Abstract
The aim of our study was to evaluate the influence of selective non-steroidal anti-inflammatory drug meloxicam and its metal (Cu(II), Zn(II), Co(II), Ni(II)) complexes on the viability and proliferation of cultured humancarcinoma of the uterine cervix (HeLa) and glioblastoma multiforme (8MGBA) cells. The investigations were performed by short-term (24 h–96 h, with monolayer cultures) and long-term (16 d, with three-dimensional colonies of cancer cells) experiments by using methods with different molecular/cellular targets and mechanisms of action, such as thiazolyl blue tetrazolium bromide (MTT) test, neutral red uptake cytotoxicity assay, crystal violet staining, double staining with acridine orange and propidium iodide, alkaline version of single cell gel electrophoresis (comet assay) and colony-forming technique. The obtained results revealed that the application of the examined compounds at concentrations ranging from 5 µg/mL to 500 µg/mL induced cytopathological changes, including DNA damages in the treated cells and a significant decrease of their viability and proliferation in a time- and concentration-dependent manner. Metal complexes were found to have a more pronounced cytotoxic/cytostatic effect, when compared to their ligand meloxicam.
Introduction
Non-steroidal anti-inflammatory drugs (NSAIDs) are among the most commonly prescribed medications in the world due to their remarkable ability to reduce pain and inflammation and also because of their antipyretic effect.[Citation1,Citation2] NSAIDs have been successfully used in the treatment of various clinical disorders, including osteoarthritis, rheumatoid arthritis, ankylosing spondylitis,[Citation3,Citation4] gout,[Citation5] dental pain [Citation6] and headache,[Citation7] in the gynaecologic practice,[Citation8,Citation9] etc.
The biological effects of NSAIDs are based mainly on inhibition of the enzyme cyclooxygenase (COX, also referred to as prostaglandin synthase), blocking the production of prostaglandins. The isoforms of COX – COX-1 and COX-2 – are under distinct regulatory control mechanisms. COX-1 is constitutively expressed in most tissues, and plays an important role in normal functioning of the gastrointestinal tract, kidneys and platelets. In contrast, COX-2 is undetectable in most healthy tissues and can be induced by different factors, such as cytokines (interleukin-1β, tumour necrosis factor-α), growth factors (fibroblast growth factor, platelet-derived growth factor, epidermal growth factor), hormones (luteinizing hormone), oncogenes (v-src) and tumour promoters and, therefore, contributes to the synthesis of prostaglandins in inflamed and malignant tissues.[Citation10,Citation11]
In recent years, there has been an increased interest in the antitumour activity of NSAIDs due to at least three reasons: (1) COX-2 is found to be overexpressed in many cancers thus making it an attractive therapeutic target for the prevention and treatment of a number of malignancies [Citation11,Citation12]; (2) chronic inflammation has been proved to play an important role in cancer development and progression [Citation13,Citation14]; (3) NSAIDs are used also by oncology patients and the ‘relationships’ between these drugs and cancer cells have to be better clarified.[Citation15]
Numerous studies have suggested that aspirin and/or other NSAIDs can be effective in the prevention and/or treatment of malignancies, such as cancers of the colon,[Citation16] breast,[Citation17,Citation18] lung,[Citation19] pancreas,[Citation20] prostate,[Citation21,Citation22] ovarium,[Citation23] squamous cell carcinoma and melanoma,[Citation24] etc. Different NSAIDs have been reported to inhibit the growth and survival of cell lines established from a wide variety of cancers, including gastric cancer,[Citation25,Citation26] cancers of the colon,[Citation27] lung,[Citation28] urinary bladder,[Citation29] osteosarcoma,[Citation30] etc.
The group of NSAIDs comprise both of traditional nonselective NSAIDs that non-specifically inhibit COX-1 and COX-2 and selective COX-2 inhibitors.[Citation31] As COX-1 is responsible for maintaining normal biological functions, the selective NSAIDs deserve special attention, because they are expected to exhibit less harmful side effects.[Citation10,Citation11]
In our previous investigation we found that the selective NSAID meloxicam and its metal (Cu(II), Zn(II), Co(II), Ni(II)) complexes significantly inhibit the in vitro growth of retrovirus-transformed chicken hepatoma (LSCC-SF-Mc29) and rat sarcoma (LSR-SF-SR) cells.[Citation32] The aim of the present study was to evaluate the influence of the same compounds on the viability and proliferation of cultured human glioblastoma multiforme and uterine cervical carcinoma cells.
Materials and methods
Materials and supplies
Cobalt acetate tetrahydrate (Co(CH3COO)2∙4H2O), copper acetate monohydrate (Cu(CH3COO)2∙H2O), zinc acetate dihydrate (Zn(CH3COO)2∙2H2O) and methanol were purchased from Merck (Germany). Nickel acetate tetrahydrate (Ni(CH3COO)2∙4H2O) and meloxicam were from Alfa Aesar (Germany). Dulbecco's modified Eagle's medium (DMEM) and fetal bovine serum were obtained from Gibco-Invitrogen (UK). Purified agar, thiazolyl blue tetrazolium bromide (MTT), 3-Amino-7-dimethylamino-2-methylphenazine hydrochloride (neutral red), Tris[4-(dimethylamino)phenyl]methylium chloride (crystal violet), 3,6-acridinediamine, N,N,N',N'-tetramethyl-, monohydrochloride (acridine orange), propidium iodide and agarose (normal and low gelling) were purchased from Sigma-Aldrich Chemie GmbH (Germany). Dimethyl sulfoxide (DMSO) and trypsin were obtained from AppliChem (Germany). The antibiotics (Penicillin and Streptomycin) for cell cultures were obtained from Lonza (Belgium). Ethylenediaminetetraacetic acid (EDTA) and all other chemicals of the highest purity commercially available were purchased from local agents and distributors. All sterile plasticware and syringe filters were from Orange Scientific (Belgium).
