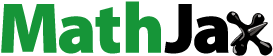
Abstract
Esters, such as methyl oleate, are functionally important compounds in many industrial sectors, mainly as components for manufacturing of emulsifiers, detergents, intermediate stabilizers and wetting agents. Acid functionalization of multi-walled carbon nanotubes (F-MWCNTs) by using a mixture of HNO3 and H2SO4 (1:3, v:v) was employed as the matrix for the adsorption of Candida rugosa lipase (CRL) as nanobioconjugates (CRL-MWCNTs) for the production of methyl oleate. Structural information of the developed CRL-MWCNTs was confirmed using Fourier Transform Infrared spectroscopy, thermogravimetric analysis and transmission electron microscopy, which revealed a successful attachment of CRL onto the F-MWCNTs. Process parameters (reaction time, temperature and alcohol:acid molar ratio) were optimized for high percent conversion of methyl oleate. Structural analysis established that CRL was successfully attached to the surface of the F-MWCNTs. Under the optimized conditions, which were 13.87 h, 51 °C, molar ratio oleic acid:methanol (1:3.80), a high ester yield of 90.90% was attained. Also, under conditions of the shortest reaction time of 6 h, 47.07 °C and acid:methanol ratio of 1:2.54, the CRL-MWCNTs catalysed a 74.51% ester yield. Hence, we established that response surface methodology (RSM) can be a practical technique for the prediction of the conditions that favour the high yield production of methyl oleate. Under optimized conditions, the CRL-MWCNTs nanobioconjugates are potentially good and economical biocatalysts for the production of methyl oleate, as well as other types of commercially important fatty-acid methyl esters.
Introduction
The appeal for clean energy has driven the demands for biodiesel (fatty-acid methyl ester, FAME) to replace petroleum products, due to its biodegradable, renewable, clean and non-toxic nature and also due to its ability to burn completely, among other advantages. Commercial FAME, such as methyl oleate, has been growing tremendously due to its functional importance in the oleochemical, pharmaceutical, food, biodiesel and cosmetic industries. Its uses include production of detergents, emulsifiers, wetting agents and intermediate stabilizers. Methyl oleate is also used as pesticide adjuvant to enhance and speed up the penetration of pesticides and, therefore, to effectively eradicate pests.[Citation1] Nevertheless, the means of extracting such esters from plants or other natural sources are often either too scarce or too expensive for commercial use. In addition, the current chemical approach for the production of methyl oleate has been linked to a number of disadvantages. These include factors, such as the need for high energy, utilization of corrosive acids and other hazardous chemicals, in addition to the undesirable counterproductive degradation of the synthesized ester due to a long reaction period.[Citation2,Citation3] Hence, the quest for alternative methods that would overcome these shortcomings, enhance productivity and improve reaction yields, requires pertinence.
In this context, the enzymatic esterification by using lipases and natural substrates for synthesizing methyl oleate may prove to be a promising alternative route to the prevailing chemical production of esters.[Citation4,Citation5] Such reaction can be performed at ambient conditions, considering the fact that improved production technologies and engineered enzymatic properties are two key elements desired by industrial manufacturers.[Citation6] Other benefits, associated with enzyme-catalysed synthetic reactions, also include being eco-friendly (green synthesis), highly selective and energy saving.[Citation7–9] Lipases (triacylglycerol ester hydrolases EC 3.1.1.3) are one of the most adaptable classes of industrial enzymes with catalysing capabilities in aqueous and organic solvents. Among others, Candida rugosa lipase (CRL) has been widely used in oil hydrolysis, transesterification, esterification and interesterification, as well as in catalysing the hydrolysis of long chain fatty-acid esters. The fact that the free form CRL is often unstable, exhibits low activity in organic solvents and has a high tendency to deactivate in prolonged contact to high temperature and extreme pH,[Citation10] makes the immobilization of the CRL onto a suitable matrix very advantageous.
The immobilization of free or soluble enzymes involves their attachment onto different types of supports that limit the mobility of such enzymes.[Citation11] This technique offers a multitude of benefits that include enhanced structural stability, activity, specificity and selectivity, as well as reduced inhibition.[Citation12,Citation13] Apart from increased flexibility of enzyme/substrate contact,[Citation13] the immobilization process may also protect the enzyme from solvent denaturation.[Citation14] In this context, the simpler and more economical method of immobilization is the physical attachment of CRL onto multi-walled carbon nanotubes (MWCNTs) as a support. This approach is suited for the fitting of large amounts of lipases, allowing large-scale processes [Citation15] and offering a feasible solution for the extension of the reaction life of the lipases.[Citation16] Such an extended reaction life may be accredited to the excellent binding capacity of the MWCNTs due to their large surface area to volume ratio, interesting physicochemical properties and biological compatibility.[Citation16]
Response surface methodology (RSM) is an effective statistical technique for the investigation of complex processes.[Citation17,Citation18] This technique offers many advantages, such as reduced number of experimental runs. This method is faster and less expensive than the classical method for gathering of research information.[Citation19] Optimizing the esterification reaction parameters plays a vital role in the economical manufacturing and in gaining a maximum yield of methyl oleate. In this regard, numerous synthetic processes have adopted this statistical approach, which includes biodiesel production from cotton seed oil,[Citation20] enzyme-catalysed synthesis of wax ester by Lipozyme IM by central composite design and transesterification of palm oil containing free fatty acids [Citation21] based on a Box–Behnken Design. Therefore, the objective of this study was to model the lipase-catalysed esterification reaction for the synthesis of methyl oleate by using immobilized C. rugosa lipase (CRL-MWCNTs). Three variables, including reaction time (6−14 h), reaction temperature (40 −60 °C) and molar ratio of oleic acid to methanol (M:M; 1:2–1:3), were used to evaluate and obtain the optimized conditions by RSM based Box–Behnken Design in 17 experimental runs using the Design Expert 7.1.6 software.
Materials and methods
Materials
MWCNTs were prepared using chemical vapour deposition method. Analytical grade solvents, i.e. methanol, oleic acid and iso-octane were purchased from QReC Chemicals (New Zealand). C. rugosa lipase Type VII (847 U/mg) and sodium hydroxide were purchased from Sigma-Aldrich (St. Louis, USA).
