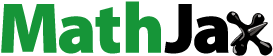
ABSTRACT
Biological control is a novel approach in crop protection. Bacteria, such as Bacillus spp. and Pseudomonas spp., are reported for this purpose and some of their products are already commercially available. In this study, the rhizosphere and phyllosphere of healthy cotton plants were used as a source of bacterial isolates with properties of potential biocontrol agents. The isolates were screened for phosphate solubilization activity, indole acetic acid (IAA) production and antifungal activity. Two isolates, S1HL3 and S1HL4, showed phosphate solubilization and IAA production simultaneously, while another two, JS2HR4 and JS3HR2, demonstrated potential to inhibit fungal pathogens. These bacteria were identified as Pseudomonas aeruginosa (S1HL3), Burkholderia sp. (S1HL4) and Bacillus sp. (JS2HR4 and JS3HR2) based on biochemical and molecular characteristics. The isolates were tested against Cotton leaf curl virus (CLCuV) in greenhouse conditions, both as individual bacterial isolates and consortia. Treated plants were healthy as compared to control plants, where up to 74% of the plants were symptomatic for CLCuV infection. Maximum inhibition of CLCuV was observed in the plants treated with a mixture of bacterial isolates: the viral load in the treated plants was only 0.4% vs. up to 74% in controls. This treatment consortium included P. aeruginosa S1HL3, Burkholderia sp. S1HL4 and Bacillus spp. isolates, JS2HR4 and JS3HR2. The principal-component biplot showed a highly significant correlation between the viral load percentage and the disease incidence.
Introduction
The effective control and management of plant diseases are prerequisites for sustainable agriculture in the twenty-first century, as many viral, fungal and bacterial diseases pose major threats to the quality and quantity of the production of economically important crops. Cotton is referred to as Pakistan's ‘white gold’. Most of the cotton cultivated in Pakistan is Gossypium hirsutum, with 3% G. arboretum and G. barbadense.[Citation1] Along with poor agricultural practices and low cultivation area, many bacteria, fungi, viruses and insects/pests bring significant losses to the yield of this crop in Pakistan. Cotton leaf curl virus (CLCuV) is the most serious disease of cotton in almost all cotton-growing areas of the country. It was initially reported in 1967 in Multan district as a minor disease to G. hirsutum, but later an epidemic of CLCuV struck during 2003–2004, yielding heavy losses to the production of cotton.[Citation2] In the last few decades, CLCuV has caused cumulative losses of 7.1 million bales ($1.2 billion) in various regions of Multan, Burewala, Khanewal, Vehari, Southern Punjab, Central and Southern Sindh.[Citation3,Citation4] Data on the losses of cotton yield revealed that 38% of the production was lost due to Cotton leaf curl disease (CLCuD) along with other abiotic and biotic factors.[Citation5]
So far, there is no single effective control measure to completely prevent or reduce the incidence of CLCuV. However, the use of chemicals and toxic compounds against whitefly, the transmission vector of CLCuV, is the desirable and unavoidable crop protection measure. Because of indiscriminate and excessive use of such chemicals, whitefly has developed resistance against them. Whitefly strains from the United States, Pakistan, Sudan, Israel, Central America and Europe were found to be resistant against organophosphates and other pesticides.[Citation6] Moreover, the maximum potential of Geminiviruses for recombination has increased the chances of development of more virulent and resistance-breaking variants to knock down genetically induced resistance in plants. Some cultivars that were resistant to Cotton leaf curl Multan virus (CLCuMV) became susceptible to Cotton leaf curl Burewala virus (CLCuBuV), which is a more virulent new generation of virus that has evolved by recombination.[Citation7] The phenomenon of recombination that led to the evolved CLCuMV and CLCuBuV variants is also responsible for the emergence of Cotton leaf curl Shahdadpur virus (CLCuShV) in Sindh.[Citation7]
Microorganisms isolated from the rhizosphere, endosphere or phyllosphere are being commonly used to reduce disease and increase plant growth in various field studies.[Citation8] It has also been reported that the use of beneficial microbes (single/mixture) in farm fields showed much better reduction in diseases as compared to synthetic chemicals.[Citation9–11] These microorganisms may use different mechanisms to provide protection or prevention against plant pathogens, including viruses. They may directly improve the plant growth or indirectly combat the plant pathogen through niche exclusion, antibiosis, competition for nutrients, production of lytic enzymes and induced systemic resistance (ISR).[Citation12–14] Among the biocontrol mechanisms, ISR is the dominant one for reducing viral diseases in host plants.[Citation15,Citation16]
Considering the lack of a most appropriate methodology to control CLCuV and the efficiency of different bacterial isolates in prevention and reduction of plant diseases, the aim of this study was to determine the potential role of various locally isolated bacterial strains in prevention and control of CLCuV.