Synthesis of the complexes
The complexes were prepared according to a previously reported procedure.[Citation32] Typically, a hot methanol solution of the respective metal acetate (Cu(II), Zn(II), Co(II), Ni(II)) (5 mL, 1 mmol/L) was added to hot methanol solution of deprotonated meloxicam (50 mL, 2 mmol/L). The resulting mixture was refluxed under stirring for approximately 0.5 h. The complexes were precipitated as microcrystalline powders. The solid compounds were filtered, washed with hot methanol and then dried in desiccator under P4O10 at a room temperature.
The selective NSAID meloxicam () and its metal (Cu(II), Zn(II), Co(II), Ni(II)) complexes, investigated in our study, are presented in
Table 1. Meloxicam and its metal complexes investigated in the present study.
The compounds were dissolved in DMSO and then diluted in culture medium. The final concentrations of DMSO in the solutions of the tested compounds are presented in
Table 2. Concentrations of the compounds (meloxicam and its metal complexes) and their solvent (DMSO) tested in the study.
Cell cultures and cultivation
Human permanent cell lines established from glioblastoma multiforme – 8MGBA [Citation33] and uterine cervical carcinoma – HeLa,[Citation34] were used as model systems in our study. The cell lines were obtained from Cell Culture Collection of the Institute of Experimental Morphology, Pathology and Anthropology with Museum -- Bulgarian Academy of Sciences.
The cell cultures were grown in DMEM medium supplemented with 5%–10% fetal bovine serum, 100 U/mL penicillin and 100 μg/mL streptomycin. The cultures were kept in a humidified incubator (Thermo Scientific, HEPA Class 100) at 37 °C under 5% CO2 in the air. For routine passages adherent cells were detached using a mixture of 0.05% trypsin and 0.02% EDTA. The cell lines were passaged 2–3 times per week (1:2 to 1:3 split). The experiments were performed during the exponential phase of cell growth.
Cytotoxicity assays
The cells were seeded in 96-well flat-bottomed microplates at a concentration of 1 × 104 cells/well. At the 24th hour the culture medium was removed and changed with DMEM medium containing different concentrations of the tested compounds. Each concentration was applied in six to eight wells. Samples of the cells grown in non-modified medium (control culture medium) and in medium containing the corresponding amount of DMSO (DMSO-control) () served as controls. The effect of the compounds on cell viability / proliferation was assessed using MTT test, neutral red uptake cytotoxicity assay (NRCA) and crystal violet staining (CVS) after 24 h, 48 h,72 h and 96 h of incubation.
The MTT assay of cell viability was performed in accordance with Mossmann's procedure.[Citation35] The cells were incubated for 3 h with MTT solution (5 mg MTT in 10 mL DMEM) at 37 °C under 5% CO2 atmosphere. The formed blue MTT formazan was extracted with a mixture of absolute ethanol and DMSO (1:1, v/v).
The NRCA was based on the method of Borenfreund and Puerner.[Citation36] A solution of NR (50 μg/mL, 0.1 mL) was added to each well containing cells. The plates were placed in CO2 incubator for 3 h to ensure the uptake of vital dye. Next, the NR-containing medium was removed and the cells were washed with phosphate-buffered saline (PBS, pH 7.2; 0.2 mL/well). The addition of 0.1 mL 1% (v/v) acetic acid solution containing 50% ethanol extracted the dye from the viable cells.
CVS was performed as described by Saotome et al. [Citation37] The microplate with tested compounds was incubated for 72 h at 37 °C in 5% CO2 atmosphere. After this period, the medium was removed and the cells were stained with 0.4% (w/v) crystal violet solution in methanol for 30 min and carefully washed with distilled water.
The final step of cytotoxicity assays – quantitative analysis, was performed by absorbance measurements in an automatic microplate reader at (Tecan, Sunrise™, Austria) at 540/620 nm (for MTT and CVS) and 540 nm (for NRCA). Cell viability, expressed as a percentage of the corresponding DMSO-control (), was calculated for each concentration of meloxicam and its metal complexes. were prepared and the effective concentrations of the compounds – CC50 (causing a 50% reduction of cell viability, as compared to culture medium control, in µmol/L) and/or CC90 (causing a 90% reduction of cell viability, as compared to culture medium control, in µmol/L) were estimated, where possible. All data points represent an average of three independent assays.
Double staining with acridine orange (AO) and propidium iodide (PI)
The ability of compounds (applied at concentrations of 250 µg/mL and 500 µg/mL) to induce cytopathological changes was assessed using double staining with AO and PI according to the standard procedures.[Citation38] The cells were grown on cover slips in 6-well plates in the presence of the tested compounds. Non-treated cells (control culture medium) and cells treated with 1% and 2% DMSO (as described in , DMSO-control) served as controls. After 72 h of incubation, the coverslips were removed and washed with PBS for 2 min. Equal volumes of fluorescent dyes containing AO (10 µg/mL in PBS) and PI (10 µg/mL in bi-distilled water) were added to the cells. Fresh stained cells were placed on a glass slide and examined under a fluorescence microscope (Leica DM 500B, Wetzlar, Germany) within 30 min before the fluorescent colour started to fade.