Purification and functionalization of MWCNTs
For purifying the MWCNTs, the MWCNTs (1 g) were transferred into a 100 mL round bottom flask containing 4.0 mol/L HCl (60 mL) and refluxed with stirring for 24 h at 100 °C. Upon cooling to room temperature, the liquid was decanted and the MWCNTs were washed repeatedly with distilled water. The suspension of MWCNTs was suspended by centrifuging (6000 rpm) for 5 min and the liquid was decanted. The process was repeated until no residual acid was detected, after which the purified MWCNTs were dried overnight in an oven at 60 °C.[Citation22] Functionalization of the MWCNTs was performed according to the method described by Yudianti et al.[Citation22] The purified MWCNTs were refluxed in a mixture of concentrated H2SO4 : HNO3 (3:1, v/v) for 6 h at 100 °C and the suspension was left overnight to ensure deposition of the functionalized MWCNTs (F-MWCNTs). Then the suspension was diluted and rinsed with distilled water, until no residual acid was detected, and subsequently dried at 80 °C.
Immobilization of CRL onto F-MWCNTs
The F-MWCNTs (100 mg) were suspended in a 50 mL flask with 20 mL phosphate buffer (pH 7.0) that contained 3 mg/mL CRL and incubated at 4 °C for 3 h with constant stirring at 150 rpm. The suspension was centrifuged for 10 min at 6000 rpm, the liquid was decanted and the unbound proteins were removed by repeated washing with phosphate buffer (pH 7.0) until no evidence of hydrolytic activity was detected in the washings. The CRL-MWCNTs were lyophilized overnight and stored at 4 °C until further use.[Citation23,Citation24]
Determination of the protein concentration
The protein concentration was determined by following the method described by Bradford [Citation25] and bovine serum albumin (BSA) was used as the reference standard for constructing a calibration curve.
Characterization of CRL-MWCNTs
The CRL-MWCNTs were characterized using Fourier Transform Infrared (FTIR) (BOMEM, Canada), thermogravimetric analysis (TGA) (Perkin Elmer) and transmission electron microscope (TEM) (JEOL, USA). For the infrared analysis, 2 mg of the sample was ground thoroughly with potassium bromide (ratio of 1:100) and the resulting powder was pressed onto a transparent pellet using a hydraulic press (Specac's Atlas, UK). The FT−IR spectra were obtained using a spectrophotometer (Perkin Elmer) in transmission mode between 400 and 4000 cm−1, with a resolution of 4 cm−1. TGA was conducted in a compact ceramic alumina crucible and heated from 30 to 800 °C under a nitrogen atmosphere using a Thermogravimetric Analyzer 4000 (Perkin Elmer) at a heating rate of 10 °C/min. The morphologies of the synthesized MWCNTs, F-MWCNTs and CRL-MWCNTs were examined by using TEM analysis. The analysis was executed using electron source as W-emitter and LaB6 that operated at an accelerating voltage of 200 kV. The analysis employed an objective lens (S-Twin) having a point resolution of 2.0 nm, or better, with a 25 ×, 500 ×, 200 × or higher magnification and a single tilt holder with a liquid crystal display camera (LCD) camera (Canon). The 0.5 mg sample of MWCNTs, F-MWCNTs or CRL-MWCNTs was ultrasonicated for 2 h in deionized water and a drop of each sample was positioned on a copper grid and observed after drying in vacuum.
Esterification reaction
A typical esterification reaction was performed in a mixture of methanol, oleic acid and iso-octane as solvent in a 50 mL round-bottom flask. The preparations containing the CRL-MWCNTs were in a working volume of 10 mL and the mixture was refluxed with stirring at 200 rpm in a paraffin oil bath. Aliquots (1 mL) of the reaction mixture were withdrawn at fixed intervals of 1 h and analysed following titration with NaOH (0.02 mol/L) using phenolphthalein as an indicator. Samplings were conducted in triplicate and the results were calculated from the average of three independents. The percentage conversion was calculated [Citation26] as follows:
(1)
(1) where V0 is the volume of NaOH at initial time (t = 0) and Vt is the volume of NaOH at a particular interval (t = t1, t2, t3 …).
Analysis of the product by gas chromatography (GC)
To further analyse the content of the obtained products from the esterification reaction, 1 mL sample from the reaction mixture was withdrawn at 0 h and 11 h. Then, it was immediately analysed by GC equipped with a flame ionization detector (FID) (Perkin Elmer, USA). The analysis was conducted using a DB-Wax column (30 m × 0.53 mm, 0.5 µm) and 1 µL of sample was injected into GC for each run. The temperature of the injector was 250 °C and the detector was kept at 270 °C. The oven temperature was set at 60 °C for 1 min and then increased to 220 °C in 10 min as a final temperature.[Citation27] Ester identification was done by comparing the retention time of the sample with the retention time of the standard (a method using Methyl heptadecanoate as an internal standard).[Citation28]
Experimental design and optimization using response surface methodology
A Box–Behnken experimental design with three variables and a total of 17 reactions was used to study the response pattern and to determine the optimum combination of variables. The effects of A (reaction time, h), B (reaction temperature, °C) and C (molar ratio of oleic acid to methanol) at three variables' levels (–1, 0, +1) in the reaction process were evaluated. The software Design Expert 7.1.6 was used for designing and analysing the experimental data, in which the percentage conversion of oleic acid was the response of the experimental design. The independent variables and their levels, as well as the actual and coded values, are illustrated in . Three repeated experiments at the centre of the design were performed to permit the estimation of the pure error. Unforeseen variability in the observed response, due to extraneous influences, was reduced by randomizing all experiments.
Table 1. The actual and coded independent variables in the Box–Behnken design for the CRL-MWCNTs catalysed synthesis of methyl oleate.
The model equation was used to estimate the optimum value and, subsequently, to describe the interaction between the factors. The quadratic equation model for predicting the optimal point was expressed as follows:
(2)
(2) where Y is the response, i and j are the linear and quadratic coefficients, respectively, Xi and Xj are the uncoded independent variables and regression coefficients, k is the number of studied and optimized factors in the experiment, β0 is a constant coefficient, βj, βjj, and βij are the interaction coefficients of linear, quadratic and second-order terms, respectively, k is the number of studied factors and ϵ is the error. The value of correlation coefficient (R2) was used as a tool to express the quality of the fit of the polynomial model. The Fisher's F-test which measures the degree of freedom, relative to the residual obtained, was used to test for the significance of the model at the 5% confidence level. The significance of a model was represented by a p-value < 0.05. Analysis of variance (ANOVA) was also carried out.
