ABSTRACT
In this study, an Hsp70 gene was isolated from maize and was designated as ZmERD2 (Early Responsive to Dehydration 2). The full cDNA sequence of ZmERD2 was 2375 bp. It includes a 1949 bp open reading frame (ORF) encoding 649 amino acids. The calculated molecular mass was 71.163 kDa. The protein encoded by ZmERD2 contains an Hsp70 conservative structure domain, three typical Hsp70 family signature sequences and a cytosolic Hsp70-specific motif, which belongs to the Hsp70 family. Phylogenetic tree analysis suggested that ZmEDR2 shares high similarity (92%–95%) with Hsp70 of other plants, such as Arabidopsis thaliana (NP 195870), Triticum urartu (EMS52605), Aegilops tauschii (EMT07406) and Nicotiana tabacum (AAR17080), and was clustered together with T. urartu and A. tauschii. The promoter of ZmERD2, which was located at about 2.2 kb upstream of ZmERD2, was cloned and was predicted to contain important regulatory elements, including TATA-box, CAAT-box and drought-, heat-, cold-, salicylic acid- and methyl jasmonate-responsive elements. Analyzed by quantitative real-time polymerase chain reaction, the expression of ZmERD2 in maize was induced by heat, high-salt, cold, polyethylene glycol, heat stress and dehydration treatments, but not by abscisic acid. The expression of ZmEDR2 was quickly induced at 42 °C and reached its peak after 1 h of heat stress, with an expression of 40-fold above the control level. These results suggested that ZmERD2 might be a stress-related gene in maize.
Introduction
Heat shock proteins (HSPs) are a protein family which can increase the tolerance ability when plants are exposed to heat shock and other environmental abiotic stress factors.[Citation1,Citation2] Based on molecular size, HSPs are grouped into six major families: Hsp110, Hsp90, Hsp70, Hsp60, Hsp40 and small HSPs.[Citation3] Among them, the Hsp70 family is an important member, since it is highly conservative and widely distributed in the cell nucleus, cytoplasm, endoplasmic reticulum, mitochondria and chloroplast and other parts of the cell.[Citation4–6] Hsp70s are essential chaperones and play a key role in refolding of partially unfolded or denatured proteins,[Citation7,Citation8] photosynthesis [Citation9] and abiotic stress response.[Citation10] They also find application in areas such as environmental monitoring [Citation11] and genetic breeding.[Citation12] For example, it is reported that the expression of Hsp70 was significantly increased to improve the tolerance ability of plant to environmental stress.[Citation13–16] Overexpression of HSC70-1 in Arabidopsis could improve the resistance to heat and high salinity.[Citation13] Tolerance to water stress and dehydration of tobacco was improved because of overexpression of Hsp70.[Citation14,Citation15] According to the results reported by Collins et al. [Citation16], under cold stress, the tolerance of mung bean hypocotyls to cold was improved after heat shock (40 °C, 3 h).
In this study, a gene encoding Hsp70 was cloned from maize (Zea mays) and designated as ZmERD2 (Early Responsive to Dehydration 2). Its gene structure, phylogenetic characteristics and the subcellular localization of the protein were characterized. Moreover, the mRNA levels of ZmERD2 under different stress conditions were investigated using quantitative real-time polymerase chain reaction (qRT-PCR) with the aim to preliminarily explore its relation to environmental stress response and provide a theoretical basis for cultivation of resistant maize varieties.
Materials and methods
Plant materials and growth conditions
Huangzao 4 was used in the experiments. It is a maize inbred line developed by Beijing Academy of Agriculture and Forestry Sciences and the Institute of Crop Sciences of the Chinese Academy of Agricultural Sciences. This line has strong combining ability and high adaptability.
Plants were grown in pots (four plants per pot) containing 20 kg of nutrition soil (soil:vermiculite:organic fertilizer in a 3:2:1 ratio) and cultured in a light incubator at 25 °C under a 14/10 h (day/night) photoperiod.
RNA/DNA isolation
Total RNA was isolated from leaves with Trizol reagent (Invitrogen) according to the manufacturer's instructions. DNA was extracted using a DNAsecure Plant Kit (TIANGEN Biotech Co., Ltd., Beijing, China).