Single-cell gel electrophoresis (alkaline comet assay)
The HeLa cells treated with Cu-Mel, Zn-Mel, Co-Mel and Ni-Mel as well as control cells (treated only with 2% DMSO) were used for the comet assay analyses. The alkaline version of the method of comet assay, published by Olive et al.,[Citation39] was used in our study in order to examine the genotoxic effect of the tested compounds. The compounds were applied at a concentration of 500 µg/mL. Control cells were cultured in a medium containing 2% DMSO.
The control and treated cells were mixed with 1.4% of low-gelling agarose (Sigma type II) and immediately spread onto microscopic slides, preliminary covered with 0.5% (w/v) normal agarose. Cells were lysed for 1 h in a lysis solution (1 mol/L NaCl, 50 mmol/L EDTA pH 8, 30 mmol/L NaOH, 0.1% (w/v) N-lauroylsarcosine; pH 10). After 1 h of incubation in the denaturing solution (30 mmol/L NaOH, 10 mmol/L EDTA; pH 12.6) for DNA unwinding the slides were electrophoresed for 20 min at 0.46 V/cm in the same denaturing buffer (30 mmol/L NaOH, 10 mmol/L EDTA; pH 12.6). At the end of the electrophoresis the slides were subsequently dehydrated for 5 min in 75% and in 96% of ethanol. Comets were observed under Leitz epifluorescent microscope (Orthoplan, Vario Orthomat 2) using a 450–490 nm band-pass filter following staining of microgels with the fluorescent dye SYBR Green I (Molecular Probes). Pictures were taken with a digital photo camera, Olympus μ8, at a resolution of 3 MP. 200 randomly chosen comets per each probe and treatment were taken for quantification. The presented parameter ‘Comet length’ was measured automatically by the software CometScore, whereas the percentage of comets was determined by a manual scoring.
Colony forming method
The influence of the compounds tested on the anchorage-independent growth of cancer cells was investigated by colony-forming method. Tumour cells (103 cells/well) suspended in 0.45% (w/v) purified agar in DMEM medium containing different concentrations of meloxicam and its metal complexes (ranging from 5 μg/mL to 500 μg/mL) were layered in 24-well microplates. Non-treated cells (culture medium control) and cells treated with DMSO (at concentrations described in , DMSO-control) served as controls. The presence / absence of colonies and their number (counted in at least 4–5 different fields of each well) were registered using an inverted microscope (Carl Zeiss, Germany) during a period of 16 d (counted on a five-day intervals).
Statistical analysis
The data were presented as mean ± standard error of the mean. Statistical differences between control and treated groups were assessed using one-way analysis of variance (ANOVA) followed by Dunnett's post-hoc test. The effective concentrations of the compounds – CC50 and/or CC90 were estimated by Origin 6.1™.
Results and discussion
The effect of meloxicam and its metal complexes on the viability and proliferation of cultured human 8MGBA and HeLa cells was evaluated by performing short (24 h–96 h, with monolayer cultures)-term and long (16 d, with three-dimensional (3D) colonies of cancer cells)-term experiments by using methods with different molecular / cellular targets and mechanisms of action.
Short-term experiments
The investigations were initiated by MTT test – the gold standard of viability / cytotoxicity assay methods,[Citation40] followed by simultaneous performance at equal conditions of MTT test, NRCA and CVS. Meloxicam and its metal (Cu(II) Zn(II), Co(II), Ni(II)) complexes were applied at the concentrations given in . Concentration-response curves were prepared by using the obtained experimental data. Some of them are presented in . The effective concentration of the compounds that reduce the amount of viable cells by 50% (CC50) and 90% (CC90), as compared to the control culture medium, were estimated from these curves ( and ) showing that metal complexes, especially those of Cu(II) and Zn(II), exhibited a higher cytotoxic/cytostatic activity, as compared to meloxicam. CC50 and CC90 were not calculated at 24 h and 48 h because >50% viable cells (as compared to the control culture medium) were found at all concentrations tested at these incubation periods.
Figure 2. Concentration-response curves of meloxicam and its metal (Cu(II), Zn(II), Co(II), Ni(II)) complexes against human 8MGBA and HeLa cancer cells evaluated by MTT test after a 96 h treatment period.
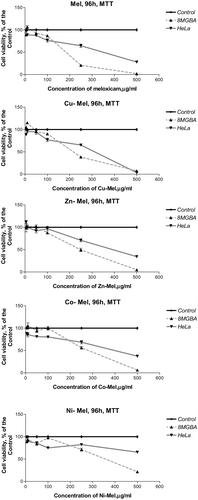
Table 3. Cytotoxicity (CC50, µmol/L) of meloxicam and its metal complexes against 8MGBA and HeLa human cancer cell lines.
Table 4. Cytotoxicity (CC90, µmol/L) of meloxicam and its metal complexes against human 8MGBA and HeLa cancer cell lines
Cytopathological changes were observed in cells treated by meloxicam and its metal complexes after double staining with AO and PI ( and ). No alterations were found in the corresponding DMSO-controls – 8MGBA and HeLa cells cultured in the presence of 1% and 2% DMSO, respectively, as compared to untreated cells (control culture medium).