Results and discussion
Characterization of CRL-MWCNTs
Fourier Transform Infrared spectroscopy
shows the spectra of as-synthesized MWCNTs ((a)), F-MWCNTs ((b)) and CRL-MWCNTs ((c)). The spectrum of as-synthesized MWCNTs revealed only two weak bands at 1632.68 cm−1, which was attributed to conjugated –C = C– bonds, and at 3432.04 cm−1, which was assigned to –OH stretching of the surface oxidized acidic groups. A prominent peak at 2800–2900 cm−1 ( (b)) that emerged after purification and functionalization processes corresponded to the C–H asymmetric and symmetric stretching vibration, derived from long alkyl chain. For the F-MWCNTs ((b)), a new weak peak was observed at 1382.79 cm−1, which could be assigned to C–O stretching vibrations, consistent with MWCNTs before acid treatment.[Citation29]
Following the acid functionalization process, a peak appearing in the region of 1730–1650 cm−1 was expected to indicate the development of carboxylic groups on the MWCNTs.[Citation22] Appearance of peak at 1742 cm−1 assigns carbonyl (C = O) stretching vibration of carboxyl groups,[Citation30,Citation31] indicating the expansion of carboxylation on the surfaces of F-MWCNTs. On the other hand, for the CRL-MWCNT spectrum ((c)), strong peaks at 1634 cm−1 were characteristic of the C = O stretching of amide of the polypeptide chains of CRL. The spectrum of CRL illustrated a medium intensity sharp peak at 1121 cm−1 owned to the vibrations of –C–O carbohydrate moiety. However, the intensity of –C = O is reduced, expanded, and shifted to a lower frequency of 1121 cm−1, 1110 cm−1 in the CRL-MWCNTs. Hence, the investigation agreed with previous findings by Prlainovic et al.[Citation32]
Thermogravimetric analysis (TGA)
The thermal decomposition of both synthesized-MWCNTs and acid-functionalized MWCNTs were analysed by a thermogravimetric analyser and the results are illustrated in . The thermogram revealed that a large loss of mass of approximately 62% was observed for the MWCNTs, between 550 °C and 700 °C ((a)). The acid F-MWCNTs demonstrated a loss of mass of about 76.7% over a broader temperature range between 400 and 700 °C for ((b)), with first loss of mass occurring at 150 °C due to the decomposition of surface hydroxyl groups. On the other hand, the carboxyl groups –COOH and C = O decomposed at higher temperatures (350−450 °C).[Citation33] No further decay in mass was observed after 700 °C, as beyond this point the remaining residue consisted essentially of thermally stable carbon.[Citation22] Since enzyme molecules tend to decompose at lower temperatures than other chemical groups [Citation22] introduced onto the MWCNTs in this study, the loss of mass (19%) for CRL-MWCNTs that occurred at lower temperatures (150 −450 °C) ((c)) may be accredited to the decomposition of the CRL protein. Hence, the results showed that physical adsorption had succeeded in attaching approximately 19% of CRL protein to the surface of the F-MWCNTs.
Transmission electron microscopy (TEM)
The TEM analyses revealed differences in the morphology of the as-synthesized MWCNTs, F-MWCNTs and CRL-MWCNTs (). The F-MWCNTs were observably shorter and the fibres remained relatively defected ((b)) than the as-synthesized MWCNTs ((a)) as a result of a stronger acid treatment (H2SO4:HNO3) during the functionalization process.[Citation22,Citation34] The open-ended pipes on the F-MWCNTs hypothetically increase the surface area for the attachment of carboxylic groups at the open ends,[Citation35] thereby rendering the surface accessible for biological and chemical reactions.[Citation22] The TEM image of the CRL-MWCNTs ((c)) depicted a layer of lipase protein that coated the surface of the MWCNTs, confirming the successful immobilization of CRL.
Analysis of the product by gas chromatography
The conversion of methyl oleate by the CRL-MWCNTs nanobioconjugates was analysed and identified by GC analysis. The sequence of boiling point values for the elements of methyl oleate was methanol (64.7 °C) < methyl oleate (351 °C) < oleic acid (360 °C). Therefore, based on (a), at 0 h reaction time, the presence only of oleic acid peak at retention of 19.667 min was shown. After the following 11 h of incubation ((b)), a new peak appeared at 19.367 min that can be assigned to methyl oleate and, therefore, tend to have a shorter retention time. Hence, the results established that the enzymatic synthesis of methyl oleate was successful.
RSM experiments and model fitting
RSM is a well-accepted statistical technique that is capable of solving multivariate equations simultaneously from analyses of multivariate data.[Citation36] The use of such method is advantageous, as it entails minimum numbers of experiments to predict the optimum conditions, obtained by multiple regression analysis of the quantitative data.[Citation36] Consequently, a graphical response surface is generated to represent the relative equation, which describes the individual and cumulative effect of the experimental variables and their subsequent effect on the response.[Citation37] In this study, the percentage conversion of oleic acid for the enzymatic production of methyl oleate was modelled using the method of RSM (), utilizing three reaction parameters: reaction time (h), reaction temperature (°C) and molar ratio of methanol to oleic acid. The effect of three experimental factors and their interaction was established and each response surface was plotted as a function of two targeted variables, while other variables were held constant.
The results for the actual values acquired from the response from the experimental runs and the predicted values obtained from the generated quadratic model [Citation38] that corresponded to the oleic acid percent conversions are tabulated in . The conversion of oleic acid to methyl oleate in the reactions was found to be in the range of 60.8%–89.9%. The best fitting model was established by a regression analysis, whereby fitting of the data to various models (linear, two factorial, quadratic and cubic) and their subsequent ANOVA illustrated that the CRL-MWCNTs catalysed esterification of methanol and oleic acid was suitably characterized by a quadratic polynomial model. The significant terms and the equation, in terms of coded factors, are represented as follows:
(3)
(3) where Y is the conversion of oleic acid (%), A is the reaction time (h), B is the temperature (°C) and C is the molar ratio of methanol to acid. Synergistic effect between mutual interacting or individual parameters is represented by a positive sign in front of the term, whereas the negative sign illustrates the antagonistic effects. The occurrence of antagonistic interaction usually corresponds to the influence of independent variables on the esterification reaction process. Furthermore, the very small p-value (≪ 0.05) [Citation36] coupled with the corresponding large F-value [Citation39] makes the corresponding coefficient of a particular term in the ANOVA more significant. Therefore, the results obtained in this investigation clearly revealed that the variable that exerted the largest effect on the esterification reaction was the reaction time.
Table 2. Experimental design and results of the response surface design.