Cloning the full-length cDNA of ZmERD2
The full-length cDNA of the ZmERD2 gene (about 2.3 kb) was cloned by reverse transcription PCR (RT-PCR). A gene-specific primer pair for ZmERD2 was designed based on the ERD2 homologous sequences in Arabidopsis thaliana. Two specific primers, ZmERD2-F1: 5′-CAATCCCTTCCCGTGCTCCGCA-3′ and ZmERD2-R1: 5′-GAAATTCATAAACTCCCACCC-3′, were designed and used to obtain a full-length ZmERD2 cDNA fragment of about 2.3 kb. PCR was performed in a 50 μL reaction volume containing 25.0 μL of 2×Taq PCR MasterMix (TransGen Biotech, China), 2.0 μL of the first-strand cDNA from the maize inbred line Huangzao 4, 1.0 μL of each primer (10 μmol/L), and ddH2O up to 50 μL. The amplifications were performed with an initial denaturation at 94 °C for 5 min, followed by 35 cycles of denaturation (94 °C, 30 s), annealing (60 °C, 30 s), extension (72 °C, 2 min) and a final extension (72 °C, 10 min) step. The PCR products were separated in a 1.0% agarose gel and the amplified bands were purified from the agarose gel by DNA fragment recovery kit (TaKaRa), subcloned into a pMD19-T vector (TaKaRa) and then sequenced (BGI, Qingdao, China).
Sequence and phylogenetic analyses
The theoretical molecular weight (MW) and isoelectronic point (pI) of the protein encoded by ZmERD2 were calculated using the ExPASy pI/Mw tool. The transmembrane helices of the protein were predicted by TMHMM Server v. 2.0 (http://www.cbs.dtu.dk/services/TMHMM/). Subcellular localization of the deduced polypeptides was predicted by WoLF PSORT (http://www.genscript.com/wolf-psort.html) [Citation17] and TargetP 1.1 Server (http://www.cbs.dtu.dk/services/TargetP).[Citation18] Multiple sequence alignments were generated using Clustal X 1.83. The neighbour-joining algorithm of the MEGA 5.10 program [Citation19] was used to construct a phylogenetic tree with Poisson correction and pairwise deletion parameters; all other parameters were set to default. A total of 1000 bootstrap replicates were performed. The three-dimensional structure of the protein encoded by ZmERD2 was predicted using SWISS-MODEL software (http://swissmodel.expasy.org/interactive) and was visualized by Swiss-PdbViewer 3.7.
Cloning and analysis of the ZmERD2 gene promoter
The promoter sequence, which was about 2.3 kb upstream of ZmERD2, was obtained by sequence alignment in the MaizeGDB database (http://www.maizegdb.org/). The specific primers ZmERD2-F2 (5′-ATGCGAGTGTTTGTTTGTAGGGAC-3′) and ZmERD2-R2 (5′-CCACACGCCGACGCAAGAGTACGT-3′) were designed for amplification of the promoter of ZmERD2. The amplifications were performed with an initial denaturation at 94 °C for 5 min, followed by 35 cycles of denaturation (94 °C, 30 s), annealing (57 °C, 30 s), extension (72 °C, 2 min 20 s) and a final extension (72 °C, 10 min) step. The PCR products were separated in a 1.0% agarose gel, and the amplified bands were purified by TaKaRa MiniBEST Agarose Gel DNA Extraction Kit Ver. 4.0 and sequenced (BGI, Qingdao, China). PlantCARE (http://bioinformatics.psb.ugent.be/webtools/plantcare/html/) was used for promoter analysis of cis-regulatory elements.
