ABSTRACT
Panax notoginseng has been used for medicinal purposes in China for many years. Saponins are believed to be the major bioactive ingredients in P. notoginseng. Two different processes, steaming and biotransformation, were used to study the transformations of saponins in P. notoginseng. During an 8-h steaming process, the ginsenosides Rb1, Rd, Rg1, Re and notoginsenoside R1, decreased to 1.07 mg/g dry weight (DW), 0.91, 0.64, 0 and 0 mg/g DW, respectively. Meanwhile, ginsenoside 20(S)-Rg3, 20(S)-Rh1, F2 and compound K significantly increased to 5.85, 6.10, 0.81 and 6.62 mg/g DW, respectively. On the other hand, one fungus was isolated from the root of P. notoginseng, which could transform ginsenoside Rb1 to ginsenoside Rd specifically. The fungus was identified as Trichoderma longibrachiatum species based on sequence analysis of the rRNA internal transcribed spacers region. The results implied a prospective feasibility for setting up different processing techniques to improve the quality of P. notoginseng and add its value.
Introduction
Panax notoginseng (Chinese ginseng) is one of the Chinese traditional medicinal plants, which belongs to Panax genus, Araliaceae family and has been used by ethnic people in Southwestern China for treatment of cardiovascular diseases, inflammation, body pain and trauma for more than 400 years. It was included as a dietary supplement by the US Dietary Supplement Health and Education Act in 1994.[Citation1] Traditionally, raw notoginseng is used as trauma hemostatic and cardiovascular medicine, whereas the processed form is used as tonic because of its blood cell increasing functions. In the past 20 years, many chemical and pharmacological studies on P. notoginseng have been carried out.[Citation2–4] Triterpenoid saponins, including ginsenosides, notoginsenosides and gypenosides, are considered to be the main bioactive compounds. So far, more than 70 saponins have been isolated from P. notoginseng, among which ginsenosides Rb1, Rg1, Re and notoginsenoside R1 are the major components, whereas the others have low contents.[Citation5]
Various studies have focused on the pharmacological activities of the minor ginsenosides, as their activities were found to be more valuable than those of the major ginsenosides.[Citation6–8] The minor ginsenosides, including ginsenosides F1, F2, Rg3, compound K (C-K) and Rh1, can be produced by hydrolysis of glycosides from the major ginsenosides Rg1, Re, Rb1 and notoginsenoside R1. Therefore, some reports have aimed to convert major ginsenosides to minor ginsenoides with higher activities.[Citation9–11] Several transformation methods have been used to achieve the aim,[Citation11,Citation12] including mild acid hydrolysis,[Citation13] enzymatic conversion [Citation14] and microbial conversion,[Citation15] but the chemical methods also bring side reactions such as epimerization, hydration and hydroxylation.[Citation16]
Because of the relative backward processing technology, the traditional Chinese medicine was used mainly for rough processing. It would be significant to make full use of the existing resources and add their value by means of utilizing new and advanced processing technologies.
In this study, the two processing methods of steaming and microbial transformation of P. notoginseng were compared and the dynamic curves of saponin transformation were investigated. One fungus was isolated from the root of P. notoginseng, which could transform ginsenoside Rb1 to ginsenoside Rd specifically. The possible transformation pathways of saponins in the processes were also discussed.
Materials and methods
Materials
The standards of ginsenosides were obtained from the National Institutes for Food and Drug Control, China. Their chemical structures are shown in . Acetonitrile was purchased from Merck Darmstadt, Germany and methanol was purchased from Tianjin Chemical Ltd., China. Raw roots of P. notoginseng were purchased from Miaoxiang Ltd., Wenshan country, Yunnan province, China and comminuted to 60-mesh size particles with electric grinder (Beijing Zhongxingweiye Instrument Co. Ltd., China) and sieve.
Fungal cultures
WYC2012 was one of the microorganism strains isolated from raw roots of P. notoginseng. We cultivated it on potato dextrose agar (PDA) containing 2% (w/v) sucrose, 2% (w/v) agar and 20% (v/v) potato extract. It was cultured on PDA medium at 23 °C for five days and stored at 4 °C until further use.
Fungal spore suspensions were prepared by washing five-day-old culture slants with sterile 0.9% NaCl and shaking at 100 rpm for 20 min. Spores were counted with a haemocytometer and adjusted to approximately 107 spores per mL.
