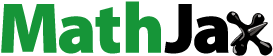
ABSTRACT
Macrophomina phaseolina (Tassi) Goid remains the prevailing causal agent of charcoal rot disease that significantly suppresses the yield of a variety of oilseed crops. Its wide host range and ability to survive under arid conditions, coupled with the ineffective use of fungicides against it, have spurred scientific endeavours for alternative avenues to control this phytopathogen. Hence, the present study aimed to provide empirical evidence of the efficacy of three fungal isolates (T2, T10 and T12) of Trichoderma harzianum as biological control agents against charcoal rot in soybean (Glycine max L.). The results of the in vitro studies revealed that all three fungal isolates significantly inhibited the growth of M. phaseolina phytopathogen, with T12 showing considerably higher inhibition effect than T2 and T10 isolates. T12 inhibited the growth of M. phaseolina in the dual culture (72.31%) and volatile production (63.36%) assays, and the hyperparasitism test indicated cell lysis following the interactions with T12 mycelia. T12 isolate was mostly effective in field experiments, observable in the attained minimum plant disease indices both in the soil incorporation (11.98%) and seed inoculation (5.55%) treatments, in comparison to isolates T2 and T10. Moreover, the stem and root lengths, as well as the seed weight, were considerably increased, as compared to the control. Hence, the findings reported in the present study supported the applicability of T12 isolate as possible alternative to fungicides for the control of charcoal rot in soybean.
Introduction
Macrophomina phaseolina (Tassi) Goid is an important root pathogen that causes charcoal rot in over 500 plant species throughout the world.[Citation1] This disease has caused economically important yield losses of various oilseed plants, such as corn (Zea mays L.), sorghum (Sorghum bicolor), sunflower (Helianthus annuus L.), cotton (Gossypium herbaceum L.), sesame (Sesamum indicum L.) and soybean (Glycine max L.).[Citation1] Moreover, studies have indicated that the reductions in yields of various crops in the United States alone attributable to charcoal rot were 1.98, 0.28 and 0.49 million metric tonnes in the years 2003, 2004 and 2005, respectively.
Moreover, the variable severity of charcoal rot infestations has been associated with environmental changes. Also, the susceptibility of various plants to infection by M. phaseolina has been described to be particularly elevated during drought and water stress.[Citation1,Citation2] Such high incidence of this disease during hot growing seasons would be due to reactions of M. phaseolina towards water stress, heat and high temperatures.[Citation3] These conditions tend to promote deeper penetration of the fungal mycelia into the host in search of nutrients, subsequently causing severe structural damage [Citation1] and fatality of the infected plants.[Citation2] This situation has been further exacerbated by the fact that the charcoal rot pathogen M. phaseolina has a wide host range, which extends over 75 plant families, including mono- and dicotyledons [Citation4] and more than 500 plant species worldwide.[Citation1] Such adaptability advocates great variability in pathogenicity and morphology among M. phaseolina isolates, as previously reported,[Citation5,Citation6] strongly suggesting that it is a highly accomplished crop pathogen and crop rotation strategy to control its spread may not be effective.[Citation7]
The extent of M. phaseolina infection in plants may result in both pre- and post-emergence plant mortalities, with most conspicuous symptom seen as the ‘black leg’, owing to the formation of sclerotia in the plant crown. Other characteristics of these symptoms also include presence of spindle-shaped lesions with dark border and light grey centre, covered with small pinhead-sized microsclerotia and sometimes pycnidia.[Citation8] Moreover, plants infected with charcoal rot generally have weak appearances and colourized leaves with fewer black secondary roots.[Citation9] Infected plants eventually die from exposure to phytotoxic metabolites released by M. phaseolina, such as phaseolinone, as well as other complications, such as vascular blockages that compromise nutrient transport.[Citation10,Citation11] The ability of M. phaseolina to propagate by forming sclerotia further increases their robustness and survivability for prolonged duration in the soil,[Citation9] even as long as 15 years as a saprophyte.[Citation8] Such extraordinary adaptability of M. phaseolina originates in its significant physiological,[Citation12] morphological,[Citation13] pathogenic and genetic diversity that has resulted in its wide distribution across diverse climatic conditions, ranging from arid to tropical regions.[Citation14,Citation15]
To date, various disease management methods, viz. cultural, regulatory, physical, chemical (fungicides) and biological have been implemented to combat and eradicate the phytopathogenic fungi. However, these methods are effective only when employed well in advance as precautionary measures.[Citation16] Moreover, the soil-borne nature of the charcoal rot disease, caused by M. phaseolina, means that the conventional use of chemical fungicides for the control of M. phaseolina infections may be less effective [Citation17] and may disrupt the balance of beneficial microbes in the soil; also, these fungicides are expensive and economically not affordable to low-income farmers.[Citation18] Apart from environmental detrimental threats and health hazards, the continuous use of fungicides and pesticides may backfire and give rise to resistant strains.[Citation19] In an effort to alleviate the dependence on chemical fungicides, the use of rhizophere Trichoderma species (fungi), i.e. Trichoderma harzianum, for controlling the spread of M. phaseolina in agronomical crops, has been suggested. Considering the adverse effects of conventional chemical treatments, the beneficial roles of T. harzianum isolates may play in eco-friendly agricultural systems and in the need to sustain the supply chain of food for human consumption; concerted efforts in providing alternative economical resources to control the invasive M. phaseolina merits special consideration.