The relationship between the predicted and experimental percentage conversion is shown in . A high coefficient of determination (R2 = 0.9763) between the predicted and experimental specific activities confirmed that the quadratic polynomial model was adequate to explain the actual relationship between the response and the significant variables. The ANOVA of the regression model () demonstrated that the model was significant, evident from a very low probability value of the Fisher's F-test [(P model > F) – 0.0001]. It can be seen that the computed value of the model (31.9731) is very high, as compared with the tabular F0.05 (9,7) − 3.68, indicating that the degree of freedom relative to the residual obtained for the model is significant at the 5% confidence level.
Figure 6. Comparison between the predicted and actual values for the percentage conversion in the CRL-MWCNTs catalysed synthesis of methyl oleate.
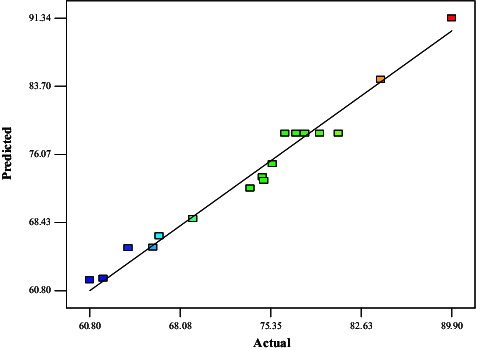
Table 3. Analysis of variance and coefficients of the model.
The ANOVA () showed that most of the independent variables were statistically significant for the CRL-MWCNTs catalysed synthesis of methyl oleate, except the temperature (p-value = 0.1708). However, the insignificant term was retained in the model equations for the purpose of maintaining the required hierarchy of the model. The reaction temperature may not have been a significant term, but the interacting variables (time × temperature, temperature × molar ratio, quadratic effects of temperature and molar ratio) were significant and could have an impact effect on the response. Although, one of parent terms may not be significant, the parent term should also be included in the model, whenever the interacting terms are significant.[Citation40]
According to the ANOVA of factors, the F-value for the lack of fit (1.5829) was lower than the tabulated value of F0.05 (3, 4) – 6.59, suggesting that the lack of fit was insignificant relative to the pure error, thereby indicating the data in the experimental domain were well represented by the model.[Citation41] This was corroborated by a p-value < 0.05 that confirmed that the quadratic model was statistically significant and sufficient to explain the actual relationship between the response and the significant variables.[Citation13,Citation42] The validity of the model was also confirmed by the high value of the adjusted R2 (0.9457), which suggested that there was a total variation of 94% for the acid conversion to the independent variables. Only 6% of the total variation could not be explained by the model. According to literature, the experimental and predicted values share good correlation when the value of multiple correlation coefficient (R) approaches 1.[Citation43] In this study, the value of R2 (0.9763) indicated a good relation between the experimental and predicted values of the response; hence, their relationships, represented by the model, were well within the chosen range.
Effect of process variables on the percentage yield of methyl oleate
illustrates the effect of the process variables, namely, time (A), temperature (B) and molar ratio of acid to alcohol (C) on the percentage yield of methyl oleate. It can be seen that the percentage of conversion of methyl oleate increased with the increase of the reaction time (A). The conversion of methyl oleate initially exhibited an upward trend with the increase of temperature (B). This observation can be attributed to the continual reduction in the structural rigidity of CRL-MWCNTs, consistent with unfolding of the lipase structure whenever the reaction temperature is elevated. The CRL-MWCNTs attained their catalytically competent form at approximately 50 °C, reflected in the high yield of the ester at this particular point. It can be concluded that the immobilization process considerably altered the structure of the CRL-MWCNTs, following adsorption on to the highly thermostable carbon nanotubes supports. The F-MWCNTs allowed for a robust setting for the CRL enzyme linkage [Citation32,Citation44] and improved the hydrophobicity of the microenvironment.[Citation45,Citation46]
Figure 7. Deviation of the reference point for the percentage conversion of methyl oleate for the effect of time (A), temperature (B) and molar ratio (C).
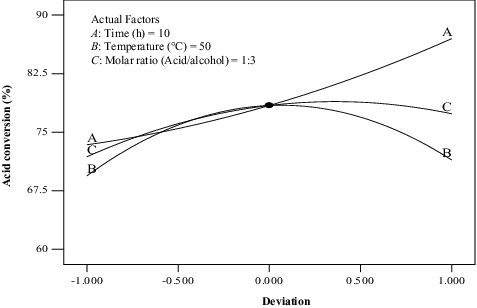
Nonetheless, the concentration of the formed ester declined at temperatures exceeding 50 °C, which was probably caused by the partial inactivation of the CRL-MWCNTs. This outcome was somewhat anticipated as the reaction temperature (T > 50 °C) had exceeded the optimal temperature of the enzyme. It can be seen that the presence of excess methanol in the reaction medium inhibited the activity of the CRL-MWCNTs when the (acid/alcohol) molar ratio (C) exceeded 1:3, hence resulting in a drop in the production of methyl oleate. According to a previous literary review, the presence of a large excess of ethanol can flood the active sites of CRL and saturate the catalytic surface with the alcohol, or prevent nucleophilic attack by shielding the protonated alcohol by its own excess.[Citation36]
Mutual effect of experimental factors on the synthesis of methyl oleate
Effect of time and temperature
illustrates the response surface curve and contour plot for the effect of time (A) and temperature (B), and their mutual interaction on the synthesis of methyl oleate by CRL-MWCNTs at a constant molar ratio of 1:3 (acid to alcohol). It was observed that the interactions of the two reaction parameters were significant because of the small p-value (0.0021) (). The effect of time on the yield of methyl oleate was very significant, as compared to the temperature of the reaction. The F-value indicated that the effect of time (104.69) was more significant, as compared with the esterification temperature (2.33), implying that the reaction temperature played a pivotal role in governing the yields of the ester. Also, it was clearly apparent from the figure that a yield as high as 87% of methyl oleate was attainable at 13.5 h and at any temperature value between 47 and 56 °C.
Figure 8. Graphs depicting the response surface plot (a) and contour plot (b) showing the effect of time (A) and temperature (B), and their mutual interaction in the enzymatic synthesis of methyl oleate at a constant molar ratio of 1:3 (oleic acid:methanol).