Materials and methods
Isolation of bacteria
The bacterial strains used in this study were isolated from the rhizosphere and phyllosphere of the cotton plants grown in the fields of CEMB, Punjab University. The soil samples and crushed leaf samples were separately transferred to test tubes containing 10 mL of sterile autoclaved water. Each sample was vortexed gently and then diluted 10−1, 10−2, 10−3 and 10−4 times. Further, 1 mL from each dilution of both rhizosphere and phyllosphere samples was inoculated on respective Luria–Bertani (LB; Gibco, USA) agar plates and incubated at 32 °C for 72 h. Following incubation, well-separated bacterial colonies were subcultured on fresh agar plates for purification.
Selection of bacterial strains as biocontrol agents (BCAs)
The obtained bacterial colonies were screened for their potency as BCAs. Phosphate solubilization, indole acetic acid (IAA) production and antifungal activity were the parameters selected for screening.
Qualitative and quantitative phosphate solubilization test
The isolates were screened for phosphate-solubilizing activity by streaking on National Botanical Research Institute's Phosphate (NBRIP) agar (Hi-media, India) containing insoluble tricalcium phosphate (TCP).[Citation17] After five days of incubation at 32 ± 1 °C, the halo zone formation was analysed for each isolate. Quantitative estimation of solubilized inorganic phosphate was performed by growing 500 µL of bacterial culture in 50 mL of NBRIP broth containing 0.5% TCP. Each bacterial strain was inoculated in triplicate and was kept at 32 ± 1 °C at 180 r min−1 in a shaking incubator (Series 25, InnOva4300, New Brunswick Scientific, USA) for five days. An uninoculated control was also kept under the same conditions. The cultures were then centrifuged at 10,000 r min−1 for 10 min and the phosphate in the supernatant was estimated spectrophotometrically (DU-520, Beckman Coulter, USA) by the vanadomolybdate-yellow method.[Citation17] The total soluble phosphate was calculated from the regression equation of a standard curve. The values of soluble phosphate liberated were expressed as μg mL−1 over the control. The pH of the culture supernatants was also measured using a pH meter (Corning, New York, USA).
IAA production
To determine whether IAA is produced, 50 mL of LB broth was inoculated with 500 µL of 24 h old bacterial cultures. Incubation was done at 32 ± 1 °C at 180 r min−1 for 24–48 h in a shaking incubator. Following incubation, 1 mL of the culture was mixed with 2 mL of Salkowsky reagent and colour development was observed as described by Loper and Schroth.[Citation18]
Biocontrol assay
To assess the bacterial strains for their antifungal potential, fungal cultures of Fusarium solani, Fusarium oxysporum and Rhizoctonia solani were kindly provided by the Institute of Agriculture Sciences, Punjab University, Lahore, India. Fungal mycelia were inoculated at the centre of potato dextrose agar (PDA) plates, while a loop full of each bacterial isolate grown on LB agar for 24 h was stab inoculated 1 cm away from the periphery of agar the plate and 2 cm away from the fungal inoculums.[Citation19] Control plates were only inoculated with the test pathogens and were used for comparison with experimental plates. All of the plates were incubated at 28 °C for seven days and inhibition was determined by observing the reduction in the radial growth of the fungal pathogens.
Biochemical characterization of selected isolates
The bacterial isolates, preliminarily characterized based on phosphate solubilization and IAA production, were further subjected to Gram staining, endospore staining, catalase and oxidase tests. The biochemical and physiological characteristics were determined by using a QTS-24 miniaturized identification system (DESTO Laboratories, Rawalpindi, Pakistan) as per instructions.