The present study aimed at utilizing locally isolated T. harzianum as a sustainable means to manage and control the soil-borne charcoal rot disease caused by M. phaseolina in the surrounding agricultural areas of Mazandaran.
Materials and methods
Sampling and collection of fungal isolates
Samples were collected from infected soybean (Glycine max L.) root and crown segments randomly selected from different areas of Iran. They were thoroughly cleaned and washed superficially with sodium hypochlorite (1%) solution for 2 min. The samples were placed on potato dextrose agar (PDA, Oxoid Ltd, England) and were incubated in darkness at 30 ºC. The M. phaseolina fungal colonies that appeared after 24−48 h were purified by hyphal-tip isolation technique,[Citation18] and a single colony was selected for morphological determination using the identification key previously described by Chang et al.[Citation20] The colonies of T. harzianum (T2, T10 and T12) fungal antagonists were obtained from soil samples collected from various areas in the Mazandaran Province, Iran.
Laboratory assessments of antagonistic mechanisms
Dual culture technique
The PDA was inoculated at 1 cm distance from the edge of the Petri dish with a 5-mm mycelial disc cut from the leading edge of an active 5-day-old colony of M. phaseolina and T. harzianum antagonistic isolates. The plates were incubated at 28 ºC for 4 d and the diameter of the inhibition zones of M. phaseolina growth, due to antagonistic activity, was measured in centimetres. The growth inhibition percentage (GIP) was expressed in terms of inhibition percentage of radial growth of the phytopathogen, i.e. by comparing with control plates without the presence of T. harzianum disc. The GIP (%) was calculated as described by Monte and Liobell [Citation21] by using the following equation:(1)
(1) where GIP, GT and GC represent the growth inhibition percentage and fungus growth (diameter of colony growth in mm) in treated and control plates, respectively. Inhibition zones of 10 mm or more were considered important and at 30 mm, the inhibition of M. phaseolina was total. The experiment was carried out based on completely randomized design (CRD) with three replications, and Mstat-c software was employed for the statistical analysis.[Citation6]
Volatile metabolites production test
Effectiveness of possible volatile metabolites produced by T. harzianum against M. phaseolina was monitored according to a method, previously described by Fernando et al.[Citation22] A 5-mm disc cut out from the growing edge of fungal antagonists and phytopathogen colonies were placed in the centre of several PDA plates. The PDA plates that contained the phytopathogen discs were inverted over the antagonist and control plate and separated by parafilm (Parafilm “M”, Bemis Flexible Packaging, Oshkosh, WI, USA). The plates were incubated for 4 d at 28 ºC and the zones of inhibition that appeared were measured to obtain the GIP (%).
Hyperparasitism test
For the hyperparasitism tests, sterile glass blank slides were placed on plates containing 10 mL of water and 2% agar, and were covered with a thin layer of PDA. Then, 5-mm discs were cut out from the active parts of the phytopathogen and the antagonist colonies and the discs were both placed at 1-cm interval on the long ends of the glass slide, followed by incubation at 26 ± 1 ºC. Parasitism behaviour, growth inhibition and interaction of antagonist with the phytopathogen were studied microscopically (Nikon Eclipse E200, Japan) after 48 and 72 h of incubation, respectively.[Citation3] The experiments were performed with two treatments and three replications in a CRD. All the above studies were performed under in vitro conditions.
