 3 mol/L HCl, 35 °C and 72 h of PA6-N, which was the optimal combination of A3B2C4.
The mean values of K for different factors at different levels in the range analysis are shown in . For each factor, a higher mean value K indicated that the level had a larger effect on the amino group content and the range value (Rj) indicated the significance of the factor's effect. Compared with the range values of different factors (Rj), the significance of the factors' levels was as follows: reaction temperature > HCl concentration > reaction time.
The synthesis optimization of PA6-AQS
Optimization of the reaction time
According to the previous experimental steps, PA6-N reacted with 0.33 mmol ASC. The adsorption of PA6 on AR14 was below 0.01% (w/w) and negligible at 150 mg/L AR14 (data not shown).
As shown in A), the decolourization efficiencies were 47.23% (PA6-AQS-2 h), 63.07% (PA6-AQS-4 h), 76.38% (PA6-AQS-6 h), 47.96% (PA6-AQS-8 h) and 41.90% (PA6-AQS-10 h) at 4 h decolourization. All of the PA6-AQS with different reaction times accelerated the decolourization efficiency of AR14 and PA6-AQS-6 h had the highest decolourization efficiency. The reaction time affected the reaction degree of PA6-N and ASC. The structure of PA6 is linear and mutually entangled, leading to the increase of steric hindrance, which is unfavourable to low molecular reagents diffusion and reaction, although the amino functional groups were at the end of the macromolecular chain. Therefore, the reaction time of PA6-AQS was longer than that of small molecules.[Citation25] The reaction time was too short, as PA6 reacted with ASC insufficiently, resulting in a low decolourization efficiency. ASC was highly alkaline and hydrolysed to form sulphonate, when the reaction time was too long, and the decolourization efficiency was not obviously enhanced. Therefore, the optimal reaction time was 6 h.
Optimization of the reaction temperature
The reaction time was set at 6 h. As shown in B), the decolourization efficiency, with and without PA6, was similar. The decolourization efficiencies were 72.23% (PA6-AQS-20 °C), 83.13% (PA6-AQS-30 °C) and 65.79% (PA6-AQS-40 °C) at 4 h decolourization. All PA6-AQS with different reaction temperatures accelerated the decolourization efficiency of AR14 and the optimal reaction temperature was 30 °C. The temperature dependence of the molecular motion may be an important factor.[Citation26] When enhancing the temperature, the thermal energy, volume expansion, intermolecular distance and activity space for molecular motion were increased to help the diffusion and reaction of the low molecular reagent. However, the reaction temperature should not be too high, because it may cause an exothermic reaction of the sulphonation process. High temperatures not only hamper the positive reactions, but also shed the surface of PA6. Therefore, the introduction of quinone was influenced.
Based on the above analysis, the optimal preparation of PA6-AQS was 6 h reaction time and 30 °C reaction temperature.
Characterization of PA6-AQS
Elemental analysis of PA6-AQS
The PA6-N polymer molecule has nitrogen without sulphur, whereas PA6-AQS has a sulphur element. To quantify the quinone content of PA6-AQS, an elemental analysis was carried out. The sulphur difference value between the PA6-AQS and PA6-N was 0.352% and the quinone content of PA6-AQS was 0.11 mmol/g.
Scanning electron microscopy (SEM) of PA6-AQS
Some small homogeneous pore structures appeared on PA6, indicating that the homogeneous pore structure and the bulk properties of PA6-AQS were obviously not damaged during the quinone modification process. Therefore, PA6-AQS was beneficial to the subsequent application. The spectrograms of PA6 and PA6-AQS are shown in
Thermogravimetric analysis (TGA)
TGA was used to determine the weight loss of PA6, PA6-N and PA6-AQS. TGA is a well-established method in the determination of the weight loss characteristics.[Citation27]
The TGA of PA6, PA6-N and PA6-AQS is shown on A). The thermal degradation of PA6 occurred in one step, which was in good agreement with the reported results in the literature.[Citation28,Citation29] The weight loss of PA6, PA6-N, and PA6-AQS started at 384.21, 376.32 and 384.22 °C, respectively. The results showed that the heat resistance performance of PA6-AQS was unchanged by the chemical modification.
Differential scanning calorimetry (DSC)
The glass transition temperature (Tg) is one of the most important parameters for polymers' differentiation.[Citation30] Tg depends on the heating rate of the experiment,[Citation31,Citation32] the thermal history [Citation33,Citation34] and the molecular parameter of the sample, affecting the chain mobility.[Citation35] B) shows that the Tg of PA6, PA6-N and PA6-AQS was 74.88, 78.12 and 74.30 °C, respectively. The results showed that hydrolysis and sulphonation did not destroy the original structure of PA6 and PA6-AQS was appropriate for practical application.
Application of PA6-AQS for denitrification
The results from the test of PA6-AQS effect on denitrification are shown in A). After 10 h of the denitrification process, the nitrate concentration with PA6 and PA6-AQS (with 0.0136 mmol AQS) was 84.03 and 22.59 mg/L, respectively, and the denitrification efficiency was enhanced by 1.78-fold with PA6-AQS.