Figure 3. A complete monolayer of 8MGBA human glioblastoma multiforme cells with bright yellow-green nucleoli and bright yellow-green granular accumulations within the cytoplasm – culture medium control (a); 8MGBA cells 72 h following the treatment with с 500 µg/mL meloxicam (b); 500 µg/mL Cu-Meloxicam (c); 500 µg/mL Zn-meloxicam (d); 500 µg/mL Co-meloxicam (e) and 500 µg/mL Ni-Meloxicam (f). Note: Significant cell losses as well as cell shrinkage with foamy vacuolation of the cytoplasm (b). Moderate cell losses (c, d and e) as well as chromatin condensation (d, e). Acridine orange – propidium iodide staining. Bar = 50 µm.
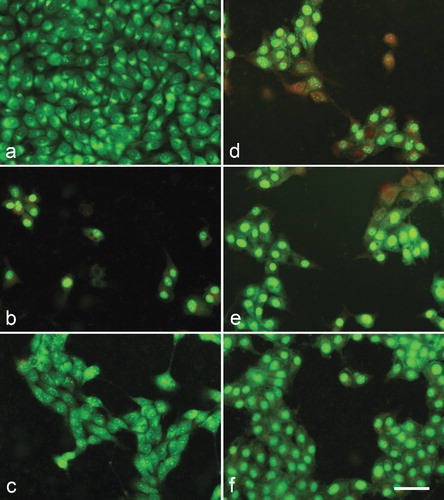
Figure 4. A complete monolayer of HeLa human cervical carcinoma cells with bright yellow-green nucleoli and bright yellow-green granular accumulations within the cytoplasm – culture medium control (a); HeLa cells 72 h following the treatment with с 500 µg/mL meloxicam (b); 500 µg/mL Cu-meloxicam (c); 500 µg/mL Zn-meloxicam (d); 500 µg/mL Co-meloxicam (e) and 500 µg/mL Ni-meloxicam (f). Note: Cell swelling with increasing of intercellular spaces (b); moderate cell losses (c), as well as appearing of dead cells (red nuclei) (c, d, e and f). Acridine orange – propidium iodide staining. Bar = 50 µm.
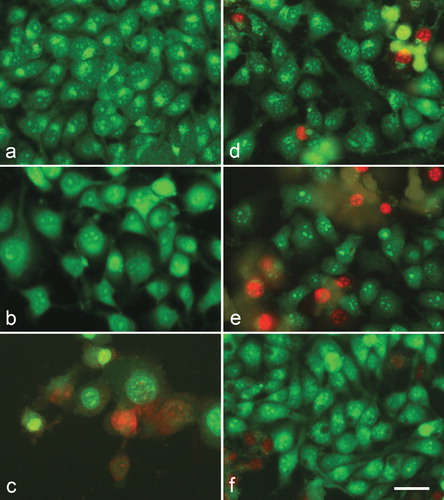
The results from the alkaline comet assay revealed that though meloxicam showed genotoxicity on the tested cells, its combination with Cu(II), Zn(II), Co(II) or Ni(II) induced increased genotoxicity on the studied cells. After automatic comet assay data quantitation by means of specialized software, named CometScore, we proved undoubtedly that the treatment of HeLa cells with all four metal complexes of meloxicam resulted in longer tail lengths of the observed comets (). The detailed analyses of the obtained comet lengths of the comets obtained after treatment with the studied substances revealed that the four used heavy metals showed almost similar genotoxicity. Interestingly, when compared to the control and to meloxicam, the four agents exhibited increased genotoxicity. The comparison between the mean tail lengths of the comets obtained with Cu-Mel, Zn-Mel, Co-Mel and Ni-Mel with that of the control (treated with 2% DMSO) cells showed more than 50% longer tails when compared to the meloxicam comets. We calculated more than 30% longer comet tail lengths (, ). The increased genotoxicity was also represented by higher percentage of dead cells and increased percentage of DNA damage, which can be seen from the . The table represents the percentage of comets assessed after manual scoring of the slides.
Table 5. Genotoxicity testing of meloxicam and its metal complexes by means of the method of comet assay.
Figure 5. Representative comet images obtained after treatment of HeLa cells with meloxicam and its metal complexes. Note: The compounds were applied at a concentration of 500 µg/mL. DMSO-control cells were cultured in a medium containing 2% DMSO.
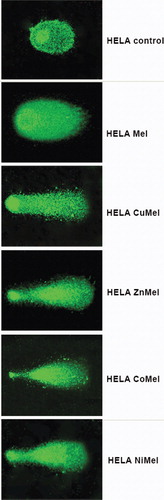
Table 6. Percentage of comets observed after treatment of HeLa cells with meloxicam and its metal complexes.
Long-term experiments
The influence of the tested compounds on the anchorage-independent growth of cancer cells was also investigated. The first colonies consisting of 8–10 HeLa cells were observed in culture medium controls and DMSO-controls on the 3rd day. The data presented in show that meloxicam and its 3d metal complexes inhibited in a concentration-dependent manner the colony-forming ability of HeLa cells. Applied at a concentration of 500 µg/mL (625 µmol/L) Cu(II) complex of meloxicam completely suppressed the 3D growth of cervical carcinoma cells in a semi-solid medium. The absence of cancer cell colonies was also registered in the wells treated by meloxicam, but at a higher concentration (500 µg/mL = 1424.5 µmol/L). Tested independently, DMSO administered at concentrations of 0.4% and 1% did not affect significantly the anchorage-independent growth of HeLa cells, as compared to the untreated controls (culture medium control). The number of 3D cell colonies was found to be diminished by ∼15.7% when DMSO was applied at the highest concentration examined (2%). This is the reason that in , only DMSO 2% is presented as DMSO control.