Biological control field assessment
Investigation of sclerotia population of M. phaseolina in soil
Field soil samples from different farms in major agricultural production zones (Ghaemshahr, Neka, Behshahr, Sari, Jooybar, Kiakola) in Iran were collected from soil depth up to 10 cm. Isolation and population estimation of the fungus were carried out according to the method described by McCain and Smith.[Citation23] The air-dried soil samples were sieved through 2 mm in diameter pores. A sample of the sieved soil (1 g) was sterilized in sodium hypochlorite solution (0.5%) for 10 min. The soil was rinsed with sterile distilled water through a 325-mesh sieve and transferred into a conical flask containing sterile distilled water (200 mL). A portion of the liquid (100 mL) was transferred into melted PDA (55 °C) supplemented with streptomycin sulphate (Sigma-Aldrich) (0.5 mL, 5%) and sodium hypochlorite (Sigma-Aldrich) (0.13 mL, 0.5%). The suspension was shaken by hand, poured into seven sterile Petri dishes and incubated in darkness at 30 °C. After a stipulated period of 96 h, the emerged fungal colonies of M. phaseolina were counted and the data were converted into colonies per 1 g of soil. The chemical and physical properties of selected soil samples were also carried out by the method of Steffens et al.[Citation24]
Soil chemical and physical properties
The soil samples collected during fieldwork were kept in brown paper bags and stored at ambient temperature. Moist samples were air dried at ambient temperature for 4 d to 5 d before storage. The soil samples were sent to the Agricultural and Natural Resources Research Center of Mazandaran for chemical analysis. Soil samples were air dried at room temperature for 2 d in aluminium dishes before sieving through a 2-mm sieve. Each soil sample was further divided into two portions –laboratory and original subsamples. The laboratory subsamples were placed in labelled plastic bags and analysis of the soil samples was carried out in order to measure the following soil chemistry: total neutralization value (TNV, %), organic carbon in percentages (OC), Ph, electrical conductivity in dS/m (ECe), soil mineral composition (mg/kg) (N, P, K, Fe, Mn, Zn and Cu) and soil texture (%) (clay, silt and sand).[Citation25]
Efficacy assessment of T. harzianum antagonists in soil and seedling treatments
The propagation of T. harzianum fungi was initiated by their addition to the soil in the form of fermented wheat and rice straw (1:1; w:w) at the rate of 50 g/m2 soil.[Citation24] The mixed straw was autoclaved for 1 h at 121 °C, kept for 10 d under greenhouse conditions and regularly watered to ensure complete fermentation. The mixed straw (150 g) was transferred into 1-L jar and autoclaved twice at 24 h intervals at 121 °C. The fermented straw was inoculated with seven-day-old antagonist conidial suspension and kept under fluorescent light for four weeks at 24 °C. The suspensions were diluted to a concentration of 3.5 × 109 conidia per cm3 prior to soil inoculation and the rate of application in the field plots was set at 50 mL/m2. For the seedling treatments, the three T. harzianum isolates were used to treat soybean seeds, according to a previously described method by Gemell et al.[Citation26] The used control was pathogen and no biocontrol agents. One kilogram of soybean was coated with slurry (40 mL) prepared by mixing 10 g of T. harzianum inoculants with 30 mL (40%) gum arabic (acacia gum) solution (Nexira®).
The efficacy of each treatment was evaluated on the basis of the plant disease index (PDI), which measures the intensity of the disease [Citation27] at certain stages of the plant growth. In this study, the R7 stage (yellowing of the leaves and yellow pods at 50% growing stage) of soybean growth was chosen and the development of the charcoal rot disease from crown to stem following removal of the epidermis was measured in percentages by using a method reported by Agarwal and Sinclair [Citation28] and the data tabulated. EquationEquation 1(1)
(1) was used for calculating the PDI (%).[Citation29] In percentage disease assessment, the diseased area is assessed up to 50% and the healthy area above 50%. In the grading system proposed by Agrawal and Sinclair,[Citation28] the rating values are as follows: 0 = no symptoms in plant stem; 1 = 1%–5% symptoms in plant stem; 2 = 5%–15% symptoms in plant stem; 3 = 15%–50% symptoms in plant stem; 4 = more than 50% symptoms in plant stem and the maximum rating value is 4 (more than 50% symptoms in plant stem) [Citation30]:
(2)
(2) where PDI is the plant disease index and is defined as the percentage ratio of the sum of disease rating and production of the total number of plants and maximum rating value.