Stress treatment
Seven treatments were designed, including a control group. Prior to subjecting plants to stress treatment, equal volumes of water were applied to each pot every other day to keep the plants in all pots under uniform water status conditions. Seedlings at the three-leaf stage were exposed to six different stress factors: cold (4 °C), heat (42 °C), abscisic acid (ABA; 100 μmol/L), poly(ethylene glycol) (PEG 6000, 20%), salt (100 mmol/L NaCl) or dehydration. In PEG and NaCl stress treatment, the roots of the plants were immersed in solution. In ABA treatment, the foliar spray method was used (5 mL per plant). In dehydration stress, the whole plant seedlings were dried on filter paper. In cold treatment, the plants were placed at 4 °C in a low-temperature light incubator, while in hot treatment, the plants were placed at 42 °C in a light incubator. Both of these treatments were executed under a 14/10h (day/night) photoperiod. Plants were maintained at these different treatments for a range of time periods (0, 1, 3, 6, 12 and 24 h). At each time point, seedlings were harvested and immediately frozen in liquid nitrogen. Control group plants were grown in parallel and were harvested at the same time points as those of the other treatment groups. All samples were stored at −80 °C for RNA extraction.
Expression analysis of ZmERD2
Total RNA was extracted from maize seedlings from different stress treatment conditions, using the Trizol reagent according to the manufacturer's protocol (Invitrogen). The first cDNA strand was generated using PrimeScript™ 1st Strand cDNA Synthesis Kit from TaKaRa Bio. The fluorescence dye method was employed for real-time fluorescent quantitative expression analysis. According to the full sequence information of gene ZmERD2, the fluorescent quantitative PCR primers were designed as follows: ZmERD2-F3, 5′-ACCACTTCGTCCA GGAGTTCAA-3′, and ZmERD2-R3, 5′-GCCCTCATACAGCGAGTCAATCT-3′. A maize actin-encoding gene was used as a control, with the following primer pair: Actin-F, 5′-CAGCAACTGGGATGATATGG-3′, and Actin-R, 5′-ATTTCGCTTTCAGCAGTGGT-3′. The reaction system (25 μL) was 2×SYBR qPCR Mix (12.5 μL), cDNA template (1 μL), 10 μmol/L forward primer (1 μL), 10 μmol/L reverse primer (1 μL) and ddH2O (9.5 μL). The reaction procedure was as follows: 3 min of pre-denaturation at 95 °C, 10 s at 94 °C, 30 s at 55 °C, 20 s at 72 °C, 40 cycles, and 5 min of extension at 72 °C. The data were obtained via Sequence Detector Version 1.3.1 and transformed to Microsoft Excel for analysis. The whole process was run in a Chromo4™ fluorescent quantitative PCR device (BioRad). All data were normalized to constitutively expressed ACTIN according to the 2−ΔΔCT method.[Citation20] Each experiment was repeated at least three times.
Data analysis
All data were expressed as mean values with standard error (x ± SE). The data were analyzed by one-way ANOVA and Duncan's multiple comparison analysis using SPSS 19.0 software. Values were considered significantly different at P < 0.05 for all experiments.
Results and discussion
cDNA cloning and sequence analysis of ZmERD2
A specific fragment with a length of about 2375 bp was obtained by RT-PCR amplification (). The fragment contained an open reading frame (ORF) of 1949 bp encoding an HSP of 649 amino acids (aa) with a deduced molecular weight of 71.163 kDa and a pI of 5.10. The deduced ZmERD2 protein was predicted to have no membrane-spanning helices and is presumably a non-membrane protein. Subcellular structure prediction results showed that the protein encoded by ZmERD2 may be located in the cytoplasm.
Figure 1. Full-length sequence of ZmERD2 amplified by RT-PCR. 1: PCR product, M: DNA molecular weight Marker III (TIANGEN).
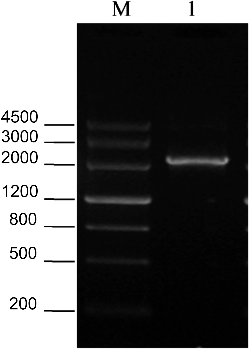
The amino acid sequence comparison revealed that there were three conserved signature motifs (): DLGTTYS (13–19 aa), IFDLGGGTFDVSLL (203–216 aa) and VVLVGGSTRIPRVQQ (340–354 aa) in ZmERD2, all of which were consistent with the structural characteristics of the Hsp70 family.[Citation21] In agreement with the subcellular structure prediction results, the predicted ZmERD2 protein was shown to have the cytoplasmic Hsp70 C-terminal motif EEVD (642–645 aa),[Citation13,Citation22] which indicated that the predicted ZmEDR2 protein is a cytoplamic Hsp70.