Table 7. Effect of meloxicam and its metal complexes on colony-forming ability of human HeLa cervical carcinoma cells.
NSAIDs are well known as anti-inflammatory, analgesic and antipyretic agents and have also been found to express anticancer effects.[Citation41] Our decision to evaluate the putative anticancer activity of meloxicam and its metal complexes is not occasional:
Meloxicam belongs to the group of selective cyclooxygenase-2 inhibitors. COX-2 is a key enzyme for converting arachidonic acids into prostaglandins and thromboxanes. The prostaglandins are known to be induced during inflammation and cancer initiation. Due to its crucial role in tumour development and progression, COX-2 has been one of the preferred targets for cancer reduction.[Citation11,Citation12,Citation42–44] Cyclooxygenase-2 is found to be overexpressed in many types of cancers, including glioblastoma multiforme [Citation45] and cervical cancer.[Citation46] Furthermore, increased COX-2 expression has been shown to directly correlate with glioma grade and to be associated with shorter survival in glioblastoma multiforme patients.[Citation47] In addition, there are data that COX-2 regulates the proliferation of glioma stem like cells.[Citation48]
Promising antitumour activity of meloxicam has been demonstrated in prostate [Citation49] and colorectal [Citation50] cancer cell lines, hepatocellular carcinoma [Citation51] and osteosarcoma.[Citation52] The performed investigations revealed that meloxicam can be useful for the treatment of liver metastasis of colorectal carcinoma [Citation53] and as a therapeutic agent in non-small cell lung cancer (NSCLC) [Citation54] and ovarian cancer.[Citation55] The potential clinical application of meloxicam, in combination with cytotoxic drugs in patients with COX-2-positive osteosarcoma, has also been discussed.[Citation56]
Meloxicam has been found to protect the heart against doxorubicin toxicity (without affecting its antitumour activity) in a solid form of Ehrlich mammary cancer model in mice.[Citation57] In addition, this selective NSAID has been proved to ameliorate doxorubicin-induced renal injury in mice via inhibition of inflammatory prostaglandin E2 (PGE2) and cytokines, caspase-3 activity. They also have, antioxidant effect, and free radical scavenging activity.[Citation58] These findings further strengthen the interest to biological activity and medicinal applications of meloxicam, because the prolonged use of NSAIDs is associated with gastrointestinal, renal and cardiovascular toxicity [Citation59] and specific COX-2 inhibitors are connected with side effects like myocardial infarction.[Citation44]
It is well known that linking a transition metal to a bioactive ligand may have synergistic effects leading to the enhancement of their biological potential, or decreasing the adverse effects of both metal ions and ligand. Coordination complexes could offer many advantages over the ligand or metal itself, like an intrinsic high biological activity, a better solubility in biological fluids and a superior transport process through the cell membranes.[Citation60,Citation61]
One of the most intriguing questions regarding biological activity of putative anticancer agents is their possible mechanism(s) of action. It could be assumed that the enzyme cyclooxygenase-2 is the main target of the selective NSAID meloxicam and (possibly) of its metal complexes. COX-2 has been proved to contribute to cancer development and progression through synthesis of prostaglandins, which stimulate specific receptors with subsequent involvement of different pathways, resulting in: inhibition of apoptosis, stimulation of angiogenesis, increased invasiveness, modulation of inflammation/immunosuppression and conversion of procarcinogens to carcinogens.[Citation42,Citation43] COX-independent targets have also been reported to be at least partially responsible for the antineoplastic activity of NSAIDs, including the inhibition of cyclic guanosine monophosphate phosphodiesterases, generation of reactive oxygen species, suppression of the apoptosis inhibitor protein, survivin, and others.[Citation62] There are data indicating that meloxicam effectuates its antitumour effects by targeting the COX-2/MMP-2/E-cadherin, through inhibiting phosphorylation of protein kinase B (PKB, AKT), by upregulating pro-apoptotic proteins (including Bax and Fas-L), downregulating anti-apoptotic proteins (including survivin), and inducing cell autophagy (by upregulating Beclin 1 and light chain 3-II) in COX-2-dependent and -independent pathways.[Citation51,Citation56] The antitumour effect of meloxicam has not been found to be correlated with the level of COX-2 protein expression.[Citation63] The higher cytotoxic / cytostatic activity of the Cu(II) complex of meloxicam, observed in our study, is not surprising. Cu(II) complexes of NSAIDs (piroxicam and meloxicam) were proved to express better anticancer effects than the bare drugs, which can be explained, at least partially, by the ability of Cu(II)-NSAIDs to bind directly to the DNA.[Citation41,Citation64]
The possible chemopreventive/therapeutic potential of NSAIDs in human glioblatoma multiforme and cervical carcinoma are not clarified yet. Promising data have been reported, such as the ability of celecoxib (a COX-2 selective NSAID) to inhibit the proliferation of various glioblatoma cell lines in vitro [Citation65] and to induce apoptosis via the NF-kB pathway.[Citation66] In addition, several case control studies have suggested that NSAIDs reduce the risk for this aggressive brain cancer, but findings from a large prospective study did not support an inverse association between NSAIDs and risk of glioma and glioblastoma.[Citation67] The NSAID sulindac has been reported to induce specific degradation of the Human papilomavirus oncoprotein E7 and to cause growth arrest (inhibition of the G1 to S transition of the cell cycle) and apoptosis in HeLa cervical carcinoma cells.[Citation68]
In our previous investigations we demonstrated the antitumour activity of metal complexes with various ligands including ursodeoxycholic acid [Citation69] and monensin [Citation70]. In this study, we presented data indicating that human glioblatoma multiforme (8MGBA) and uterine cervical carcinoma (HeLa) cells are sensitive not only to the cytotoxic / cytostatic effects of the selective NSAID meloxicam, but are also sensitive at a higher degree to its 3d metal (Cu(II), Zn(II), Co(II), Ni(II)) complexes. The cancer cell growth / survival inhibitory potential of the compounds examined was proved by cytological, cytotoxical and molecular biological methods with different molecular/cellular targets such as mytochondria (MTT), lysosomes (NRCA), nucleus (CVS), DNA (Comet assay) and mechanisms of action; after short (72 h and 96 h) and long (16 d) treatment intervals; in classical 2D monolayer cultures as well as in unchorage-independent 3D cell colonies. The higher antitumour activity of the examined metal complexes deserves special interest at least for one other reason – zinc, copper and cobalt are essential elements and exhibit low toxicity to the humans and animals.