Measurement of stem length, root length and seed weight
The experiment was carried out based on CRD in four replications. The soybean seeds were sown in plots of three rows that consisted of five rows (5 m length × 40 cm width) and 8 cm intra- and inter-rows per replication. To prevent the transfer of fungi between the treated plots, each plot in this study was well separated by several other empty plots. The PDI, root and stem length (cm), as well as seed weight (g) were measured from 25 candidate plants selected from each replication and were statistically analysed by using the Mstat-c V 1.42 statistical software (Michigan State University, version 1.42). The differences between treatments were evaluated by analysis of variance (ANOVA) and the obtained means were compared using the Duncan's multiple range test (DMRT) at 5% probability level.
Results and discussion
Sampling and collection of fungal isolates
The detrimental effects on the environment and the low efficacy of the fungicides against M. phaseolina have incited efforts among the scientific community to search for other safer and sustainable alternatives to control this fungal phytopathogen. In this context, the use of natural biological control agents, i.e. soil micro-organisms, as a primary defence line, may prove useful for scientific and agricultural settings.[Citation31] The review of literature has indicated a variety of microbes, such as Bacillus spp. and Pseudomonas spp. bacteria [Citation32] and Trichoderma spp mycoparasitic fungi,[Citation3,Citation19] as potential antagonists against the M. phaseolina phytopathogen.[Citation33] The present study successfully isolated 12 colonies of T. harzianum fungus from soil samples, collected from various areas in the Mazandaran Province of Iran. The samples were assessed for their effectiveness as biological control agents against M. phaseolina. The preliminary results obtained in this study indicated that only three isolates of T. harzianum (T2, T10 and T12) considerably inhibited the growth of M. phaseolina in the in vitro assays, hence only the three isolates were utilized in subsequent assessments.
Laboratory assessments of antagonistic mechanisms of T. harzianum isolates
Dual culture technique
It was revealed in the in vitro assays that the growth rates of T2, T10 and T12 isolates exhibited growth inhibition of M. phaseolina and showed exceptional hyperparasitism against the colonies of M. phaseolina. The observed results from the dual culture tests are shown in and . Evaluation of the inhibition zones surrounding the T12, T2 and T10 isolates revealed inhibition of the M. phaseolina by as much as 72.31%, 45.23% and 44.13%, respectively. A closer examination revealed significant growth of the T. harzianum hyphae over the pathogen disc and new colonies of the antagonists had ventured and overrun the colonies of the phytopathogen (). Such observations is in concurrence with previously reported isolates of T. harzianum (T100) and Bacillus sp., used to control the charcoal root rot.[Citation34] However, the observable differences between the antagonistic behaviour of the fungal isolates (T12, T2 and T10) seen here may be due to the influence of various factors, such as humidity and structure of the soil, as well as other uncontrolled environmental conditions.[Citation34]
Table 1. Inhibition percentage of radial growth of M. phaseolina colonies affected by T. harzianum isolates in the in vitro dual culture and volatile production tests.