Figure 2. Deduced sequence alignment of ZmERD2 and homologous proteins of other eukaryotes. Underlined residues are three conserved signature motifs of eukaryotes: DLGTTYS, IFDLGGGTFDVSLL and VVLVGGSTRIPRVQQ; the C-terminus cytosolic Hsp70-specific motif (EEVD) is boxed.
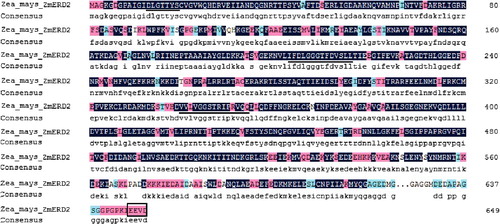
The predicted three-dimensional structure of ZmERD2 is shown in . The SWISS-MODEL template was 4fl9.1, the model range was 7–560 aa and the sequence similarity was 80.94%. shows that there is a high degree of spatial overlap between ZmERD2 and the template. The predicted ZmERD2 protein was also shown to possess three distinct domains of Hsp70s: an N-terminal adenosine triphosphatase (ATPase) domain, a substrate-binding domain and a highly variable C-terminal domain.
Homologous alignment and phylogenetic analysis of Hsp70s
The BLAST program analysis showed that the deduced amino acid sequence of the ZmERD2 shared 95% homology with the amino acid sequences of HSPs of Oryza sativa Indica group (Genebank: CAA47948), about 94% with those of Triticum urartu (EMS52605), Aegilops tauschii (EMT07406), Nicotiana tabacum (AAR17080) and Gossypium hirsutum (ACJ11742) and shared about 92%–93% similarity with other known Hsp70s, such as those of A. thaliana (NP195870), Dendrobium officinale (AGR45355), Medicago sativa (AAV98051), N. tabacum (EOY18179), Saussurea medusa (AAV97978), Ageratina adenophora (ACM42161) and Brassica napus (AAB88009), which indicated that Hsp70 is a conservative protein.
For further analysis, Clustal X and MEGA 5.10 were used to align multiple sequences and construct a neighbour-joining phylogenetic tree (). The phylogenetic tree consisted of two branches with Hsp70 of monocotyledons and dicotyledons. Among them, ZmERD2 was clustered together with cytosolic Hsp70s from A. tauschii (EMT07406) and T. urartu (EMS52605), both belonging to the graminaceous plants. Thus, the phylogenetic tree and the analysis of conserved motifs of the predicted ZmERD2 protein indicated that ZmERD2 is a cytoplasmic Hsp70.
Figure 4. Phylogenetic analysis of heat shock proteins in different plants: Aegilops tauschii (Tausch's goatgrass), Triticum urartu (red wild einkorn), Oryza sativa Indica group (hsien rice), Dendrobium officinale, Saussurea medusa, Ageratina adenophora (Eupatorium adenophorum), Nicotiana tabacum (tobacco), Medicago sativa (alfalfa), Brassica napus (rapeseed), Arabidopsis thaliana (thale cress), Theobroma cacao (cacao tree) and Gossypium hirsutum (upland cotton).
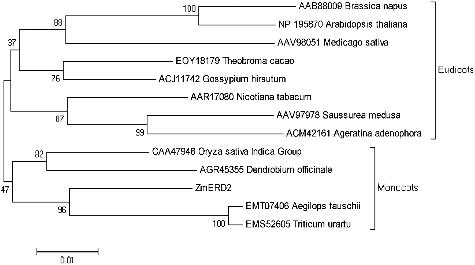
Cloning and cis-regulatory elements analysis of the ZmERD2 gene promoter region
The promoter sequence located about 2235 bp upstream of the ZmERD2 gene was cloned from genomic DNA of maize by specific amplification (). The cis-regulatory elements of the promoter predicted by PlantCARE included important core elements such as TATA-box and CAAT-box, which showed that the ZmEDR2 promoter region could initiate expression of ZmEDR2 (). There were also an A-box and other cis-regulatory elements responsive to heat, cold, dehydration, salicylic acid (SA) and jasmonate (JA). Thus, it was predicted that ZmEDR2 may be involved in signal transduction pathways related to stress resistance.