Molecular identification of bacterial isolates
To characterize the isolated bacterial strains at the molecular level, the 16S rRNA gene was amplified and sequenced. For this purpose, the total genomic DNA of selected bacterial isolates was obtained by the Cetyltrimethylammonium bromide (CTAB) method as described by Wilson.[Citation20] Amplification was performed in a polymerase chain reaction (PCR) thermal cycler (PCR System 9700; Applied Biosystems, USA) in 20 µL reaction mixture containing 0.1 mmol L−1 of each primer, 2.5 mmol L−1 MgCl2, 1 mmol L−1 of each deoxynucleoside triphosphate (dNTP), 50 ng µL−1 of template DNA and 2 U µL−1 Taq DNA polymerase (Thermoscientific, USA). Universal primers 5′-AACGCGAAGAACCTTAC-3′ and 5′-CGGTGTGTACAAGACCC′-3′ were used to amplify a 1.5 kb gene fragment. The cycling conditions were, initial denaturation at 95 °C for 5 min followed by 35 amplification cycles of denaturation at 95 °C for 1 min, annealing at 57 °C for 1 min and extension at 72 °C for 1.5 min, followed by final extension at 72 °C for 10 min. The PCR products were analysed by electrophoresis in a 1% agarose gel in 1X TAE (Tris-acetate-ethylenediaminetetraacetic acid) buffer with ethidium bromide staining. The PCR products were gel purified and were sequenced by Macrogen (Korea). The deduced sequences were submitted to the GenBank database and accession numbers were obtained. Further, identification of phylogenetic neighbours and calculation of pairwise similarity of these sequences were done by using the National Center for Biotechnology Information (NCBI) taxonomy tree building service, ClustalW and MEGA 5.2 software.
Greenhouse trial of selected BCAs on CLCuV-susceptible cotton plants
To test the potential efficacy of the screened bacterial strains as biological control agents, a CLCuV-susceptible cotton variety, CIM-496, was used in the greenhouse experiments. Seven different combinations of bacterial consortia were formulated for seven individual treatments. In total, 64 cotton plants were included in the trial with 8 plants per treatment and a control (non-infected) variant. The trial was based on randomized complete block design. The pots were fertilized with a nitrogenous fertilizer (urea at 9 g/pot) once a month and watered on alternate days. The temperature conditions were kept as 30/25 ± 2 °C (day/night) with 70% relative humidity during the study period, while the photoperiod was maintained at a 16/8 h light/dark regime during the experiment by exposing the plants to sunlight and artificial light.
Maintenance of CLCuV inoculum
During experimentation, the virus inoculum was maintained on naturally infected old plants of the susceptible variety. To ensure efficient transmission to the healthy plants, infected plants with 15–20 whiteflies per leaf were kept among the experimental pots. The whiteflies from infected plants were allowed to feed on healthy plants throughout the whole experiment in order to transfer the virus. Maximum/minimum temperature and humidity level were maintained as per requirement of the plants.
Preparation of bacterial formulations and application on cotton plants
Selected bacterial isolates were grown in LB medium in 250 mL Erlenmeyer flasks for 24 h. All isolates were harvested after 24 h and mixed in seven different combinations that were subsequently used for potted plant experiments in greenhouse conditions. Foliar sprays of BCAs in consortia were applied once on the healthy experimental plants before the transfer of CLCuV from infected plants. After the whiteflies started feeding on the healthy plants, BCAs spray was initiated after 5 days and was repeated thrice at the interval of every 15 days, while control plants were sprayed with water against the treated plants. CLCuV symptoms were scored in the treated and control plants after the application of BCA mixture at a regular interval of seven days according to a 0–6 integer scale as described by Akhtar et al. [Citation4,Citation21].
0: No symptoms;
1: Thickening of a few small scattered veins;
2: Thickening of a small group of veins;
3: Thickening of all veins;
4: All vein thickening and leaf curling at the top of the plant;
5: Severe vein thickening and leaf curling on half of the plant;
6: Severe vein thickening, leaf curling, foliar outgrowth (enation).