Volatile metabolite production test (VMPT) and hyperparasitism test
The results showed that all three isolates (T2, T10 and T12) were effective in inhibiting the growth of M. phaseolina, which was possibly attributed to the liberation of volatile compounds [Citation20,Citation35] capable of inhibiting the growth of the phytopathogen ( and ). Interestingly, the volatiles produced by T12 isolate (63.36%) were more effective in suppressing the growth of M. phaseolina than those of T2 (51.31%) and T10 (43.30%) (). Similarly, T12 isolate also showed positive antagonistic behaviour in the hyperparasitism tests, observable from its mycelial interactions with that of the M. phaseolina, which disrupts the cell wall of the phytopathogen cells (). According to the literature, the antagonistic properties of T. harzianum may be ascribed to the production of a number of volatile substances, which include acetaldehyde, isocyanide derivatives, terpene derivatives, derivatives of alpha-piron, hydrazone derivatives, piperazine, polyketides, alcohols, lactones and 6-pentyl-2H-pyran.[Citation20] Moreover, the positive mycelial growth of T12 isolate towards M. phaseolina may be attributable to the attraction towards certain chemicals/toxins released by M. phaseolina.[Citation36] Furthermore, the distinctively larger inhibition zone surrounding the mycelia of the T12 seen in this study () strongly implied liberation of higher amount of antibiotic that led to the observed inhibition. This is one of the many key criteria considered by scientists, when bioprospecting for potent biological control agent [Citation19] to control M. phaseolina. The distinctive clear zones surrounding the mycelia of T12 is in consistency with the prevailing findings of previous researchers.[Citation37,Citation38] Hence, it can be construed that the differences in the inhibition zone diameters are due to antibiotic production between the isolates (T2, T10 and T12) and may be strain dependent. This is consistent with the descriptions made by previous studies.[Citation37,Citation38] Thus, the obtained results prove that the proper selection of an effective antagonist strain is fundamental for controlling the spread of charcoal rot disease caused by M. phaseolina. Considering the larger inhibition zone surrounding the T12 isolate, its use as biological control to manage such disease appears to be adequate and suitable for the subsequent field study.
Biological control assessment in the field
Field experiments: plant disease index
The intensity of the charcoal rot disease was assessed by the methods of PDI, growth parameters (stem length and root length) and seed weight. Valiente et al. [Citation39] described that volatile compounds are conveniently effective in inhibiting the growth of M. phaseolina due to their extensive range of penetration into the soil pores, thereby better inhibiting the growth of mycelia and sclerotium of the phytopathogen. However, the seed inoculation performed noticeably better than the soil incorporation technique, which concurred with a previous study reporting that certain antagonists promoted the growth of plants, in addition to inhibiting the phytopathogen.[Citation39] The reason may closely be associated with the time factor needed for growth and establishment of T. harzianum in the soil, before any significant inhibitory effect of the antagonist on M. phaseolina is visibly noticed.[Citation39] Furthermore, different species of Trichoderma sp. have been found effective in protecting the root system in a number of crops against other types or strains of pathogenic fungi, e.g. Fusarium solani and M. phaseolina.[Citation40] It was apparent that the population of M. phaseolina microsclerotia was considerably varied in different areas of Mazandaran Province. The highest and lowest numbers of sclerotia were observed in soil samples from Jooybar (32 per 1 g of soil) and Ghaemshahr (14 per 1 g of soil), respectively (). The corresponding physical and chemical properties of the soils are described in . It is pertinent to highlight that the presence of 32 sclerotia per gram of soil is regarded as a considerably dense M. phaseolina infection. Considering the high M. phaseolina sclerotia population in the soil samples of Jooybar, the location was selected for the subsequent field studies.
Table 2. Sclerotia population of M. phaseolina pathogen in different regions of Mazandaran Province.
Table 3. Soil physical and chemical properties from the experimental field (Jooybar).
Measurement of stem length, root length and seed weight
The changes in the root structure of soybean plant, attributable to the infection by charcoal rot pathogen, tend to adversely affect the proper functioning of the root, hence resulting in stunted growth.[Citation41] In view of such changes, it is vital that measurements of the stem and root length, as well as seed weight of the infected soybean plant, are made in order to assess the extent of the damages, caused by the disease. The results revealed that field plots treated with suspensions of T. harzianum (T2, T10 and T12) showed improved root and stem lengths, as well as a general increase in seed weight. Interestingly, considerable difference in growth parameters was seen in plots treated with T12 suspension, when compared to those treated with T2 and T10 suspensions, relative to the control. and outline the corresponding ANOVA and means comparison results for the stem and root lengths, as well as seed weight of the soybean plants in the field study. Most importantly, the significant stem lengths observed in all plot treatments, coupled with a large coefficient of variance (CV% = 10.0312) inferred prevalence of significant inhibitory variability between the T. harzianum isolates (T2, T10 and T12) utilized in this study (). Remarkably, it was observed that there were large and significant differences in the stem lengths of the treated plants ranging between 65.27 and 87.38 cm (). Likewise, the ANOVA of the PDI was also significant among the treatments (T2, T10 and T12), as compared to the control (). A noteworthy decline in the PDI in comparison to the soil incorporation technique is illustrated with the attainment of the lowest PDI in the soil (11.98%) and seed inoculation (5.55%) treatments, as compared to the control (49.06%) (). Therefore, the results obtained here strongly demonstrated the applicability of all three isolates (T2, T10 and T12), as effective biological control agents in the management of charcoal rot under actual agricultural settings.