Figure 5. PCR analysis of the ZmERD2 promoter. 1: PCR product, M: DNA molecular weight Marker III (TIANGEN).
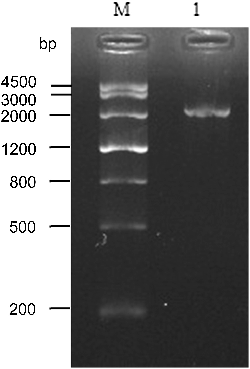
Table 1. Predicted cis-acting elements in the ZmERD2 gene promoter.
ZmERD2 gene expression under different types of abiotic stress
To further understand the function of the ZmERD2 gene in maize, the expression of ZmERD2 under several types of abiotic stress was analyzed at the transcript level (). The results showed that the expression patterns of the ZmERD2 gene treatments were different under different abiotic stress factors. The expression of the ZmERD2 gene was strongly induced upon heat stress and the highest relative expression was detected after 1 h exposure to heat, when it increased 40-fold compared to that in the control group. The expression then quickly began to decrease and was only 18-fold higher than the control level at 3 h; thereafter, the transcript level decreased to the normal level at 24 h but remained at elevated levels compared to the control group until 24 h. The transcript levels of the ZmERD2 gene were highest at 1 h, which indicated that it rapidly responds to heat stress. Different studies have confirmed that the rapid expression of Hsp70 genes and synthesis of their respective protein products can be induced and increased by heat stress. For example, in Arabidopsis, the expression of Hsp70 reached its peak after 1 h of heat stress at 47 °C and then decreased.[Citation23] In non-heading Chinese cabbage, the expression of BccpHSC70-2 was significantly upregulated after 4 h of heat stress at 38 °C and then decreased to normal level.[Citation24] Analysis of Hsp70 superfamily proteins of rice has shown that many Hsp70s could be induced by heat stress at 42 °C and the expression is significantly increased after 1 h of heat stress.[Citation25] This study also indicated that the expression of ZmEDR2 from maize was quickly induced and reached its peak after 1 h of heat stress at 42 °C. These data suggest that most of the higher plants are sensitive to heat stress and could improve their tolerance ability to heat stress through synthesis of stress-tolerance proteins.
Figure 6. Expression of ZmERD2 gene in maize seedlings under various types of stress. Different letters indicate significant differences at P < 0.05.
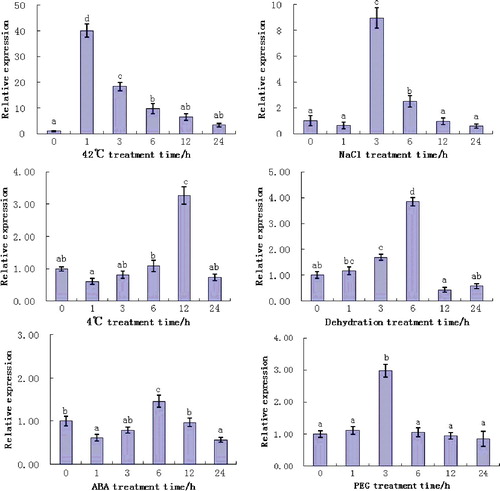
This study showed that the expression of ZmEDR2 could be induced and increased not only by heat stress, but also by cold, high salinity, PEG and dehydration. The expression pattern, however, was different under different stress factors. ZmERD2 expression could be induced by salt stress but the response to salt stress was not as rapid as that to heat stress. The expression of ZmERD2 gene was significantly upregulated after 3 h of exposure to salt stress and reached a peak which was nine-fold higher than the normal level; thereafter, the transcript level decreased to the normal level at 24 h. Under cold stress at 4 °C, the expression of ZmEDR2 reached a maximum at 12 h, when the expression was 3.3-fold higher than the normal level, which is consistent with the report of Liu et al. [Citation26]. Like in low-temperature (4 °C) stress, the ZmERD2 gene was also induced by dehydration and PEG stresses. The highest relative expression was detected at 6 and 3 h, respectively, of treatment and the increase was, respectively, up to about 3.8-fold and 2.7-fold higher compared to the control group. Under ABA stress, the highest expression of ZmEDR2 (1.5-fold higher than the normal level) was observed at 6 h. According to the report of Livak and Schmittgen,[Citation20] expression of a gene by induction should reach twice the normal level. Thus, our results suggest that the ZmERD2 gene may respond differently to different stress factors, such as high temperature, high salt, low temperature, PEG and dehydration, with different response times and expression levels. It might be possible to speculate that the differences in the upregulation patterns may reflect the involvement of different molecular pathways.