The percentage of disease incidence (%DI) of the plants was calculated using the following equation:where Sd is the sum of all disease ratings, Y is the total number of plants assessed and Z is a constant that refers to the integer scale ratings (0–6) that we included.
Virus detection in cotton plants
At the end of the trial, young leaves were picked from the cotton plants in the greenhouse and total genomic DNA was isolated from them through the CTAB method.[Citation22] DNA was resuspended in 1X TE (Tris-ethylenediaminetetraacetic acid) buffer. Further, to determine viral load in treated and control plants, PCR was performed using the AV1 gene (DNA-A; CLCuV)-specific primers L1 5′-AGCCCAGTACAGCAACTGTG-3′ and R1 5′-CTTGACCAAAGCCTGTTCC-3′ to amplify a 180 bp conserved coat protein gene fragment. The PCR mixture was prepared by adding 3 µL of template DNA (50 ng µL−1), 1 mmol L−1 dNTPs, 0.1 mmol L−1 of each primer, 2.5 mmol L−1 MgCl2, 2 U µL−1 Taq DNA polymerase (Thermoscientific) in a total volume of 20 µL. The cycling profile was initial denaturation at 95 °C for 4 min followed by 35 cycles of denaturation at 95 °C for 1 min, annealing at 56 °C for 45 s and extension at 72 °C for 45 s and a final extension at 72 °C for 7 min. The PCR products were analysed in a 2% agarose gel with ethidium bromide staining.
Data analysis
The quantity of virus in each sample (virus titre) was detected by densitometric analysis of agarose gel images using ImageJ software (version 1.33, USA). Principal-component biplots were drawn using GenStat software (10th edition, VSN International, UK).
Results and discussion
Modern practices regarding the control of viral diseases in plants mainly include the use of synthetic chemicals to destroy the vector, manipulation of environmental factors, incorporation of resistance against the virus by genetic engineering and biocontrol measures. The use of pesticides or insecticides is definitely an important and effective measure to control the vector, and a large number of such chemicals are available, which give promising results. However, it is no longer a sustainable approach for the control of CLCuV because of the emergence of resistant whitefly species.[Citation23] There is an increasing demand of new methods that may replace the older ones in a better way and support the drooping cotton economy. Several approaches have been made to control CLCuV, but no such strategy is available that can provide absolute protection to the cotton crop.
One of the novel approaches for the management of CLCuD is the use of microbial inoculants that can significantly reduce disease incidence and may also promote the growth of the plants along with ensuring user and environmental safety. The trend of using microbial inoculations to reduce viral disease is gaining ground and is reported for tomato mottle virus in tomato,[Citation9] cucumber mosaic virus in cucumber,[Citation24] tomato mosaic virus in tomato,[Citation25] tomato necrosis virus,[Citation26] banana bunchy top virus in banana,[Citation27] tomato spotted wilt virus in tomato [Citation11] and sunflower necrosis virus in sunflower.[Citation28] To the best of our knowledge, there has been no attempt, so far, to use BCAs in the management of CLCuD.
Biochemical identification of bacterial strains
The ultimate goal of the present study was to isolate beneficial bacteria and then develop a mixture of these strains that would provide resistance against CLCuD. Generally, there is no target site for the isolation of such bacteria that can be helpful in providing resistance against plant diseases, but the best place to obtain such bacteria is the same as that for the target pathogen.[Citation29] Keeping this in view, the microorganisms included in this study were also isolated from the phyllosphere. Morphologically, the colonies of all obtained isolates were round in shape, except JS2HR4, which had colonies with irregular rhizoidal margins. Two bacterial isolates were Gram-positive and another two were Gram-negative when stained and observed under a light microscope. When tested for oxidase reaction, appearance of violet colour within 30 s was only observed for S1HL3 and S1HL4. The results from further biochemical tests are summarized in . S1HL3 was tentatively identified as Pseudomonas aeruginosa, S1HL4 as Burkholderia, JS2HR4 and JS3HR2 belonged to Bacillus species.
Table 1. Biochemical identification test results for selected isolates using QTS-24 miniaturized test system.