Table 4. Analysis of variance for the field assessment studies on the efficacy of T. harzianum isolates as biological control agents against M. phaseolina charcoal rot pathogen.
Table 5. Evaluation of the efficacy of T. harzianum isolates as biological control agents against M. phaseolina in the soil incorporation and seed inoculation techniques.
Assessment of efficacy of antagonists in soil and seedling incorporation
Although the study showed that all three isolates (T2, T10 and T12) could inhibit the growth of the M. phaseolina phytopathogen, it was evident that the T2 isolate was the least effective, whereas T12 showed the highest inhibitory effect. Such inference was made following the evaluation of the stem, root and grains between the control and plants obtained from the field treatments when T12 suspension was used as seed inoculation (). The structure of the stem, root and seeds reflected the comparative PDI between the treated plants (T12 seed inoculation) and control. Once again, it was revealed that the seed inoculation technique yielded better results as compared to the soil incorporation technique, because plots treated with T12 showed significant maximum increase in stem length (). Significant increase in root length (23.04–26.68 cm) and seed weight was also seen in both techniques, as compared to the control (). The study successfully demonstrated that the application of T12 suspension improved the root length and seed weight more significantly than other isolates, thereby strongly suggesting its higher efficacy in managing charcoal rot disease.
The perceived reduction in disease intensity seen in the low PDI and improved growth parameter was in concurrence with previous studies.[Citation26,Citation41] Based on the results from the in vitro and field conditions, it appeared that the T12 isolate of T. harzianum may potentially be a promising biocontrol agent, thereby envisaging its use as an environment-friendly alternative to combat charcoal rot in soybean. It is believed that the effective T. harzianum T12 isolate may be used in the soil incorporation techniques or in combination with other cultural methods for disease management during the dry growing season. In this perspective, future studies, utilizing the bioactive compounds derived from T12 isolate, viz. enzymes β-glucosidase and cellobiose, as active components in biopesticide should be done; an important aspect in the green and sustainable approach of in situ biocontrol of M. phaseolina merits special consideration.
Conclusions
Having in mind the valuable roles of Trichoderma isolates as eco-friendly biological control agents in agricultural systems, the use of effective isolates in integrated pest management programmes may be more preferable. It is taken into consideration that over the course of evolution, the local Trichoderma sp. would develop special adaptive antagonistic mechanisms that would specifically target other competing phytopathogenic fungi that are typically found in its environment. In this perspective, development of novel charcoal rot green disease management strategies in the Mazandaran Province, by identifying the most effective local T. harzianum fungal isolates (T2, T10 and T12), deserves pertinence. In the explicit case of the Mazandaran Province, all three isolates, T2, T10 and T12, demonstrated adequate antagonistic behaviour against M. phaseolina; however, T12 was evidently the most effective. Therefore, this study concurred that the application of T12 isolate controlled charcoal rot caused by M. phaseolina, is beneficial. T12 isolated in this study appeared to have evolved specific adaptation, possibly by producing bioactive compounds to control the growth of M. phaseolina. Such inference was made from the consistency of T12 to exhibit the highest inhibition of the fungal phytopathogen in all evaluations.
Acknowledgments
The Agricultural and Resource Research Center of Mazandaran (Iran) is acknowledged for providing the soybean seeds, antagonistic fungus, T. harzianum and for the technical support during this study.
Disclosure statement
No potential conflict of interest was reported by the authors.
Additional information
Funding
References
- Rayatpanah S, Nanagulyan SG, Ala SV, et al. Pathogenic and genetic diversity among Iranian isolates of Macrophomina phaseolina. Chil J Agric Res. 2012;72:40–44.
