ABSTRACT
To obtain an alternative source for the production of gentiopicroside, here genomic DNA segments of the medicinal plant Gentiana macrophylla were randomly transferred into Hansenula polymorpha by 25 KeV nitrogen ions (N+) at a dose of 2.5 × 1016 ions/cm2 under vacuum pressure of 1 × 10−3 Pa. To screen for potential gentiopicroside-producing recombinant yeast strains, geraniol 10-hydroxylase (G10H) and secologanin synthase (SLS) involved in the gentiopicroside biosynthesis pathway were used as molecular markers. Based on the conserved protein sequences of G10H and SLS, degenerate primers were designed and used for colony polymerase chain reaction (PCR). PCR-positive results for both the G10H and SLS genes were obtained in 79 out of 653 transformants by low-energy ion beam-mediated transformation. These 79 potential gentiopicroside-producing strains were further analysed by Fehling's test, thin-layer chromatography, high performance liquid chromatography and high performance liquid chromatography-mass spectrometry. The results showed that the retention time and ion peaks of the sample from one stable recombinant strain designated as DL67 were consistent with those of the gentiopicroside standard. The corresponding gentiopicroside yield was 8.41 mg/g dry cell weight after strain DL67 was cultured for 96 h. This could offer a new starting point for the construction of recombinant yeasts for production of medicinal plant compounds.
Introduction
Gentiopicroside, the main secoiridoid glucoside of Gentiana macrophylla, is a compound of pharmaceutical importance. It has a range of valuable pharmacological functions, including antifungal activity,[Citation1] antibacterial activity against Staphylococcus aureus,[Citation2] antipsychotic activity [Citation3] and in vivo hepatoprotective activity against chemically and immunologically induced hepatic injuries.[Citation4] Gentiopicroside is mainly isolated from G. macrophylla, a perennial herb belonging to the genus Gentianaceae, which is widely used as a traditional herbal drug to treat diabetes, apoplexy, paralysis and rheumatism because its roots are rich in gentiopicroside.[Citation5] Recently, wild resources of G. macrophylla have been overexploited in China owing to the increasing commercial interest in gentiopicroside as a medical treatment. What is more, the seeds of G. macrophylla are difficult to germinate and only a very tiny proportion of seedlings actually produce mature adult plants in the wild. Thus, the unsustainable use of wild resources of G. macrophylla means that the plant is at risk of becoming extinct.[Citation6] Thus, it is important to establish an alternative method to obtain gentiopicroside.
Various strategies have been attempted in concerted efforts for the production of desirable medicinal compounds from plants.[Citation7,Citation8] Notably, yeasts have been engineered and widely exploited as an alternative sustainable source of plant-derived secondary metabolites because of their fast growth rate and low production costs,[Citation9,Citation10] while reducing the environmental costs associated with the consumption of natural resources. Unfortunately, the genes responsible for gentiopicroside biosynthesis have not been fully elucidated. In the medical plant Catharanthus roseus, the precursors of gentiopicroside and secoiridoids are biosynthesized via the iridoid pathway and have been well studied and reviewed.[Citation11] In higher plants, the first formation step of isopentenyl diphosphate (IPP), a precursor of terpenoids, is through to be both the plastidial methyl-erythritol phosphate (MEP) pathway and the cytosolic mevalonate (MVA) pathway.[Citation12,Citation13] Isomerization of IPP by IPP isomerase produces geranyl diphosphate, which is converted into geraniol via the enzyme geraniol synthase.[Citation14] Geraniol is then converted into 10-hydroxygeraniol by the enzyme of geraniol 10-hydroxylase (G10H). Further, through a series of catalytic reactions involving the enzymes acyclic monoterpene primary alcohol dehydrogenase, monoterpene cyclase, secologanin synthase (SLS) and other undefined enzymes, 10-hydroxygeraniol is converted into secologanin.[Citation15] Ultimately, secologanin is converted into gentiopicroside and other secoiridoids through several currently unknown steps. Therefore, the construction of gentiopicroside-producing recombinant yeast by traditional genetic engineering mediated synthetic biology remains challenging.