Conclusions
The results obtained by us revealed that the investigated compounds decreased in a time- and concentration-dependent manner the viability and proliferation of the treated 8MGBA and HeLa human cancer cells. Metal complexes, especially Cu(II) complex, are more pronounced cytotoxic and cytostatic agents, as compared to meloxicam. These data provided a new evidence that the selective NSAID meloxicam and its 3d metal (Cu(II), Zn(II), Co(II), Ni(II)) complexes may have cytotoxic and cytostatic activity against human cervical carcinoma and glioblastoma multiforme cells and highlight the need for further research in this field.
Disclosure statement
No potential conflict of interest was reported by the authors.
Additional information
Funding
References
- Laine L. Approaches to nonsteroidal anti-inflammatory drug use in the high-risk patient. Gastroenterol. 2001;120:594–606.
- Díaz-Rodríguez L, García-Martínez O, Morales MA, et al. Effects of indomethacin, nimesulide, and diclofenac on human MG-63 osteosarcoma cell line. Biol Res Nurs. 2012;14(1):98–107.
- Zochling J, Van Der Heijde D, Dougados M, et al. Current evidence for the management of ankylosing spondylitis: a systematic literature review for the ASAS/EULAR management recommendations in ankylosing spondylitis. Ann Rheum Dis. 2006;65:423–432.
- Karateev AE, Erdes ShF. Use of nonsteroidal anti-inflammatory drugs in case of ankylosing spondylitis: long and persistently!. Ter Arkh. 2014;86(11):123–127. (in Russian)
- Van Durme CM, Wechalekar MD, Buchbinder R, et al. Non-steroidal anti-inflammatory drugs for acute gout. Cochrane Database Syst Rev. 2014;9:CD010120.
- Becker DE. Pain management: Part 1: Managing acute and postoperative dental pain. Anesth Prog. 2010;57(2):67–78.
- Silberstein SD, Stirpe JC. COX inhibitors for the treatment of migraine. Expert Opin Pharmacother. 2014;15(13):1863–1874.
- Connolly TP. Cyclooxygenase-2 inhibitors in gynecologic practice. Clin Med Res. 2003;1:105–110.
- Tablov B, Tablov V, Popov I. Modern aspects of perioperative analgesia by using nonsteroidal anti-inflammatory drugs in gynecology (Part I). Akush Ginekol (Sofia). 2006;45(1):41–44. (in Bulgarian)
- Fosslien E. Molecular pathology of cyclooxygenase 2 in neoplasia. Ann Clin Lab Sci. 2000;30:3–21.
- Dempke W, Rie C, Grothey A, et al. Cyclooxygenase-2: a novel target for cancer chemotherapy? J. Cancer Res Clin Oncol. 2001;127:411–417.
- Khan MN, Lee YS. Cyclooxygenase inhibitors: scope of their use and development in cancer chemotherapy. Med Res Rev. 2011;31(2):161–201.
- Setiawan VW, Matsuno RK, Lurie G, Wilkens LR, Carney ME, Henderson BE, Kolonel LN, Goodman MT. Use of nonsteroidal anti-inflammatory drugs and risk of ovarian and endometrial cancer: the Multiethnic Cohort. Cancer Epidemiol Biomarkers Prev. 2012;21(9):1441–1449.
- Cebola I, Peinado MA. Epigenetic deregulation of the COX pathway in cancer. Prog Lipid Res. 2012;51(4):301–313.
- Vardy J, Agar M. Nonopioid drugs in the treatment of cancer pain. J Clin Oncol. 2014;32(16):1677–1690.
- Rigas B, Tsioulias GJ. The evolving role of nonsteroidal anti-inflammatory drugs in colon cancer prevention: a cause for optimism. J Pharmacol Exp Ther. 2015;353(1):2–8.
- Cui Y, Deming-Halverson SL, Shrubsole MJ, et al. Use of nonsteroidal anti-inflammatory drugs and reduced breast cancer risk among overweight women. Breast Cancer Res Treat. 2014;146(2):439–46.
- Huang XZ, Gao P, Sun JX, et al. Aspirin and nonsteroidal anti-inflammatory drugs after but not before diagnosis are associated with improved breast cancer survival: a meta-analysis. Cancer Causes Control. 2015;26(4):589–600.
- Xu J, Yin Z, Gao W, et al. Meta-analysis on the association between nonsteroidal anti-inflammatory drug use and lung cancer risk. Clin Lung Cancer. 2012;13(1):44–51.