- Singh N, Pandey P, Dubey RC, et al. Bological control of root fungus Macrophomina phaseolina and growth enhancement of Pinus roxburgii (Sarg) by rhizosphere compentent Bacillus subtilis BNI. World J Microb Biot. 2008;24:1669–1679.
- Vinale F, Sivasithamparam K, Ghisalberti EL, et al. Trichoderma–plant–pathogen interactions. Soil Biol Biochem. 2008;40:1–10.
- Jana T, Sharma T, Prasad RD, et al. Molecular characterization of Macrophomina phaseolina and Fusarium species by a single primer RAPD technique. Microbiol Res. 2003;158:249–257.
- Rayatpanah S, Nanagulyan SG, Alavi SV, et al. Phenotypic variations among isolates of Macrophomina phaseolina from different hosts in Northern Iran. Aust J Agric Resour. 2009; 3(3):2908–2913.
- Fernandez RB, De Santiago A, Delgado SH, et al. Characterization of Mexican and non-Mexican isolates of Macrophomina phaseolina based on morphological characteristics, pathogenicity on bean seeds and endoglucanase gene. J Plant Pathol. 2006; 88:1–8.
- Jalil S, Sadaqat HA, Tahir HN, et al. Response of sunflower under charcoal rot (Macrophomina phaseolina) stress conditions. Int J Sci Eng Res. 2013; 1:2347–3878.
- Kaur S, Dhillon GS, Brar SK, et al. Emerging phytopathogen Macrophomina phaseolina: biology, economic importance and current diagnostic trends. Crit Rev Microbiol. 2012; 1–16.
- Baird RE, Watson CE, Scruggs M. Relative longevity of Macrophomina phaseolina and associated mycobiota on residual soybean roots in soil. Plant Dis. 2003;87:563–566.
- Ndiage M. Ecology and management of charcoal rot (Macrophomina phaseolina) on cowpea in the Sahel [dissertation]. Netherlands: Wagening en University; 2007.
- Abbas HK, Baird RE, Wadl PA, et al. Microsatellites from the charcoal rot fungus (Macrophomina phaseolina). Mol Ecol. 2009;9:946–948.
- Mahdizadeh V, Safaie N, Mohamadi Goltapeh E. Diversity of Macrophomina phaseolina based on morphological and genotypic characteristics in Iran. Plant Pathol. 2011;27:128–137.
- Mayek-Perez N, Garcia-Espinosa RC, Lopez-Castan JA, et al. Water relations, histopathology, and growth of common bean (Phaseolus vulgaris L.) during pathogenesis of Macrophomina phaseolina under drought stress. Physiol Mol Plant Pathol. 2002;60:185–195.
- Srinivasan A, Wickes BL, Romanelli AM, et al. Cutaneous infection caused by Macrophomina phaseolina in a child with acute myeloid leukemia. J Clin Microbiol. 2009;47:1969–1972.
- Reyes-Franco MC, Hernandez-Delgado S, Beas-Fernandez RM, et al. Pathogenic variability within Macrophomina phaseolina from Mexico and other countries. J Phytopathol. 2006;154:447–453.
- Ganeshamoorthi P, Anand T, Saravanan A, et al. Cultural and genetic variability in Macrophomina phaseolina (Tassi.) Goid and incidence of mulberry root rot. Arc Phytopathol Pfl. 2010;20:123–132.
- Anis M, Abbasi W, Zaki MJ. Bioefficacy of microbial antagonists against Macrophomina phaseolina on sunflower. Pak J Bot. 2010;42:2935–2940.
- Aboshosha SS, Attaalla SI, EL-Korany AE, et al. Characterization of Macrophomina phaseolina isolates affecting sunflower growth in El-Behera governorate. Egypt J Agric Biol. 2007;6:807–815.
- Afouda LCA, Schulz D, Wolf G, et al. Biological control of Macrophomina phaseolina on cowpea (Vigna unguiculata) under dry conditions by bacterial antagonists. Int J Biol Chem Sci. 2012;6:5068–5077.
- Chang KF, Hwang SF, Wang H, et al. Etiology and biological control of Sclerotinia blight of coneflower using Trichoderma spesies. Plant Pathol J. 2006;5:15–19.