Steaming of notoginseng
Notoginseng root (dry) was steamed at 120 °C for 0.5, 1, 1.5, 2, 3, 4, 6 and 8 h. The raw notoginseng was used as control. The raw and steamed samples were frozen for 2 h before lyophilization (Shanghai Pudong Freeze Dryer Equipment Co. Ltd., China). The obtained ginsenoside contents were expressed as mg/g of dry weight (DW).
Biotransformation of ginsenosides
Erlenmeyer flasks (100 mL) containing 20 mL of sterilized medium, with an initial pH of 5.0 were inoculated with 2% (v/v) fungal spore suspension and incubated at 28 °C with a rotary speed of 150 rpm. After the fungal spore suspension was incubated for 24 h, P. notoginseng or monomer ginsenoside (ginsenoside Rb1) was added to a final concentration of 10 mg/mL or 1 mg/mL (the latter was used in the present study) to initiate biotransformation. The sterilized raw notoginseng, which proceeded the biotransformation without the WYC2012 fungal strain, was used as control. The fermentation times were 24, 36, 48, 60, 72, 84, 96, 108 and 120 h. Three flasks were run under the same conditions and the data represented were the mean values with standard deviations from three independent samples. The obtained ginsenoside contents were expressed as mg/g of DW.
High performance liquid chromatography (HPLC) analysis
The raw and steamed samples and biotransformated mixtures were extracted with n-butanol saturated with H2O, evaporated under vacuum (Shanghai Jinghong Laboratory Equipment Co. Ltd., China), and the residues were dissolved in methanol for the HPLC analysis.
HPLC analysis was performed on a Dionex Ultimate 3000 liquid chromatograph (Sunnyvale, CA, USA) equipped with a quaternary pump, an automatic injector and a photodiode array detector 3000. The reversed-phase column used was a Waters Symmetry C-18 (5 µm, 4.6 mm × 250 mm) column. The gradient elution system consisted of water (A) and acetonitrile (B). Separation was achieved by using the following gradient: 0–30 min: 20% B, 30–60 min: 20%–45% B, 60–78 min: 45%–75% B, 78–80 min: 75%–80% B. The column temperature was set at 30 °C. The flow rate was 1 mL/min and the injection volume was 10 µL. The UV detection wavelength was set at 203 nm. The compounds quantification was done via standard curves, which were made by using reference compounds.
Molecular methods
The genomic DNA of the strain WYC2012 was extracted and purified by the E.Z.N.A.® Fungal DNA Kit (Omega Bio-Tek Inc., USA). The internal transcribed spacer (ITS) rRNA gene sequences of the strain WYC2012 was sequenced by the Shanghai Majorbio Bio-Pharm Technology Co. Ltd., China. The ITS rRNA gene sequences of the related taxa were obtained from GenBank. A phylogenetic tree was constructed using the neighbour-jointing method through the MEGA 5.05 programme. A bootstrap analysis with 1000 replicates was also conducted to obtain confidence levels for the branches. ITS1-5.8S-ITS2 rRNA gene sequences of the strain WYC2012 were aligned with those of the type strains found to have the closest taxonomic relationships.
Two oligonucleotide primers, forward primer 5'-GGAAGTAAAAGTCGTAACAAGG-3' and reverse primer 5'-TCCTCCGCTTATTGATATGC-3', were used for the polymerase chain reaction (Applied Biosystems, USA) to amplify the ITS rRNA gene. The amplification procedure was: 95 °C for 4 min; then 30 cycles at 94 °C for 45 s, 53 °C for 45 s and 72 °C for 50 s, with a final extension at 72 °C for 8 min.
Statistical analysis
A one-way analysis of variance (ANOVA) was employed to determine whether the results had statistical significance. In some cases, Student's t-test was used for comparing two groups. Both statistical analyses were processed by SPSS (Statistical Product and Service Solutions) software. The level of statistical significance was set at p < 0.05.