- Tan XL, Reid Lombardo KM, Bamlet WR, et al. Aspirin, nonsteroidal anti-inflammatory drugs, acetaminophen, and pancreatic cancer risk: a clinic-based case-control study. Cancer Prev Res (Phila). 2011;4(11):1835–1841.
- Dell'Atti L. Correlation between prolonged use of aspirin and prognostic risk in prostate cancer. Tumori. 2014;100(5):486–490.
- Vidal AC, Howard LE, Moreira DM, et al. Aspirin, NSAIDs, and risk of prostate cancer: results from the REDUCE study. Clin Cancer Res. 2015;21(4):756–762.
- Duncan K, Uwimpuhwe H, Czibere A, et al. NSAIDs induce apoptosis in nonproliferating ovarian cancer cells and inhibit tumor growth in vivo. IUBMB Life. 2012;64(7):636–643.
- Johannesdottir SA, Chang ET, Mehnert F, et al. Nonsteroidal anti-inflammatory drugs and the risk of skin cancer: a population-based case-control study. Cancer. 2012;118(19):4768–4776.
- Chiou SK, Hoa N, Hodges A, et al. Indomethacin promotes apoptosis in gastric cancer cells through concomitant degradation of Survivin and Aurora B kinase proteins. Apoptosis. 2014;19(9):1378–1388.
- Akrami H, Aminzadeh S, Fallahi H. Inhibitory effect of ibuprofen on tumor survival and angiogenesis in gastric cancer cell. Tumour Biol. 2015;36(5):3237–3243.
- Bank A, Yu J, Zhang L. NSAIDs downregulate Bcl-X(L) and dissociate BAX and Bcl-X(L) to induce apoptosis in colon cancer cells. Nutr Cancer. 2008;60(1):98–103.
- De Groot DJ, Van Der Deen M, Le TK, et al. Indomethacin induces apoptosis via a MRP1-dependent mechanism in doxorubicin-resistant small-cell lung cancer cells overexpressing MRP1. Br J Cancer. 2007;97(8):1077–1083.
- Kim MS, Kim JE, Lim do Y, et al. Naproxen induces cell-cycle arrest and apoptosis in human urinary bladder cancer cell lines and chemically induced cancers by targeting PI3K. Cancer Prev Res (Phila). 2014;7(2):236–245.
- Correia I, Arantes-Rodrigues R, Pinto-Leite R, et al. Effects of naproxen on cell proliferation and genotoxicity in MG-63 osteosarcoma cell line. J Toxicol Environ Health A. 2014;77(14-16):916–923.
- Ong CK, Lirk P, Tan CH, et al. An evidence-based update on nonsteroidal anti-inflammatory drugs. Clin Med Res. 2007;5(1):19–34.
- Culita DC, Alexandrova R, Dyakova L, et al. Evaluation of Cytotoxic and Antiproliferative Activity of Co(II), Ni(II), Cu(II) and Zn(II) Complexes with Meloxicam on Virus – Transformed Tumor Cells. Revista de Chimie. 2012;63(4):384–389.
- Perzelova A, Maccova I, Mraz P, et al. Characterization of two new permanent glioma celllines 8-MG-BA and 42-MG-BA. Neoplasma. 1998;42:25–29.
- Callaway E. Deal done over HeLa cell line. Nature. 2013;500(7461):132–133.
- Mosmann T. Rapid colorimetric assay for cellular growth and survival: application to proliferation and cytotoxicity assays. J Immunol Meth. 1983;65(1–2):55–63.
- Borenfreund E, Puerner JA. Toxicity determined in vitro by morphological alterations and neutral red absorption. Toxicol Lett. 1985;24(2–3):119–124.
- Saotome K, Morita H, Umeda M. Cytotoxicity test with simplified crystal violet staining method using microtitre plates and its application to injection drugs. Toxic in vitro. 1989;3(4):317–321.
- Wahab SIA, Abdul AB, Alzubairi AS, et al. J Biomed Biotechnol. 2009;2009:769568. Available from: http://www.ncbi.nlm.nih.gov/pmc/articles/PMC4433944/.
- Olive PL, Banath JP, Durand RE. Heterogeneity in radiation-induced DNA damage and repair in tumor and normal cells measured using the "comet" assay. Radiat Res. 1990;122(1):86–94.
- Niles AL, Moravec RA, Riss TL. Update on in vitro cytotoxicity assays for drug development. Expert Opin Drug Discov. 2008;3(6):655–669.
- Chakraborty S, Bose M, Sarkar M. Spectroscopic studies of the binding of Cu(II) complexes of oxicam NSAIDs to alternating G-C and homopolymeric G-C sequences. Spectrochim Acta A Mol Biomol Spectrosc. 2014;122:690–697.
- Telliez A, Furman C, Pommery N, et al. Mechanisms leading to COX-2 expression and COX-2 induced tumorigenesis: topical therapeutic strategies targeting COX-2 expression and activity. Anticancer Agents Med Chem. 2006;6(3):187–208.
- Capone ML, Tacconelli S, Di Francesco L, et al. Pharmacodynamic of cyclooxygenase inhibitors in humans. Prostaglandins Other Lipid Mediat. 2007;82(1–4):85–94.
- Misra S, Sharma K. COX-2 signaling and cancer: new players in old arena. Curr Drug Targets. 2014;15(3):347–359.
- Bodey B, Siegel SE, Kaiser HE. Cyclooxygenase-2 (COX-2) overexpression in childhood brain tumors. In Vivo. 2006;20(4):519–525.