Figure 4. Application of PA6-AQS for denitrification. Effect of PA6-AQS on the denitrification efficiency (A). Repeated usage of PA6-AQS for denitrification (B).
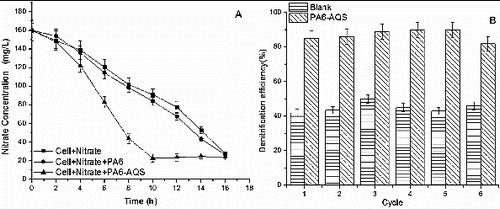
In addition, Xu et al. [Citation36] studied that polyethylene terephthalate modified by 1,8-dichloroanthraquinone (PET-1,8-DCA) was the functional biocarrier for accelerating denitrification with 0.14 mmol anthraquinone and the denitrification efficiency was enhanced by 1.49-fold. Liu et al. [Citation20] studied that the denitrification efficiency increased by nearly 1.5-fold with active carbon felt/electropolymerization of polypyrrole (PPy)/AQS (0.012 mmol) by electropolymerization, when compared to the control. Guo et al. [Citation37] studied that anthraquinone was immobilized by entrapment in calcium alginate. The result suggested that the artificial redox mediator (1.13 mmol anthraquinone) was found to be capable of enhancing the denitrification rate by about twofold. Compared with the above experiments, the synthetic method was easy to implement and synthetize, and the dosage of the redox mediator was less in the research.
An experiment for PA6-AQS' repeated usage for denitrification was also studied. After six cycles, the denitrification efficiency became stabilized above 80%, which showed that PA6-AQS exhibited good stability and reusability (B)). This result also suggested that the redox mediator on PA6 was recycled during the transfer of electrons from an electron donor to the terminal electron acceptor and was good for practical application.
Conclusions
The research presented here focused on the preparation and application of a novel polymer biocarrier of PA6-AQS. The study showed the optimization of the synthesis conditions by the use of azo dye decolourization efficiency, as a catalysing efficiency index. SEM images, DSC and TGA showed that the structure of PA6-AQS surface was distributed homogeneously and was not obviously damaged. Moreover, the denitrification efficiency was enhanced by 1.78-fold, compared with the control under optimal conditions and PA6-AQS exhibited a good catalytic performance. Consequently, PA6-AQS has potential applications for wastewater treatment, as a functional biocarrier.
Disclosure statement
No potential conflict of interest was reported by the authors.
Additional information
Funding
References
- Lai C, Guo W, Tang X, et al. Cross-linking conducting polythiophene with yellow-green light-emitting properties and good thermal stability via free radical polymerization and electropolymerization. Synth Met. 2011;161(17–18):1886–1891.
- Viswanath R, Naik H, Kumar G, et al. Synthesis and photoluminescence enhancement of PVA capped Mn2+ doped ZnS nanoparticles and observation of tunable dual emission: A new approach. Appl Surf Sci. 2014;301:126–133.
- Chan K, Yamao T, Kotaki M, et al. Unique structural features and electrical properties of electrospun conjugated polymer poly(3-hexylthiophene) (P3HT) fibers. Synth Met. 2010;160(23–24):2587–2595.
- Yıldırım M, Kaya İ. Synthesis and characterization of a novel kind soluble, conjugated, and fluorescent chelate polymer containing fluorene ring in the backbone: optical, electrical and electrochemical properties. Synth Met. 2011;161(1–2):13–22.
- He D, Susanto H, Ulbricht M. Photo-irradiation for preparation, modification and stimulation of polymeric membranes. Prog Polym Sci. 2009;34(1):62–98.
- Jurecska L, Barkács K, Kiss É, et al. Intensification of wastewater treatment with polymer fiber-based biofilm carriers. Microchem J. 2013;107:108–114.
- Szabó T, Mihály J, Sajó I, et al. One-pot synthesis of gelatin-based, slow-release polymer microparticles containing silver nanoparticles and their application in anti-fouling paint. Prog Org Coat. 2014;77(7):1226–1232.
- Shao Y, Li L, Gu X, et al. Evaluation of chitosan–anionic polymers based tablets for extended-release of highly water-soluble drugs. Asian J Pharm Sci. 2014;10:1–7.
- Chu D, Curdy C, Riebesehl B, et al. In situ forming parenteral depot systems based on poly (ethylene carbonate): effect of polymer molecular weight on model protein release. Eur J Pharm Biopharm. 2013;85(3):1245–1249.
- Yang Z, Liu X, Gao B, et al. Flocculation kinetics and floc characteristics of dye wastewater by polyferric chloride–poly-epichlorohydrin–dimethylamine composite flocculant. Sep Purif Technol. 2013;118:583–590.
- Alvarado L, Torres I, Chen A. Integration of ion exchange and electrodeionization as a new approach for the continuous treatment of hexavalent chromium wastewater. Sep Purif Technol. 2013;105:55–62.
- Panigrahi A, Pilli S, Mohanty K. Selective separation of bisphenol A from aqueous solution using supported ionic liquid membrane. Sep Purif Technol. 2013;107:70–78.