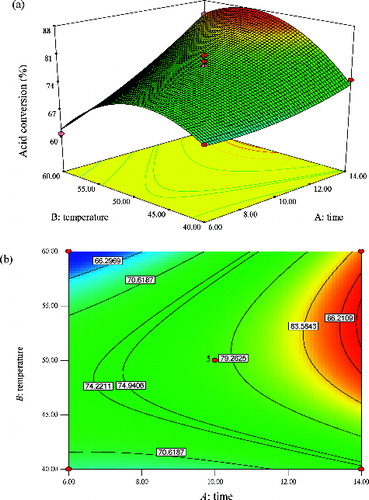
Increasing the contact time between the enzyme and substrates significantly elevated the percentage conversion of methyl oleate. It can be concluded that the reaction time had a positive effect on the synthesis of methyl oleate. The outcome is also reflected in the predictive equation (EquationEquation (3)(3)
(3) ), in which the factor of time has a positive coefficient. Nonetheless, the CRL-MWCNTs catalysed esterification achieved an equilibrium state at 13.5 h. The continual increase in percentage conversion of ester, with the increase of reaction time, could be associated with the longer reaction time for the CRL-MWCNTs to catalyse the esterification reaction. Hence, the prolonged contact time increased the probability of effective collisions between enzyme and substrates [Citation36]; thereby a higher percentage yield of methyl oleate was achieved. This outcome is suggestive of the conformational change of CRL-MWCNTs that is related to greater protein unfolding [Citation42,Citation44] of the structurally more rigid CRL-MWCNTs biocatalysts at high temperatures of 42–56 °C.
It is a well-known fact that temperature has two important roles in any reaction system. An increase in reaction temperature brings several benefits, which include reduced mixture viscosity and improved substrate diffusion process.[Citation42] Henceforth, such changes possibly improved the integration of reactants and alleviated problems associated with mass transfer limitations, thus favouring effective interactions between enzyme molecules and reactants [Citation42] that promoted higher yield of the ester. However, there is a limit as to how high an enzyme catalysed reaction should be increased. Higher reaction temperatures tend to promote propensity of enzyme inactivation, due to conformational changes in the enzyme tertiary structure.[Citation45,Citation46] The study found that by increasing the reaction temperature up to 56 °C, higher yields of methyl oleate were possible, albeit by also increasing the reaction time. From an economic point of view, it is more desirable for the esterification process to be executed at a much lower reaction temperature, because less energy is required to operate the large bioreactors.[Citation42]
Effect of time and molar ratio
Response surface curve and contour plot showing predicted response surface of methyl oleate percent acid conversion as a function of time and molar ratio (acid/alcohol) at a constant temperature of 50 °C is depicted in . Again, the results clearly revealed that the effect of time on the yield of methyl oleate was more significant than molar ratio. The F-value of time (104.69) was higher than that of the molar ratio (17.18), suggesting that the reaction time had more impact on the yield of methyl oleate (). According to the ANOVA of factors, the mutual interaction between both parameters was very significant, which is supported by a very small p-value (0.0007). It can be seen that the highest percentage conversion can be achieved during longer incubation time as well as a higher molar ratio of the substrate. Hence, this observation is referred to the direct proportionality between reaction time and molar ratio of the substrate. The results showed that a yield of methyl oleate as high as 90% could be attained at a reaction time of ∼13.5 h by using a substrate molar ratio in the range of 1:3.3–1:4.0 (acid:alcohol).
Figure 9. Graphs depicting the response surface plot (a) and contour plot (b) showing the effect of time (A) and molar ratio (C), and their mutual interaction in the enzymatic synthesis of methyl oleate at a constant temperature (50 °C).
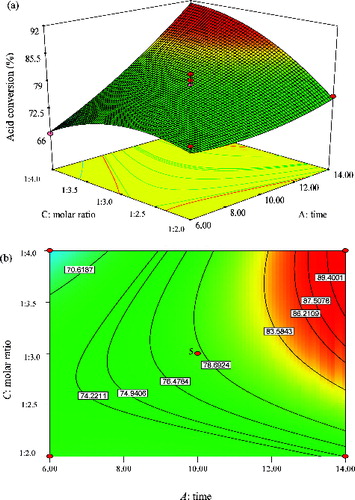
It was established from the investigation that both parameters had to be increased to their maximum values in order to obtain high yields of methyl oleate. The outcome suggested that the enzyme activity can be enhanced by increasing the concentration of alcohol and that the substrate molar ratio is one of the main variables that can determine the fate of the percentage conversion of methyl oleate. The improvement in the yield of the ester at higher molar ratios of substrates may be attributed to a better solubility of oleic acid in higher amounts of methanol and also a greater availability of the methanol for the enzyme.[Citation39] Several studies have reported some inhibitory effects resulting from high concentrations of alcohol during the esterification reaction [Citation44,Citation47]; however, the results of this study revealed otherwise. This is because the nature of the esters that will be synthesized varies from one ester to another. Therefore, the determining of the extent of molar ratio of acid:alcohol required in any enzyme catalysed esterification reaction are, expectedly, not the same.[Citation48]
According to some reports, at higher methanol to acid molar ratios, the rate of reaction be will be enhanced.[Citation21,Citation44] Likewise, a higher ratio over the stoichiometric value can result in a higher rate of ester formation, thereby, ensuring the complete reaction.[Citation21] In fact, high alcohol:acid ratio promoted the esterification reaction towards a high product formation.[Citation49] Thus, the yield of methyl oleate rose with the increase in the quantity of methanol. The positive effect exerted by methanol on the percent yield of methyl oleate is corroborated in the observed positive coefficient (EquationEquation (3)(3)
(3) ) proposed for the factor of molar ratio in the model equation. The outcome can be explained by the thermodynamics of the reaction, which favour product formation when the starting material is present in excess.[Citation50] For that very reason, many lipase catalysed esterification reactions intentionally employ an excess of alcohol to improve the yield of ester,[Citation50] apart from reducing the toxicity of the acids in the reaction medium that could deactivate enzyme activity.[Citation51]
Effect of temperature and molar ratio
depicts the mutual interaction on effect of temperature with molar ratio at a constant time of 10 h on the CRL-MWCNTs catalysed synthesis of methyl oleate. The maximum predicted values were indicated by the surface confined in the smallest ellipse in a contour plot.[Citation52,Citation53] Elliptical contours are obtained when there is a perfect interaction between the independent variables.[Citation21,Citation52,Citation53] It was observed that there was a significant interaction between both variables. This was signified by a maximum predicted yield represented by the surface confined by the smallest ellipse in the contour plot ( (b)). The elliptical profile of the contour plot indicated that the interaction between the amount of methanol and temperature is strong. Although the interaction between temperature and molar ratio was strong, their mutual interaction, however, was the least favourable (F-value = 8.44), as compared to the interactions between time and molar ratio (F-value = 33.44) ().
Figure 10. Graphs depicting the response surface plot (a) and contour plot (b) showing the effect of temperature (B) and molar ratio (C), and their mutual interaction in the enzymatic synthesis of methyl oleate at constant time (10 h).