- Kulkarni S, Rader JS, Zhang F, et al. Cyclooxygenase-2 is overexpressed in human cervical cancer. Clin Cancer Res. 2001;7(2):429–434.
- Xu K, Wang L, Shu HK. COX-2 overexpression increases malignant potential of human glioma cells through Id1. Oncotarget. 2014;5(5):1241–1252.
- Sharma V, Dixit D, Ghosh S, et al. COX-2 regulates the proliferation of glioma stem like cells. Neurochem Int. 2011;59(5):567–571.
- Montejo C, Barciab E, Negro S, et al. Effective antiproliferative effect of Meloxicam on prostate cancer cells: development of a new controlled release. Int J Pharm. 2010;387(1–2):223–229.
- Goldman AP, Williams CS, Sheng H, et al. Meloxicam inhibits the growth of colorectal cancer cells. Carcinogenesis. 1998;19(12):2195–2199.
- Dong X, Li R, Xiu P, et al. Meloxicam executes its antitumor effects against hepatocellular carcinoma in COX-2- dependent and -independent pathways. PLoS One. 2014;9(3):e92864.
- Naruse T, Nishida Y, Hosono K, et al. Meloxicam inhibits osteosarcoma growth, invasiveness and metastasis by COX-2-dependent and independent routes. Carcinogenesis. 2006;27(3):584–592.
- Iwase N, Higuchi T, Gonda T, et al. The effect of meloxicam, a selective COX-2 inhibitor, on the microvasculature of small metastatic liver tumors in rats. Jpn J Clin Oncol. 2007;37(9):673–678.
- Tsubouchi Y, Mukai S, Kawahito Y, et al. Meloxicam inhibits the growth of non-small cell lung cancer. Anticancer Res. 2000;20(5A):2867–2872.
- Xin B, Yokoyama Y, Shigeto T, et al. Anti-tumor effect of non-steroidal anti-inflammatory drugs on human ovarian cancers. Pathol Oncol Res. 2007;13(4):365–369.
- Naruse T, Nishida Y, Ishiguro N. Synergistic effects of meloxicam and conventional cytotoxic drugs in human MG-63 osteosarcoma cells. Biomed Pharmacother. 2007;61(6):338–346.
- Hassan MH, El-Beshbishy HA, Aly H, et al. Modulatory effects of meloxicam on cardiotoxicity and antitumor activity of doxorubicin in mice. Cancer Chemother Pharmacol. 2014;74(3):559–569.
- Hassan MH, Ghobara M, Abd-Allah GM. Modulator effects of meloxicam against doxorubicin-induced nephrotoxicity in mice. J Biochem Mol Toxicol. 2014;28(8):337–346.
- Palayoor ST, J-Aryankalayil M, Makinde AY, et al. Gene expression profile of coronary artery cells treated with nonsteroidal anti-inflammatory drugs reveals off-target effects. J Cardiovasc Pharmacol. 2012;59(6):487–499.
- Tamasi G, Casolaro M, Magnani A, et al. New platinum – oxicamcomplexes as anti-cancer drugs. Synthesis, characterization, release studies from smart hydrogels, evaluation of reactivity with selected proteins and cytotoxic activity in vitro. J Inorg Biochem. 2010;104(8):799–814.
- Cini R., Anti-inflammatory compounds as ligands in metal complexes as revealed in X-ray structural studies. Comments Inorg Chem. 2000;22(3-4):151–186.
- Tinsley HN, Grizzle WE, Abadi A, et al. New NSAID targets and derivatives for colorectal cancer chemoprevention. Recent Results Cancer Res. 2013;191:105–20.
- Ayakawa S, Shibamoto Y, Sugie C, et al. Antitumor effects of a cyclooxygenase-2 inhibitor, meloxicam, alone and in combination with radiation and/or 5-fluorouracil in cultured tumor cells. Mol Med Rep. 2009;2(4):621–625.
- Roy S, Banerjee R, Sarkar M. Direct binding of Cu(II)-complexes of oxicam NSAIDs with DNA backbone. J Inorg Biochem. 2006;100(8):1320–1331.
- Kardosh A, Blumenthal M, Wang WJ, et al. Differential effects of selective COX-2 inhibitors on cell cycle regulation and proliferation of glioblastoma cell lines. Cancer Biol Ther. 2004;3(1):55–62.
- Sareddy GR, Geeviman K, Ramulu C, et al. The nonsteroidal anti-inflammatory drug celecoxib suppresses the growth and induces apoptosis of human glioblastoma cells via the NF-κB pathway. J Neurooncol. 2012;106(1):99–109.
- Daugherty SE, Moore SC, Pfeiffer RM, et al. Nonsteroidal anti-inflammatory drugs and glioma in the NIH-AARP Diet and Health Study cohort. Cancer Prev Res. (Phila). 2011;4(12):2027–2034.
- Karl T, Seibert N, Stöhr M, et al. Sulindac induces specific degradation of the HPV oncoprotein E7 and causes growth arrest and apoptosis in cervical carcinoma cells. Cancer Lett. 2007;245(1–2):103–111.
- Dyakova L, Culia DC, Marinescu G, et al. Metal Zn(II), Cu(II), Ni(II) complexes of ursodeoxycholic acid as putative anticancer agents. Biotechnol Biotechnol Equip. 2014;28(3):543–551.
- Pantcheva I, Alexandrova R, Zhivkova T, et al. In vitro activity of biometal(II) complexes of monensin against virus-induced transplantable animal tumors. Biotechnol Biotechnol Equip. 2013;27(2):3703–3708.