Results and discussion
Effect of steaming on notoginseng constituents
The HPLC chromatograms of raw P. notoginseng, steamed P. notoginseng and microbially transformed P. notoginseng are shown in . The contents of ginsenosides in the raw and steamed samples are shown in . The results indicated a distinct difference in saponin composition between the raw and steamed P. notoginseng. During the steaming process, ginsenosides Rb1, Rd, Rg1, Re and notoginsenoside R1, the five main saponin constituents in the raw notoginseng, decreased gradually, and reached 1.07, 0.91, 0.64, 0 and 0 mg/g DW, respectively during the 8-h steaming process, whereas some new saponins were formed (). [Citation17] In the process of steaming, the transformation of saponins began at an early stage. For instance, decreases of Rg1, Rb1, Rd, Re and R1 could be observed evidently after 2 h of steaming (A)). Then, the extent of transformation (both degradation and formation) of saponins increased steadily during the steaming process. After 4 h of steaming, Re was difficult to be detected in the chromatogram; Rg1, Rb1, Rd and R1 were at very low concentrations; ginsenoside 20(S)-Rg3, 20(S)-Rh1 and F2, which were trace saponins in raw notoginseng, significantly increased to 5.85, 6.10, 0.81 mg/g DW, respectively; C-K reached 6.69 mg/g DW, which could not be detected in raw P. notoginseng (B)). The peak areas of six ginsenosides, 20(R)-Rh1, 20(R)-Rg3, Rk3, Rh4, Rk1 and Rg5, were significantly increased as compared to the raw sample (B)).
Figure 2. HPLC chromatograms of raw P. notoginseng (A), steamed P. notoginseng at 120 °C for 4 h (B) and biotransformed P. notoginseng with T. longibrachiatum for 72 h (C).
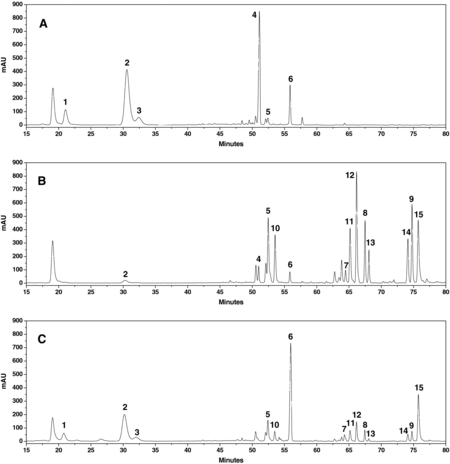
Table 1. Ginsenoside contents in raw notoginseng and in notoginseng steamed at 120 °C.
Figure 3. Transformation curves of the saponins R1, Rg1, Re, Rb1, Rd (A) and 20(S)-Rh1, F2, 20(S)-Rg3, C-K (B) during the steaming process.
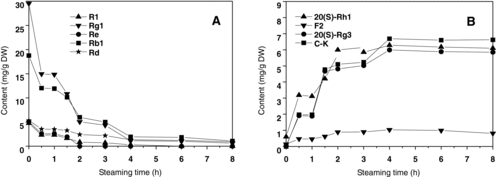
The glycosyl group is prone to being hydrolyzed in dammarane-type tetracyclic triterpenoid glycosides of notoginseng. Some reactions, including rearrangement, reduction and oxidation, can occur at the side chain to produce diverse products.[Citation17,Citation18] The mechanism of saponin transformations in the steaming process could be extrapolated from the chemical structures of saponins, especially the changes in their sugar moiety. During the steaming, the C-20 glucosyl group of ginsenoside Rb1 was hydrolyzed to yield ginsenoside Rd. The hydrolysis of the xylosyl residue attached to C-6 of notoginsenoside R1 and the hydrolysis of the rhamnosyl residue at C-6 of ginsenoside Re formed ginsenoside Rg1. Rg1 and Rd were likely to be the parent compounds of newly formed saponins. Further hydrolysis of the glucosyl group at C-20 of Rg1 yielded Rh1, which then formed Rh4 and Rk3 through dehydration at C-20. Similarly, the hydrolyzation of the glucosyl groups at C-3 and C-20 of Rd produced F2 and Rg3, respectively. Ginsenoside F2 was hydrolyzed at the glucosyl group of C-3 to yield C-K. The dehydration of Rg3 at C-20 yielded Rg5 and Rk1 ().