- Bharti S, Mishra S, Sen G. Ceric ion initiated synthesis of polyacrylamide grafted oatmeal: its application as flocculant for wastewater treatment. Carbohydr Polym. 2013;93(2):528–536.
- Fang R, Cheng X, Xu X. Synthesis of lignin-base cationic flocculant and its application in removing anionic azo-dyes from simulated wastewater. Bioresour Technol. 2010;101(19):7323–7329.
- Mishra S, Mukul A, Sen G, et al. Microwave assisted synthesis of polyacrylamide grafted starch (St-g-PAM) and its applicability as flocculant for water treatment. Int J Biol Macromol. 2011;48(1):106–111.
- Rengaraj S, Moon S. Kinetics of adsorption of Co (II) removal from water and wastewater by ion exchange resins. Water Res. 2002;36(7):1783–1793.
- Jurecska L, Barkács K, Kiss É, et al. Intensification of wastewater treatment with polymer fiber-based biofilm carriers. Microchem J. 2013;107:108–114.
- Van Der Zee F, Bisschops I, Lettinga G, et al. Activated carbon as an electron acceptor and redox mediator during the anaerobic biotransformation of azo dyes. Environ Sci Technol. 2003;37(2):402–410.
- Feng F, Uno B, Goto M, et al. Anthraquinone-2-sulfonyl chloride: a new versatile derivatization reagent—synthesis mechanism and application for analysis of amines. Talanta. 2002;57(3):481–490.
- Liu H, Guo J, Qu J, et al. Biological catalyzed denitrification by a functional electropolymerization biocarrier modified by redox mediator. Bioresour Technol. 2012;107:144–150.
- Iwaya T, Sasaki M, Goto M. Kinetic analysis for hydrothermal depolymerization of nylon 6. Polym Degrad Stab. 2006;91(9):1989–1995.
- Kiziltas A, Gardner D, Han Y, et al. Dynamic mechanical behavior and thermal properties of microcrystalline cellulose (MCC)-filled nylon 6 composites. Thermochim Acta. 2011;519(1–2):38–43.
- Szegedi A, Popova M, Goshev I, et al. Controlled drug release on amine functionalized spherical MCM-41. J Solid State Chem. 2012;194:257–263.
- American Public Health Association. Standard methods for the examination of water and wastewater. 20th ed. Washington (DC): American Public Health Association, American Water Works Association, Water Environment Federation; 1992.
- Gamedze M, Maseko R, Chigondo F, et al. Serendipitous synthesis of 3-hydroxy tetrahydrofurans from tin catalyzed sulfonylation of acyclic 1,2,4-triols. Tetrahedron Lett. 2012;53(44):5929–5932.
- Wolińska-Grabczyk A, Bednarski W, Jankowski A, et al. Temperature dependence of molecular motions in the polyurethane-based membranes studied with paramagnetic spin probe. Polymer. 2005;46(8):2461–2471.
- Miranda M, Bica C, Nachtigall S, et al. Kinetical thermal degradation study of maize straw and soybean hull celluloses by simultaneous DSC–TGA and MDSC techniques. Thermochim Acta. 2013;565:65–71.
- Kusmono, Ishak Z, Chow W, et al. Influence of SEBS-g-MA on morphology, mechanical, and thermal properties of PA6/PP/organoclay nanocomposites. Eur Polym J. 2008;44(4):1023–1039.
- Scaffaro R, Botta L, Frache A, et al. Thermo-oxidative ageing of an organo-modified clay and effects on the properties of PA6 based nanocomposites. Thermochim Acta. 2013;552:37–45.
- Mizuno M, Pikal M. Is the pre-Tg DSC endotherm observed with solid state proteins associated with the protein internal dynamics Investigation of bovine serum albumin by solid state hydrogen/deuterium exchange. Eur J Pharm Biopharm. 2013;85(2):170–176.
- Kousksou T, Jamil A, Omari K, et al. Effect of heating rate and sample geometry on the apparent specific heat capacity: DSC applications. Thermochim Acta. 2011;519(1–2):59–64.
- Chataing G, Chater M, Vergnaud J. Effect of heating rate in DSC on heat flux-time curves and profiles of temperature and state of cure developed in the sample. Thermochim Acta. 1986;97:281–286.
- Li J, Bao R, Yang W, et al. Effect of annealing temperature on the mechanical properties, thermal behavior and morphology of β-iPP/PA6 blends. Mater Des. 2012;40:392–399.
- Cartledge H, Baillie C, Mai Y. Friction and wear mechanisms of a thermoplastic composite GF/PA6 subjected to different thermal histories. Wear. 1996;194(1–2):178–184.
- Baird J, Taylor L. Evaluation of amorphous solid dispersion properties using thermal analysis techniques. Adv Drug Deliv Rev. 2012;64(5):396–421.
- Xu Q, Guo J, Niu C, et al. The denitrification characteristics of novel functional biocarriers immobilised by non-dissolved redox mediators. Biochem Eng J. 2015;95:98–103.
- Guo J, Kang L, Yang J, et al. Study on a novel non-dissolved redox mediator catalyzing biological denitrification (RMBDN) technology. Bioresour Technol. 2010;101(11):4238–4241.