Screening of bacterial isolates
Phosphate solubilization
Distinctly separated colonies obtained on LB agar plates were then screened for certain activities which are the characteristics of BCAs. For instance, qualitative tests for phosphate solubilization resulted in 12 out of 30 isolates developing zones. The total diameter of the zones produced by selected bacterial strains ranged from 4 to 15 mm (data not shown). P. aeruginosa (S1HL3) and Burkholderia (S1HL4) produced sharp zones of solubilization of 13 and 15 mm in diameter, respectively. Other isolates were either non-phosphate solubilizers or produced a very small zone of solubilization and, hence, were not included in the list of potential BCAs. The quantitative estimation of TCP solubilization for both isolates (S1HL3 and S1HL4) ranged from 392 to 509 mg L−1. In the cultures of these two isolates, the pH decreased from 7 to 5.6 after an incubation period of five days. Microorganisms such as Pseudomonas, Bacillus, Rhizobium and Enterobacter are regarded as powerful phosphate solubilizers and have been tested in vivo for their efficiency.[Citation30] It has also been demonstrated that phosphate solubilization decreases as the buffering capacity of the medium increases and reaches a maximum at acidic pH.[Citation31]
IAA production
Similarly, 10 bacterial isolates produced the plant growth-promoting hormone IAA, while others were negative for the said trait. The intensity of the pink colour was compared with the control. Conclusively, only two isolates, S1HL3 and S1HL4, were found positive for both phosphate solubilization and IAA production.
Fungal antagonism
Regarding the antagonism property of the bacterial isolates, two isolates from the rhizosphere were capable of inhibiting F. oxysporum and F. solani to a different extent when streaked opposite to fungus inoculums on PDA. Three more isolates were found capable of inhibiting R. solani, but the inhibition was not very prominent. Hence, only two isolates, JS2HR4 and JS3HR2 (Bacillus spp.), were selected as BCAs and used in the greenhouse trial after further characterization.
Molecular characterization and phylogenetic analysis
Molecular identification of the bacterial strains was done by performing 16S rRNA gene sequence analysis. A sharp amplification was observed at 1.5 kb as depicted in . The retrieved sequences were submitted in GenBank and the following accession numbers were obtained: KC928325.1 (Bacillus subtilis), KC928326 (Bacillus methylotrophicus), KC928323 (P. aeruginosa) and KC928324 (Burkholderia). The similarity search for the sequenced amplification products showed that the 16S rRNA gene sequence of isolate S1HL3 was 99% similar to that of P. aeruginosa; the S1HL4 sequence was similar to Burkholderia sp. (99%); that of JS2HR4, with B. methylotrophicus (100%) and the JS3HR2 sequence, with B. subtilis (99%). The phylogenetic tree constructed based on these sequences is shown in
Efficacy of bacterial isolates for control of CLCuV in greenhouse conditions
Based on plant growth promotion traits (preliminary experiments; data not shown), seven different treatments (T1–T7) were designed in order to obtain an efficient consortium for the management of CLCuV (). Vein thickening in a large group of veins was observed among control plants (treated only with water) after 15 days of whitefly attack, while BCA treated plants showed no such symptoms (). Thickening of a small group of veins appeared in the T3 and T4 plants after the third week of viral transmission. The %DI values were calculated for each treatment after the second, third, fourth and fifth weeks of virus transmission in plants (). The disease reactions observed in each treatment variant were compared according to the mean values of %DI observed. It was found that foliar application of some of the bacterial isolates to plants individually (i.e. as single bacterial isolates) as BCA led to short-term protection as compared to that observed in the plants treated with mixtures.
Table 2. Inclusion criteria for the design of consortia with bacterial isolates selected for control of CLCuD in greenhouse experiments.
Figure 3. Appearance of CLCuD symptoms in control and treated plants after two weeks of whitefly attack. Leaves from control and treated plants (a); control plant (b); BCA treated plant (c).
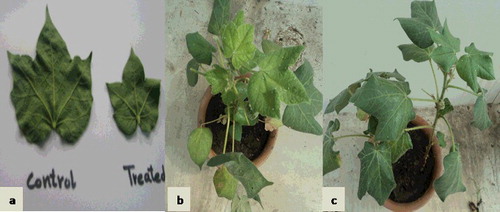
Table 3. Incidence of disease in control and treated plants over a period of five weeks after virus transmission.