Biotransformation of ginsenosides by WYC2012
The identity of the confirmed saponins following biotransformation is shown in C) and . Ginsenoside Rb1 (12.03 mg/g DW) and Rg1 (14.95 mg/g DW), the main constituents in raw notoginseng, were transformed significantly by the fungal fermentation. After fermentation for 72 and 120 h, Rb1 and Rg1 decreased with 96.6% and with 28.1%, respectively. During a fermentation time from 0 to 72 h, the protopanaxatriol groups of R1, Rg1, Re and 20(S)-Rh1 changed little, but the protopanaxadiol group of Rb1 and Rd changed significantly. The level of the ginsenoside Rd significantly increased from 3.54 to 15.40 mg/g DW, and the level of ginsenoside Rb1 decreased from 12.03 to 0.41 mg/g DW after 72 h of fermentation.
Table 2. Ginsenoside contents in sterilized notoginseng and in notoginseng biotransformed by the strain WYC2012.
Some reports have demonstrated that ginsenoside Rb1 is deglycosylated by intestinal bacteria after oral administration.[Citation19,Citation20] The deglycosylated aglycone metabolites, such as compound K, Rd and Rg3 have a wide range of pharmacological properties. Therefore, knowledge, regarding the biotransformation of major ginsenosides to their respective deglycosylated metabolites, is of great importance.
In order to confirm that ginsenoside Rd was derived from ginsenoside Rb1, monomer ginsenoside Rb1 was added to the medium for biotransformation. The conversion yield of ginsenoside Rb1 by WYC2012 was confirmed quantitatively by HPLC analysis. As shown in , almost all ginsenoside Rb1 was transformed to ginsenoside Rd. Thus, WYC2012 transformed 1.0 mg/mL ginsenoside Rb1 to 0.791 mg/mL ginsenoside Rd, corresponding to a molar conversion yield of 92.6%.
Figure 5. Time course of Rd production from Rb1 during the biotransformation process by WYC2012 strain. Note: Results are obtained as mean values ± standard deviation (SD).
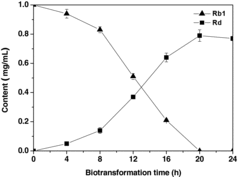
Theoretically, four glucose moieties attached to the ginsenoside Rb1 could be available for hydrolysis by WYC2012, namely, the outer and inner glucose moieties attached at positions C-3 and C-20. Based on an analysis of the hydrolysis products of ginsenoside Rb1, WYC2012 hydrolyzed the outer glucose moiety attached to the C-20 position for ginsenoside Rd production. Furthermore, the inner glucose moiety attached to the C-3 position of ginsenoside Rb1 was not hydrolyzed.
Phylogenetic study
The phylogenetic tree constructed during the study is shown in . Strain WYC2012, which was calculated to belong to the Trichoderma genus, had the highest degree of ITS rRNA gene sequences similarity with the T. longibrachiatum strain DAOM 167674 (EU280099) (100%) and T. longibrachiatum strain DAOM 231259 (EU401562) (99%). Therefore, the strain WYC2012 was classified as T. longibrachiatum. As determined by taxonomic evaluation, strain showing less than 1% difference in its ITS rRNA gene sequence with the corresponding type stains was assumed to belong to the same species as the type stains.
Conclusions
The biotransformation of major ginsenosides to their respective deglycosylated metabolites is of great importance and several kinds of microbes have been used in such researches. In the present study, T. longibrachiatum WYC2012 could convert ginsenoside Rb1 to ginsenoside Rd with high selectivity, and the molar conversion yield reached 92.6%. The specificity of the biotransformation (Rb1–Rd), which can produce ginsenoside Rd efficiently, has not been reported before. In conclusion, two different processes, steaming and biotransformation, were used to study the transformations of saponins in P. notoginseng. The result implies a prospective feasibility for setting up different processing techniques to improve the quality of P. notoginseng and add its value. On the other hand, processed P. notoginseng will provide a potential access to certain effective saponin components, such as Rh1, Rg3, compound K, Rd which are in low contents or absent in plants.
Compliance with Ethics Requirements
This article does not contain any studies with human or animal subjects.
Disclosure statement
The authors declare that they have no conflict of interests.
Additional information
Funding
References
- Sun S, Wang CZ, Tong R, et al. Effects of steaming the root of Panax notoginseng on chemical composition and anticancer activities. Food Chem. 2010;118:307–314.