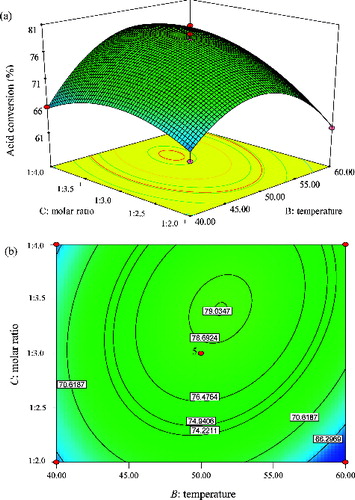
According to , the F-value of temperature was significantly lower (2.33) and less important than the molar ratio (17.18), which strongly indicated that molar ratio was the variable with the biggest impact on the esterification process in obtaining a high yield of the ester. As shown in (b), a high conversion of approximately ∼80% can be achieved at a temperature of 52 °C and molar acid:alcohol ratio of 1:3.4. According to other studies, the equilibrium of reactions can be affected by the temperature of the reaction.[Citation15,Citation36] In general, elevating the reaction temperature increases the percentage conversion, as the equilibrium constant was also increased. Apart from the improvement in mass transfer limitation between reactant and catalyst, the solubility of the reaction mixture is increased [Citation49] and the viscosity of the medium is reduced, therefore, enhancing proper mixing of the reactants.[Citation41] In addition, the higher temperature also activates the CRL-MWCNTs due to greater unfolding of the lipase structure, consequently enhancing the conversion of the substrate.[Citation41]
Attachment of CRL onto F-MWCNTs potentially increased the structural rigidity of the enzyme; therefore, premature protein unfolding of the CRL-MWCNTs was averted. Hence, the CRL-MWCNTs were rendered more resistant against thermal denaturation and were able to catalyse at higher temperatures.[Citation54] However, a decline in the yield of ester when the reaction temperature exceeded 52 °C was possibly attributable to the reversible nature of the esterification reaction. According to a previous literary review, a high reaction temperature can result in the decrease of percentage conversion. This is probably linked to the superheated excessive evaporation of the reactant in the reaction system,[Citation35] which, in this case, could lead to the loss of methanol. It is pertinent to highlight the fact that increasing the temperature beyond 52 °C did not improve the percentage conversion of the ester, due to the probable increase in the inactivation of the enzyme as the protein denaturation process had begun to set in.[Citation49] It was obvious that increasing the substrate's molar ratio (acid:alcohol, 1:3.4) had a positive effect on the yield of methyl oleate. The result is evidently a consequence of the accessibility of the enzymes to large amounts of substrates which increased the probability of substrate-enzyme collision.[Citation41,Citation54] Furthermore, an excess of alcohol will shift the thermodynamic equilibrium of the reaction towards the formation of ester.[Citation49]
Attaining optimum conditions and model verification
The highest percentage conversion from the various run was 89.9% at 14 h of reaction time, 50 °C and substrate molar ratio of 1:4 oleic acid:methanol (). Minimizing the production cost is an important aspect to keep in mind [Citation41] when developing industrial processes for the esterification of methanol with oleic acid for the production of detergents, emulsifiers, wetting agents and also as intermediate stabilizers.[Citation1] Therefore, a statistically assisted optimized process is an appropriate method to solve this problem. In this regard, attaining a high degree of conversion was possible by solving the regression equation (EquationEquation (1)(1)
(1) ) using Design Expert 7.1.6 and purely seeking the optimum point on the response surface.[Citation41] The determined optimum point indicated the necessary optimal combination of variables to achieve the highest percentage conversion.
To check the adequacy of the model equation, sets of confirmation experiments were carried out within and outside the design space. By using the optimization function of the Design Expert 7.1.6, two maximum percentage conversions were selected based on: (Equation1(1)
(1) ) the highest percent conversion with all variables in the model range and (2) the highest percent conversion at the shortest reaction time. The experiments for both of the proposed optimization conditions were conducted and the results are shown in . The obtained results were found to be relatively close to the predicted percentage conversion. Similarities in the predicted and actual values confirmed the eligibility of the quadratic model suggested by the software. Thus, RSM with appropriate experimental design could be effectively applied to optimize the process parameters in this investigation. The results also showed that the simplistic developed enzyme revealed good tendency to produce methyl oleate while giving high percentage conversion of the ester under optimized conditions.
Table 4. The attained optimum conditions for the CRL-MWCNTs catalysed synthesis of methyl oleate.
Comparison with other studies employing enzyme assisted synthesis of methyl oleate
To date, research on enzymatic assisted esterification for the synthesis of methyl oleate catalysed by lipase or immobilized lipase onto MWCNTs has yet to be widely explored. In another study, cell-bound lipase (CBL) from Geotrichum sp. was reported to be effectively applied in repeated synthesis of methyl oleate in a microaqueous solvent.[Citation55] Four recombinant CRL isozymes (LIP1–LIP4) were used for the production of FAME. The optimal FAME conversion yield obtained was (61.5% ± 1.5%) at 22 h, 20 °C with molar methanol:oil ratio 4 and 3 methanol addition times (1 mol/6 h).[Citation56] Celite® R632-supported lipase sol–gels (Novozym® 435 and Lipase PS ‘Amano’ SD) were produced to catalyse enzymatic transesterification of triolein to produce methyl oleate,[Citation57] which demonstrated optimized production of enzyme from an endophytic yeast C. guilliermondii isolated from castor leaves (Ricinus communis L.) and of the immobilization process by covalent bonding onto agarose and 60 G silica gel for the enzymatic synthesis of methyl oleate, producing a high conversion yield (86%). Shu et al. [Citation58] studied a novel solid acid catalyst, sulfonated-multiwalled carbon nanotubes (s-MWCNTs), to synthesize methyl oleate (95.46%) at conditions of catalyst/oleic acid mass ratio of 0.20%, methanol/oleic acid molar ratio of 5.8, temperature of 135 °C, and reaction time of 1.5 h.
Conclusions
The present study outlined the response methodological approach for the synthesis of the FAME methyl oleate by using nanobioconjugates of C. rugosa lipase adsorbed onto MWCNTs. Careful observation of the three important parameters at individual and combinatorial levels by RSM method via the Box–Behnken design showed strong mutual interactive effects on the methyl oleate synthesis. The highest percentage conversion was attained under the optimized conditions of 90.90% with all variables in the model range and 74.51%, under the shortest reaction time. We demonstrated that RSM can be a practical technique to predict the conditions that favour high yield production of methyl oleate. Based on the findings, the CRL-MWCNTs nanobioconjugates showed potential as good biocatalysts to produce methyl oleate. Their development also turned out to be relatively cheap, when compared to Novozyme (> US$900) and Lipozyme (US$400).