It has been already proved that Bacillus, Pseudomonas and Burkholderia are among those species that not only promote plant growth, but also provide resistance against diseases.[Citation32] Although BCAs can combat plant pathogens when they are used individually, in our study, it was observed that the consortia of bacterial strains conferred a better degree of resistance than some of the single isolates. This could suggest that in consortium treatment, if one organism fails to block the pathogen in different field and environmental circumstances, then the other organism(s) may provide defence against the pathogen.[Citation28] Various studies have demonstrated that combined use of Bacillus and Pseudomonas with other BCAs results in more effective control of diseases in plants. For instance, Trichoderma sp. and Pseudomonas sp. reduced root rot in pea and rice plants and the results were better than those obtained with single T. herzianum or Pseudomonas sp. treatment.[Citation33,Citation34] Moreover, it was observed that the BCAs applied in this investigation did not show any negative effects on the plants; instead, they worked synergistically or separately to protect the plants.
Quantification of virus titre
The results from the PCR amplification of the conserved coat protein gene of CLCuV showed that the plants treated with consortia were healthier when compared with those treated with single isolates and the control plants. The quantity of virus in each sample was detected. It was found that the CLCuV titre was highest in control plants (74%) and lowest in the plants of the T6 and T7 treatment variants (0.6% and 0.4%, respectively). In T3 and T4 plants, the percentages of the viral load were 72% and 25%, respectively (). A possible explanation of the low virus titre among the plants is the likely inhibition or down-regulation of coat protein expression – and, hence, a reduced number of virus particles – during the early stages of infection, resulting in impaired replication and delayed disease onset. For example, peanut bud necrosis virus disease was also controlled by blockage of the systemic movement of the virus in wild Arachis after inoculation with BCAs.[Citation35] There might also be interference of plant defence signals with the replication of virus, cell-to-cell movement or systemic spread of the virus in the plant or the induction of systemic resistance.[Citation11,Citation28] It could be speculated that the low viral titres were due to ISR. Thus, it could be hypothesized that the bacterial isolates used in this experiment may have triggered ISR in the host plants that may have eventually initiated defence mechanisms [Citation36] providing sustainable resistance against CLCuV.
Principal-component biplot
The principal-component biplot () clearly depicts that the correlation between the percentage of viral load and the percentage of disease incidence was highly significant (the bigger the vector between the vector point and labels, the more significant the results are). The treatment and disease incidence vectors determine that the consortium treatments to the right of tend to have the least viral load and no CLCuV symptoms, whereas, treatments to the left of the figure tend to have maximum viral load and were considered as CLCuV-susceptible. Moreover, treatment vectors T6 and T7 which gave similar scores (and small angles between the vectors), generated almost similar responses, indicating that the plants subjected to these treatments could be considered as very resistant to the virus because of the applied treatment.
Figure 5. A principal-component biplot of the viral load percentage, the percentage of disease incidence with bacterial treatment.
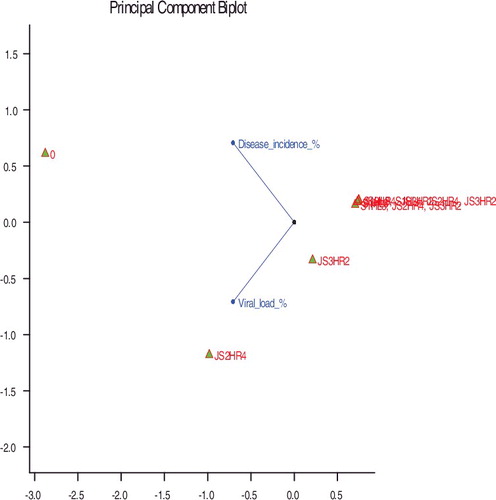
Taken together, the information obtained from the present study could help in the development of bioformulations for effective management of CLCuV. There is a need to determine the quantity, frequency and the best method of delivery of these potential BCAs in the field. Molecular evidence or involvement of different plant and viral genes in combating CLCuD is yet to be explored.