- He NW, Zhao Y, Guo L, et al. Antioxidant, antiproliferative, and pro-apoptotic activities of a saponin extract derived from the roots of Panax notoginseng (Burk.) F.H. Chen. J Med Food. 2012;15:350–359.
- Sun S, Qi LW, Du GJ, et al. Red notoginseng: Higher ginsenoside content and stronger anticancer potential than Asian and American ginseng. Food Chem. 2011;125:1299–1305.
- Wan JB, Lee SM, Wang JD, et al. Panax notoginseng reduces atherosclerotic lesions in apoE-deficient mice and inhibits TNF-α-Induced endothelial adhesion molecule expression and monocyte adhesion. J Agri Food Chem. 2009;57:6692–6697.
- Kim DH. Chemical diversity of Panax ginseng, Panax quinquifolium, and Panax notoginseng. J Ginseng Res. 2012;36:1–15.
- Wang CZ, Mcentee E, Wicks S, et al. Phytochemical and analytical studies of Panax notoginseng (Burk.) F. H. Chen. J Nat Med. 2006;60:97–106.
- Peng XD, Dai LL, Huang CQ, et al. Relationship between anti-fibrotic effect of Panax notoginseng saponins and serum cytokines in rat hepatic fibrosis. Biochem Biophys Res Commun. 2009;388:31–34.
- Laua AJ, Toh DF, Chua TK, et al. Antiplatelet and anticoagulant effects of Panax notoginseng: comparison of raw and steamed Panax notoginseng with Panax ginseng and Panax quinquefolium. J Ethnopharmacol. 2009;125:380–386.
- Choi WY, Choi WS, Kwon HS, et al. Enhancement of low molecular weight ginsenosides from low-quality ginseng through ultra-high-pressure and fermentation processes. Eur Food Res Technol. 2013;237:429–440.
- Quan LH, Min JW, Jin Y, et al. Enzymatic biotansformation of ginsenoside Rb1 to compound K by recombinant β-glucosidase from Microbacterium esteraromaticum. J Agri Food Chem. 2012;60:3776–3781.
- Kim YS, Yoo MH, Lee GW, et al. Ginsenoside F1 production from ginsenoside Rg1 by a purified β-glucosidase from Fusarium moniliforme var. subglutinans. Biotechnol Lett. 2011;33:2457–2461.
- Kim DK, Baik MY, Kim HK, et al. Standardization of ginseng processing for maximizing the phytonutrients of ginseng. Food Sci Biotechnol. 2013;22:221–226.
- Han BH, Park MH, Han YN, et al. Degradation of ginseng saponins under mild acidic conditions. Planta Med. 1982;44:146–149.
- Ko SR, Choi KJ, Uchida K, et al. Enzymatic preparation of ginsenosides Rg2, Rh1, and F1 from protopanaxatriol-type ginseng saponin mixture. Planta Med. 2003;69:285–286.
- Park CS, Yoo MH, Noh KH, et al. Biotransformation of ginsenosides by hydrolyzing the sugar moieties of ginsenosides using microbial glycosidases. Appl Microbiol Biotechnol. 2010;87:9–19.
- Choi HS, Kim SY, Park Y, et al. Enzymatic transformation of ginsenosides in Korean Red Ginseng (Panax ginseng Meyer) extract prepared by Spezyme and Optidex. J Ginseng Res. 2014;38(4):264–269.
- Wang D, Liao PY, Zhu HT, et al. The processing of Panax notoginseng and the transformation of its saponin components. Food Chem. 2012;132:1808–1813.
- Liao PY, Wang D, Zhang YJ, et al. Dammarane-type glycosides from steamed notoginseng. J Agri Food Chem. 2008;56:1751–1756.
- Akao T, Kida H, Kanaoka M, et al. Intestinal bacterial hydrolysis is required for the appearance of compound K in rat plasma after oral administration of ginsenoside Rb1 from Panax ginseng. J Pharm Pharmacol. 1998;50:1155–1160.
- Odani T, Tanizawa H, Takino Y. Studies on the absorption, distribution, excretion and metabolism of ginseng saponins. IV. Decomposition of ginsenoside-Rg1 and -Rb1 in the digestive tract of rats. J Chem Pharm Bull. 1983;31:3691–3697.