Acknowledgments
The authors would like to express their appreciation to the Faculty of Science, Universiti Teknologi Malaysia for their facilities.
Disclosure statement
The authors declare that they have no competing interests.
Additional information
Funding
References
- Long X, Jian L, Lina Y, et al. Study on synthesis of methyl oleate catalyzed by ceric ammonium sulfate. Int J Sci Engine Res. 2013;4(9):1909–1911.
- Aranda DAG, Santos RTP, Tapanes NCO, et al. Acid-catalyzed homogeneous esterification reaction for biodiesel production from palm fatty acids. Catal Lett. 2008;122:20–25.
- Lotero E, Liu Y, Lopez DE, et al. Synthesis of biodiesel via acid catalysis. Ind Eng Chem Res. 2005;44:5353–5363.
- Pecnik S, Knez Z. Enzymatic fatty acid ester synthesis. J Am Oil Chem Soc. 1992;69:261–265.
- Mahmood I, Ahmad I, Chen G, et al. A surfactant-coated lipase immobilized in magnetic nanoparticles for multicycle ethyl isovalerate enzymatic production. Biochem Eng J. 2013;73:72–79.
- Iyer PV, Ananthanarayan L. Enzyme stability and stabilization-Aqueous and non-aqueous environment. Process Biochem. 2008;43:1019–1032.
- Rios AP, Hernadenez-Fernandez FJ, Gomez D, et al. Understanding the chemical reaction and mass-transfer phenomena in a recirculating enzymatic membrane reactor for green ester synthesis in ionic liquid/supercritical carbon dioxide biphasic system. J Supercrit Fluids. 2007;43(2):303–309.
- Szczesna-Antczak M, Kubiak A, Antczak T, et al. Enzymatic biodiesel synthesis - key factors affecting efficiency of the process. Renew Energy. 2009;34:1185–1194.
- Sun WJ, Zhao HX, Cui FJ, et al. D-isoascorbylpalmitate: lipase-catalyzed synthesis, structural characterization and process optimization using response surface methodology. Chem Cent J. 2013;7:114.
- Zhou X, Nie H, Yao Z, et al. Facile synthesis of nanospindle-like Cu2O/straight multi-walled carbon nanotube hybrid nanostructures and their application in enzyme-free glucose sensing. Sens Actuators B Chem. 2012;168:1–7.
- Khan AA, Alzohairy MA. Recent advanced and application of immobilized enzyme technologies: A review. Res J Bio Sci. 5. 2010;8:565–575.
- Mateo C, Palomo JM, Fernandez-Lorente G, et al. Improvement of enzyme activity, stability and selectivity via immobilization techniques. Enz Microb Technol. 2007;40:1451–1463.
- Mohamad NR, Huyop F, Aboul-Enein HF, et al. Response surface methodological approach for optimizing production of geranyl propionate catalysed by carbon nanotubes nanobioconjugates. Biotechnol Biotechnol Equip. 2015a;29(4):732–739.
- Karra-Chaabouni M, Ghamgui H, Sofiane B, et al. Production of flavour esters by immobilized Staphylococcus simulans lipase in a solvent-free system. Process Biochem. 2006;41:1692–1698.
- Zhao X, Qi F, Yuan C, et al. Lipase-catalyzed process for biodiesel production: Enzyme immobilization, process simulation and optimization. Renew Sust Energ Rev. 2015;44:182–197.
- Zhang P, Henthorn DB. Synthesis of PEGylated single wall carbon nanotubes by a photoinitiated graft from polymerization. AIChE J. 2010;56:1610–1615.
- Sinkuniene D, Bendikiene V, Juodka B. Response surface methodology-based optimization of lipase-catalyzed triolein hydrolysis in hexane. Romanian Biotechnol Lett. 2011;16(1):5891–5901.
- Rodrigues RC, Ayub MAZ. Effects of the combined use of Thermomyces lanuginosus and Rhizomucor miehei lipases for the transesterification and hydrolysis of soybean oil. Process Biochem. 2011;46(3):682–688.
- Basri M, Zaliha RR, Ebrahimpour A, et al. Comparison of estimation capabilities of response surface methodology (RSM) with artificial neural network (ANN) in lipase-catalyzed synthesis of palm-based wax ester. BMC Biotechnol. 2007;7:53–63.
- Fan X, Wang X, Chen F. Biodiesel production from crude cottonseed oil: an optimization process using response surface methodology. TOEFJ. 2011;4:1–8.
- Dwivedi G, Sharma MP. Application of Box–Behnken design in optimization of biodiesel yield from Pongamia oil and its stability analysis. Fuel. 2015;145:256–262.
- Yudianti R, Onggo H, Sudirman, et al. Analysis of functional group sited on multi-wall carbon nanotube surface. TOMSJ. 2011;5:242–247.
- Ye Y, Zhu-Ping H, Yong-Juan X, et al. Effect of support surface chemistry on lipase adsorption and activity. J Mol Catal B: Enzym. 2013;94:69–76.
- Mohamad NR, Marzuki NHC, Buang NA, et al. An overview of technologies for immobilization of enzymes and surface analysis techniques for immobilized enzymes. Biotechnol Biotechnol Equip. 2015b;29(2):205–220.
- Bradford MM. A rapid and sensitive method for the quantitation of microgram quantities of protein utilizing the principle of protein-dye binding. Anal Biochem. 1976;72:258–254.
- Radzi SM, Mustafa WAF, Othman SS, et al. Green synthesis of butyl acetate: a pineapple flavour via lipase catalyzed reaction. World Acad Sci Eng Technol. 2011;59:677–680.
- Mohammad Fauzi AHM, Amin NAS. Optimization of oleic acid esterification catalyzed by ionic liquid for green biodiesel synthesis. Energ Convers Manage. 2013;76:818–827.
- Li D, Xiaojing W, Kaili N, et al. Synthesis of wax esters by lipase-catalyzed Esterification with immobilized lipase from Candida sp. 99–125*. Chinese J Chem Eng. 2001;19(6):978–982.
- Gong H, Kim ST, Lee JD, et al. Simple quantification of surface carboxylic acids on chemically oxidized multi-walled carbon nanotubes. Appl Surf Sci. 2013;266:219–224.
- Chen S, Shen W, Wu G, et al. A new approach to functionalization of single-walled carbon nanotube with both alkyl and carbonyl groups. Chem Phys Lett. 2005;402:302–317.