Conclusions
The obtained results suggest that the studied Bacillus, Pseudomonas and Burkholderia isolates are among those strains that not only promote plant growth, but also provide resistance against diseases. In addition to providing resistance against bacterial or fungal diseases, these isolates can also protect against viral diseases in plants. Although, these BCAs can combat plant pathogens when they are used individually; in our study, it was observed that the degree of resistance was much higher when consortia of bacterial strains were applied onto the plants. Further research is needed to optimize the protocol for administration of these potential BCAs and to elucidate the molecular mechanisms involved in the resistance to CLCuD.
Disclosure statement
No potential conflict of interest was reported by the authors.
References
- Briddon R, Markham P. Cotton leaf curl virus disease. Virus Res. 2000;71:151–159.
- Amrao L, Akhter S, Tahir MN, et al. Cotton leaf curl disease in Sindh province of Pakistan is associated with recombinant begomovirus components. Virus Res. 2010;153:161–155.
- Hussain M, Azhar FM, Khan AA, et al. Expression of genes controlling the inheritance of resistance to cotton leaf curl virus disease (CLCuD) in Gossypium hirsutum L.: a quantitative analysis. Pak J Bot. 2012;44:247–254.
- Akhtar KP, Khan AI, Hussain M, et al. Comparison of resistance level to cotton leaf curl virus (CLCuV) among newly developed cotton mutants and commercial cultivars. Plant Pathol J. 2002;18:179–186.
- Amjad M, Bashir MH, Afzal M, et al. Efficacy of some insecticides against whitefly (Bemisia tabaci Genn.) infesting cotton under field conditions. PJLS S. 2009;7:140–143.
- Cahill M, Gorman K, Day S, et al. Baseline determination and detection of resistance to imidacloprid in Bemisia tabaci (Homoptera: Aleyrodidae). Bull Entomol Res. 1996;86:343–349.
- Farooq A, Farooq J, Mahmood A, et al. An overview of cotton leaf curl virus disease (CLCuD) a serious threat to cotton productivity. AJCS. 2011;5:1823–1831.
- Senthilraja G, Anand T, Kennedy J, et al. Plant growth promoting rhizobacteria (PGPR) and entomopathogenic fungus bioformulation enhance the expression of defense enzymes and pathogenesis-related proteins in groundnut plants against leafminer insect and collar rot pathogen. Physiol Mol Plant Path. 2013;82:10–19.
- Murphy JF, Zehnder GW, Schuster DJ, et al. Plant growth-promoting rhizobacterial mediated protection in tomato against Tomato mottle virus. Plant Dis. 2000;84:779–784.
- Gray E, Smith D. Intracellular and extracellular PGPR: commonalities and distinctions in the plant–bacterium signaling processes. Soil Biol Biochem. 2005;37:395–412.
- Kandan A, Ramiah M, Vasanthi V, et al. Use of Pseudomonas fluorescens-based formulations for management of tomato spotted wilt virus (TSWV) and enhanced yield in tomato. Biocontrol Sci Technol. 2005;15:553–569.
- Haas D, Keel C. Regulation of antibiotic production in root-colonizing Pseudomonas spp. and relevance for biological control of plant disease. Annu Rev Phytopathol. 2003;41:117–153.
- Ongena M, Jourdan E, Schäfer M, et al. Isolation of an N-alkylated benzylamine derivative from Pseudomonas putida BTP1 as elicitor of induced systemic resistance in bean. MPMI. 2005;18:562–569.
- Jeger MJ, Jeffries P, Elad Y, et al. A generic theoretical model for biological control of foliar plant diseases. J Theor Biol. 2009;256:201–214.
- Lenardon SL, Bazzalo M, Abratti G, et al. Screening sunflower for resistance to sunflower chlorotic mottle virus and mapping the resistance gene. Crop Sci. 2005;45:735–739.
- van Loon LC, Glick BR. Increased plant fitness by rhizobacteria. In: Sandermann H, editor. Molecular ecotoxicology of plants. Volume 170, ecological studies. Heidelberg: Springer; 2004. p. 177–205.
- Nautiyal CS. An efficient microbiological growth medium for screening phosphate solubilizing microorganisms. FEMS Microbiol Lett. 1999;170:265–270.
- Schroth MN, Loper JE. Influence of bacteria sources of indol-3-acetic acid on root elongation of sugar beet. Phytopathol. 1986;76:386–389.