According to statistical data from the National Bureau of Statistics of China (http://data.stats.gov.cn/easyquery.htm?cn=C01), in 2012, maize was the food crop with the largest planting area and maximum yield in China. Therefore, development of maize is of strategic importance for national food security. At the same time, the effect of heat stress on the growth of maize has become more and more serious in recent years due to global warming. Heat stress throughout the whole growing period of maize could result in decrease of yield and quality.[Citation27,Citation28] Since HSPs are chaperones which could increase the tolerance ability to various stress conditions,[Citation29,Citation30] the results from this study that ZmEDR2 may be related to heat stress as well as to heat, cold, dehydration, PEG and high salinity could lay the basis for future in-depth molecular analysis of ZmEDR2 role and function. In order to confirm the effect of overexpression on plant resistance to stress, ZmEDR2 has been transformed into A. thaliana and tobacco (data not shown). Tobacco plants with integrated ZmEDR2 have been obtained and the gene is being transformed into A. thaliana. Further research will be focused on improvement of plant resistance to stress by overexpression of genes encoding Hsp70 with the aim to provide a theoretical basis for cultivation of stress-resistant maize varieties.
Conclusions
In this study, an HSP gene in maize, ZmERD2, was cloned and the deduced protein was predicted to contain an Hsp70 conservative structure domain, three typical Hsp70 family signature sequences and a cytosolic Hsp70-specific motif. According to the results obtained by real-time PCR, ZmEDR2 is especially sensitive to heat stress and may also play a role in plant responses to cold, dehydration, PEG and high salinity. These results will serve as a basis for further research focused on improvement of abiotic stress resistance by overexpression of Hsp70 genes with the aim to provide a theoretical basis for cultivation of stress-resistant maize varieties.
Disclosure statement
No potential conflict of interest was reported by the authors.
Additional information
Funding
References
- Sung DY, Guy CL. Physiological and molecular assessment of altered expression of Hsc70-1 in Arabidopsis: evidence for pleiotropic consequences. Plant Physiol. 2003;132:979–987.
- Cho EK, Hong CB. Molecular cloning and expression pattern analyses of heat shock protein 70 genes from Nicotiana tabacum. J Plant Biol. 2004;47:149–159.
- Wang W, Vinocur B, Shoseyov O, et al. Role of plant heatshock proteins and molecular chaperones in the abiotic stress response. Trends Plant Sci. 2004;9:244–252.
- Kiang JG, Tsokos GC. Heat shock protein 70 kDa: molecular biology, biochemistry, and physiology. Pharmacol Ther. 1998;80:183–201.
- Renner T, Waters ER. Comparative genomic analysis of the Hsp70s from five diverse photosynthetic eukaryotes. Cell Stress Chaperones. 2007;12:172–185.
- Sung DY, Kaplan F, Guy CL. Plant Hsp70 molecular chaperones: protein structure, gene family, expression and function. Physiol Plant. 2001;113:443–451.
- Hartl FU, Hayer-Hart M. Molecular chaperones in the cytosol from nascent chain to folded protein. Science. 2002;295:1852–1858.
- Meimaridou E, Gooljar SB, Chapple JP. From hatching to dispatching: the multiple cellular roles of Hsp70 molecular chaperone machinery. J Mol Endocrinol. 2009;42:1–9.