- Lee SY, Park SJ. Hydrogen adsorption of acid-treated multi-walled carbon nanotubes at low temperature. Bull Korean Chem Soc. 2010;31:1596–1600
- Prlainovic NZ, Bezbradica DI, Knezevic-Jugovic ZD, et al. Adsorption of lipase from Candida rugosa on multi walled carbon nanotubes. J Ind Eng Chem. 2013;19:279–285.
- Raghavendra T, Basak A, Manocha LM, et al. Robust nanobioconjugates of Candida antarctica lipase B – Multiwalled carbon nanotubes: Characterization and application for multiple usages in non-aqueous biocatalysis. Bioresour Technol. 2013;140:103–110.
- Osorio AG, Silveira ICL, Bueno VL, et al. H2SO4/HNO3/HCl - Functionalization and its effect on dispersion of carbon nanotubes in aqueous media. Appl Surf Sci. 2008;255(5):2485–2489.
- Liu J, Rinzler AG, Dai H, et al. Fullerene pipes. Science. 1998;280:1253–1255.
- Liu W, Yin P, Liu X, et al. Design of an effective bifunctional catalyst organotriphosphonic acid-functionalized ferric alginate (ATMP-FA) and optimization by Box–Behnken model for biodiesel esterification synthesis of oleic acid over ATMP-FA. Bioresour Technol. 2014;173:266–271.
- Sultania M, Rai JS, Srivastava D. Process modeling, optimization and analysis of esterification reaction of cashew nut shell liquid (CNSL)-derived epoxy resin using response surface methodology. J Hazard Mater. 2011;185(2–3):1198–1204.
- Jaliliannosrati H, Amin NAS, Talebian-Kiakalaieh A, et al. Microwave assisted biodiesel production from Jatropha curcas L. seed by two-step in situ process: Optimization using response surface methodology. Bioresour Technol. 2013;136:565–573.
- Rahman MB, Chaibakhsh N, Basri M, Rahman RNZRA, Salleh AB, Mat Radzi S. Modelling and optimization of lipase-catalysed synthesis of dilauryladipate ester by response surface methodology. J Chem Technol Biotechnol. 2008;83:1534–1540.
- Piexoto J. A property of well-formulated regression models. The Am Stats. 1990;44:26–30.
- Nuthalapati V, Chidambaram R, Das Gupta N, et al. Optimization of growth medium using a statistical approach for the production of Plant Gallic acid from a newly isolated Aspergillustubigiensis NJA-1. J Pure Appl Biol. 1999;8:3313–3324.
- Wahab RA, Basri M, Rahman RNZRA, et al. Enzymatic production of a solvent-free menthyl butyrate via response surface methodology catalyzed by a novel thermostable lipase from Geobacillus zalihae. Biotechnol Biotechnol Equip. 2014;28:1065–1072.
- Pujari V, Chandra TS. Statistical optimization of medium components for improved synthesis of riboflavin by Eremotheciumashbyii. Bioprocess Eng. 2000;23:303–307.
- Mohamad NR, Buang NA, Mahat NA, et al. A facile enzymatic synthesis of geranyl propionate by physically adsorbed Candida rugosa lipase onto multi-walled carbon nanotubes. Enzym Microb Tech. 2015;72:49–55.
- Miranda M, Urioste D, Andrade Souza LT, et al. Assessment of the morphological, biochemical, and kinetic properties for Candida rugosa lipase immobilized on hydrous niobium oxide to be used in the biodiesel synthesis. Enzym Res. 2011;2011:216435.
- Chaibakhsh N, Abdul Rahman MB, Abd-Aziz S, et al. Optimized lipase-catalysed synthesis of adipate ester in a solvent free system. J Ind Microbiol Biotechnol. 2009;36:1149–1155.
- Kuo CH, Chen HH, Chen JH, et al. High yield of wax ester synthesized from cetyl alcohol and octanoic acid by Lipozyme RMIM and Novozyme 435. Intl J Mol Sci. 2012;13:11694–11704.
- Salihu A, Alam MZ, Karim MIA, et al. Esterification for butyl butyrate formation using Candida cylindracea lipase produced from palm oil mill effluent supplemented medium. Arab J Chem. 2014;7(6):1159–1165.
- Raghavendra T, Panchal N, Divecha J, et al. Biocatalytic synthesis of flavor ester ‘pentyl valerate’ using Candida rugosa lipase immobilized in microemulsion based organogels: effect of parameters and reusability. Bio Med Res Int. 2014;2014:353–845.
- Martins AB, Graebin NG, Lorenzoni ASG, et al. Rapid and high yields of synthesis of butyl acetate catalyzed by Novozym 435: Reaction optimization by response surface methodology. Process Biochem. 2011;46:2311–2316.
- Couto R, Vidinha P, Peres C, et al. Geranyl acetate synthesis in a packed-bed reactor catalyzed by Novozym in supercritical carbon dioxide and in supercritical ethane. Ind Eng Chem Res. 2011;50(4):1938–1946.
- Feng Y, Zhao X, Lv F, et al. Optimization on Preparation Conditions of Salidroside Liposome and Its Immunological Activity on PCV-2 in Mice. J Evid Based Complementary Altern Med. 2015;2015:178128.
- Wu CY, Liu Z, Hu Y, et al. Optimization on preparation conditions of Rehmannia glutinosa polysaccharide liposome and its immunological activity, Carbohydrate Polymers. 2014;104(1):118–126.
- Pires-Cabral P, da Fonseca MMR, Ferreira-Dias S. Esterification activity and operational stability of Candida rugosa lipase immobilized in polyurethane foams in the production of ethyl butyrate. Biochem Eng J. 2010;48(2):246–252.
- Yan JY, Yan YJ. Optimization for producing cell-bound lipase from Geotrichum sp. and synthesis of methyl oleate in microaqueous solvent. Appl Microbiol Biotechnol. 2008;78:431–439.
- Chang SW, Huang M, Hsieh YH, et al. Simultaneous production of fatty acid methyl esters and diglycerides by four recombinant Candida rugosa lipase's isozymes. Food Chem. 2014;155:140–145.
- Oliveira ACD, Watanabe FMF, Vargas JVC, et al. Production of methyl oleate with a lipase from an endophytic yeast isolated from castor leaves. Biocatal Agric Biotechnol. 2012;1:295–300.
- Shu Q, Zhang Q, Xu G, et al. Preparation of biodiesel using s-MWCNT catalysts and the coupling of reaction and separation. Food Bioproducts Process. 2009;8(7):164–170.