- Sharma K, Mishra AK, Misra RS. Morphological, biochemical and molecular characterization of Trichoderma harzianum isolates for their efficacy as biocontrol agents. J Phytopathol. 2009;157:51–56.
- Wilson K. Preparation of genomic DNA from bacteria. Curr Protoc Mol Biol. 2001;00:I:2.4:2.4.1–2.4.5.
- Akhtar KP, Hussain M, Khan AI, et al. Influence of plant age, whitefly population and cultivar resistance on infection of cotton plants by cotton leaf curl virus (CLCuV) in Pakistan. Field Crops Res. 2004;86:15–21.
- Clarke JD. Cetyltrimethyl ammonium bromide (CTAB) DNA miniprep for plant DNA isolation. Cold Spring Harb Protoc. 2009;2009(3):pdb. prot5177.
- Basit M, Sayyed AH, Saeed S, et al. Lack of fitness costs associated with acetamiprid resistance in Bemisia tabaci (Hemiptera: Aleyrodidae). J Econ Entomol. 2012;105:1401–1406.
- Zehnder GW, Yao C, Murphy JF, et al. Induction of resistance in tomato against cucumber mosaic cucumovirus by plant growth-promoting rhizobacteria. BioControl. 2000;45:127–137.
- De Meyer G, Audenaert K, Höfte M. Pseudomonas aeruginosa 7NSK2-induced systemic resistance in tobacco depends on in planta salicylic acid accumulation but is not associated with PR1a expression. Eur J Plant Pathol. 1999;105:513–517.
- Maurhofer M, Hase C, Meuwly P, et al. Induction of systemic resistance of tobacco to tobacco necrosis virus by the root-colonizing Pseudomonas fluorescens strain CHA 0: influence of the gacA gene and of pyoverdine production. Phytopathol. 1994;84:139–146.
- Kavino M, Harish S, Kumar N, et al. Induction of systemic resistance in banana (Musa spp.) against Banana bunchy top virus (BBTV) by combining chitin with root-colonizing Pseudomonas fluorescens strain CHA0. Eur J Plant Pathol. 2008;120:353–362.
- Srinivasan K, Mathivanan N. Biological control of sunflower necrosis virus disease with powder and liquid formulations of plant growth promoting microbial consortia under field conditions. Biol Control. 2009;51:395–402.
- Bashan Y, Holguin G, Lifshitz R. Isolation and characterization of plant growth-promoting rhizobacteria. In: Glick BR, Thompson JE, editors. Methods in plant molecular biology and biotechnology. Boca Raton: CRC Press; 1993. p. 331–345.
- Khan AA, Jilani G, Akhtar MS, et al. Phosphorus solubilizing bacteria: occurrence, mechanisms and their role in crop production. J Agric Biol Sci. 2009;1:48–58.
- Walpola BC, Song J-S, Keum M-J, et al. Evaluation of phosphate solubilizing potential of three Burkholderia species isolated from green house soils. Korean J Soil Sci Fert. 2012;45:602–609.
- Berg G. Plant–microbe interactions promoting plant growth and health: perspectives for controlled use of microorganisms in agriculture. Appl Microbiol Biotechnol. 2009;84:11–18.
- Dandurand L, Knudsen G. Influence of Pseudomonas fluorescens on hyphal growth and biocontrol activity of Trichoderma harizanum in the spermosphere and rhizosphere of pea. Phytopathol (USA). 1993;83:265–270.
- Mathivanan N, Prabavathy V, Vijayanandraj V. Application of talc formulations of Pseudomonas fluorescens Migula and Trichoderma viride Pers. ex SF Gray decrease the sheath blight disease and enhance the plant growth and yield in rice. J Phytopathol. 2005;153:697–701.
- Reddy A, Reddy L, Mallikarjuna N, et al. Identification of resistance to peanut bud necrosis virus (PBNV) in wild Arachis germplasm. Ann Appl Biol. 2000;137:135–139.
- Van Loon L, Bakker P, Pieterse C. Systemic resistance induced by rhizosphere bacteria. Annu Rev Phytopathol. 1998;36:453–483.