- Schroda M, Vallon O, Wollman F, et al. A chloroplast-targeted heat shock protein 70 (HSP70) contributes to the photoprotection and repair of photosystem II during and after photoinhibition. Plant Cell. 1999;11:1165–1178.
- Wang W, Vinocur B, Shoseyov O, et al. Role of plant heatshock proteins and molecular chaperones in the abiotic stress response. Trends Plant Sci. 2004;9:244–252.
- Ireland HE, Harding SJ, Bonwick GA, et al. Evaluation of heat shock protein 70 as a biomarker of environmental stress in Fucus serratus and Lemna minor. Biomarkers. 2004;9:139–155.
- Wang W, Vinocur B, Altman A. Plant responses to drought, salinity and extreme temperatures: towards genetic engineering for stress tolerance. Planta. 2003;218:1–14.
- Sung DY, Kaplan F, Guy CL. Plant Hsp70 molecular chaperones: protein structure, gene family, expression and function. Physiol Plant. 2001;113:443–451.
- Alvim FC, Carolino SMB, Cascardo JCM, et al. Enhanced accumulation of BiP in transgenic plants confers tolerance to water stress. Plant Physiol. 2001;126:1042–1054.
- Cho EK, Hong CB. Over-expression of tobacco NtHSP70-1 contributes to drought-stress tolerance in plants. Plant Cell Rep. 2006;25:349–358.
- Collins GG, Nie XL, Saltveit ME. Heat shock protein and chilling sensitivity of mung bean hypocotyls. Plant Physiol. 1993;89:117–124.
- Horton P, Park KJ, Obayashi T, et al. WoLF PSORT: protein localization predictor. Nucleic Acids Res. 2007;35:W585–W587.
- Emanuelsson O, Brunak S, Heijne GV, et al. Locating proteins in the cell using TargetP, SignalP and related tools. Nat Protoc. 2007;2:953–971.
- Tamura K, Peterson D, Peterson N, et al. MEGA5: molecular evolutionary genetics analysis using maximum likelihood, evolutionary distance, and maximum parsimony methods. Mol Biol Evol. 2011;28:2731–2739.
- Livak KJ, Schmittgen TD. Analysis of relative gene expression data using real-time quantitative PCR and the 2−ΔΔCT method. Methods. 2001;25:402–408.
- Karlin S, Brocchieri L. Heat shock protein 70 family: multiple sequence comparisons, function, and evolution. J Mol Evol. 1998;47:565–577.
- Liu S, Zhang P, Cong B, et al. Molecular cloning and expression analysis of a cytosolic Hsp70 gene from Antarctic ice algae Chlamydomonas sp. ICE-L. Extremophiles. 2010;14:329–337.
- Hara M, Kurita I. The natural alkaloid sanguinarine promotes the expression of heat shock protein genes in Arabidopsis. Acta Physiol Plant. 2014;36:3337–3343.
- Song HB, Song XM, Liul HH, et al. cDNA clones and expression analysis of cpHSC70and mtHSC70 in non-heading Chinese cabbage. Plant Mol Biol Rep. 2014;32:531–540.
- Neelam K, Sarkar P, Kundnani AG. Functional analysis of Hsp70 superfamily proteins of rice (Oryza sativa). Cell Stress Chaperones. 2013;18:427–437.
- Liu SK, Wang J, Cong BL. Characterization and expression analysis of a mitochondrial heat-shock protein 70 gene from the Antarctic moss Pohlia nutans. Polar Biol. 2014;37:1145–1155.
- Zhang BR, Dong ST, Hu CH, et al. [The effect of high temperature on starch synthesis and yield of maize]. Acta Agron Sin. 2007;33(1):38–42. Chinese.
- Zhao LF, Li HC, Liu TY, et al. [Effects of high temperature during flowering on photosynthetic characteristics, yield and quality of different maize genotypes]. 2012;45(23):4947–4958. Chinese.
- Morris JP, Thatje S, Hauton C. The use of stress-70 proteins in physiology: a re-appraisal. Mol Ecol. 2013;22:1494–1502.
- Mayer MP, Bukau B. Hsp70 chaperones: cellular functions and molecular mechanism. Cell Mol Life Sci. 2005;62:670–684.