- Monte E, Liobell A. Trichoderma in organic agriculture. In: Proceeding V World Avocado Congress (Actas V Congreso Mundial Del Aquacade). 2003 Oct 19; Granada-Malaga (Spain). p. 725–733.
- Fernando D, Ramarathnam WG, Krishnamoorthy RA, et al. Identification and use of potential bacterial organic antifungal volatiles in biocontrol. Soil Biol Biochem. 2005;37:955–964.
- McCain AH, Smith RS. Quantitative assay of Macrophomina phaseolina from soil. J Phytopathol. 1972;62:1098.
- Steffens M, Kölbl A, Totsche KU, et al. Grazing effects on soil chemical and physical properties in a semiarid steppe of Inner Mongolia (PR China). Geoderma. 2008:143:63–72.
- Bashour II, Sayegh, AH. Methods of analysis for soils of arid and semiarid regions. Rome: Food and Agriculture Organization of the United Nations, American University of Beirut; 2007.
- Gemell LG, Hartley EJ, Herridge DF. Point-of-sale evaluation of preinoculated and custom-inoculated pasture legume seed. Aust J Exp Agric. 2005;45:161–169.
- Etebarian HR. Evaluation of Trichoderma isolates for biological control of charcoal stem rot in melon caused by Macrophomina phaseolina. J Agric Sci Technol. 2006;8:243–250.
- Agarwal VK, Sinclair JB. Principles of seed pathology. 2nd ed. Boca Raton (FL): CRC Press, Inc.; 1996.
- Manici LM, Cerato C, Caputo F. Pathogenic and biological variability of Macrophomina phaseolina (Tassi) Goid isolates in different areas of sunflower cultivation in Italy. Proc Int Sunflower Conf. 1992;1:779–784.
- Hossain MT, Hossain SMM, Bakr MK, et al. Survey on major diseases of vegetable and fruit crops in Chittagong region. Bangladesh J Agric Res. 2010;35:423–429.
- Abdullah MT, Ali NY, Suleman P. Biological control of Sclerotinia sclerotiorum (Lib.) de bary with Trichoderma harzianum and Bacillus amyloliquefaciens. Crop Prot. 2008;27:1354–1359.
- Hajieghrari B, Torabi-Giglou M, Momammadi M, et al. Biological potential of some Iranian Trichoderma isolates in the control of soil borne plant pathogenic fungi. Afr J Biotechnol. 2008;7:967–972.
- Harman GE, Howell CR, Viterbo A, et al. Trichoderma species – opportunictic, avirulant plant symbionts. Nat Rev Microbiol. 2004;2:43–56.
- Vasebi Y, Alizadeh A, Safaie N. Biological control of soybean charcoal root rot disease using bacterial and fungal antagonists In Vitro and greenhouse condition. J Crop Prot. 2013;2:139–150.
- Rayatpanah S, Alavi V, Arab G. Reaction of some soybean advanced lines to charcoal rot diseases, Macrophomina phaseolina (Tassi) Goid, in east Mazandaran. Iran J Agric Res. 2007;23:181–189.
- Senthilkumar M. Studies on the biocontrol mechanisms of soybean bacterial endophytes on charcoal rot fungus Rhizoctonia bataticola [dissertation]. India: Indian Agricultural Research Institute; 2006.
- Landa BB, Navas-Cortès JA, Jimenez-Diaz RM. Biological control of Macrophomina phaseolina on cowpea (Vigna unguiculata). Int J Biol Chem Sci. 2012;94:946–960.
- El-Fiki A, Mohamed FG, Hl-Deeb AA, et al. Some applicable methods for controlling sesame charcoal rot disease (Macrohomina phaseolina) under greenhouse conditions. Egypt J Phytopathol. 2004;32:87–101.
- Valiente C, Diaz K, Gacitua S, et al. Control of charcoal root rot in Pinus radiate nurseries with antagonistic bacteria. World J Microb Biotechnol. 2008;24:557–568.
- Malik G, Dawar S. Biological control of root infecting fungi with Trichoderma harzianum. Pak J Agric Sci. 2003;35:971–975.
- Pearson CAS, Schwenk FW, Crowe FJ, et al. Colonization of soybean roots by Macrophomina phaseolina. Plant Dis. 1984;68:1086–1088.