This problem might be addressed by a new highly interdisciplinary field, ion beam bioengineering, in which low-energy ion implantation is applied to living biological materials to advance the engineering of organisms.[Citation16] In 1988, the etching effect of ion beams on the cell wall was discovered, which subsequently led to the rapid development of low-energy ion-beam-induced exogenous macromolecule transfer into biological cells.[Citation17]
In this study, the ion beam-mediated gene transfer method was used to introduce genomic DNA segments of the medicinal plant G. macrophylla into Hansenula polymorpha to achieve single-step whole-pathway assembly of gentiopicroside biosynthesis in yeasts. Similarly, to screen for potential gentiopicroside-producing recombinant yeast strains, the downstream biosynthesis genes involved in the gentiopicroside biosynthesis pathway were used as molecular markers in colony polymerase chain reaction (PCR).
Materials and methods
Strains, media and instruments
H. polymorpha DL-1 was used as host cells. The strain was grown in yeast extract peptone dextrose (YPD) medium, containing yeast extract (10 g/L), peptone (20 g/L) and glucose (20 g/L). The corresponding transformants were grown at 37 °C in YPD medium. For screening, transformants were cultured in basal medium containing glycerol (20 g/L) and yeast nitrogen base (YNB, 13.4 g/L) in baffled flasks. An ion implanter (Chengdu Tongchuang Applied Plasma Technology Center, Chengdu, China) was used to produce ion implantation sources in this study. Gentiopicroside standard (≥98.5%, HPLC) was supplied by Shanghai R&D center for standardization of traditional Chinese medicine.
Preparation of monolayer H. polymorpha cells
A starter H. polymorpha culture was prepared by inoculating a fresh single colony at 37 °C for 16 h in 3 mL of YPD medium. The cells were harvested by centrifugation (3000 g, 5 min) and subsequently resuspended in 1 mL protection buffer composed of 0.5% glucose and 0.5% starch to a density of approximately 1 × 107 CFU/mL. Then, an aliquot (0.1 mL) of the suspension was plated onto the centre of a sterile 90 mm Petri dish to form a pellicle with a diameter of approximately 60 mm in a monolayer manner, and was further air dried under aseptic conditions.
Ion beam assisted G. macrophylla genomic DNA segment transfer into H. polymorpha
For gene transfer, genomic DNA segments of G. macrophylla were prepared following the standard cetyltrimethylammonium bromide protocol, and subsequently diluted with Tris-EDTA (TE) buffer (10 mmol/L Tris-Cl, 1 mmol/L ethylenediaminetetraacetic acid, pH 7.5) to 100 μg/mL. For H. polymorpha ion implantation, the Petri dish with the monolayer of H. polymorpha cells was placed in a vacuum bio-sample target chamber of the ion implantation facility, and then the cells were implanted by 25 KeV nitrogen ions (N+) at a dose of 2.5 × 1016 ions/cm2 under vacuum pressure of 1 × 10−3 Pa. After ion implantation, 2 mL of TE buffer with or without G. macrophylla genomic DNA was immediately added to the plate; respectively, the latter was used as the control. The cells were incubated for 2 h at 37 °C on a rotary shaker (100 r/min). Later the cells were thoroughly resuspended in 2 mL of TE buffer and 0.1 mL of the suspension was plated onto YPD medium. After incubation for 72 h at 37 °C, the clones were randomly selected and analysed by PCR for the presence of the G10H and SLS genes. In addition, the clones were cultured by shifting cells from YPD to basal liquid medium for further 96 h at 37 °C. To further screen gentiopicroside-producing yeast strains, 3 mL of culture supernatants was used directly for Fehling's test.
High-throughput screening of potential gentiopicroside-producing recombinant yeasts using colony PCR
Colony PCR was employed to screen the potential gentiopicroside-producing yeast recombinant strains based on the deduced putative biosynthesis pathway for gentiopicroside biosynthesis. The yeast colonies were picked from an agar plate culture and treated with 100 µL of 300 mmol/L lithium acetate (LiOAc), 2% sodium dodecyl sulfate (SDS)s solution. The cell suspensions were incubated at 75 °C for 30 min. After the addition of 300 µL of 100% ethanol, the samples were mixed and placed at −20 °C for further 30 min. The mixture was further centrifuged at 13,000 × g for 5 min. Following the centrifugation, the supernatant was withdrawn and dissolved by brief vortexing in 100 µL H2O. Finally, cell debris was spun down by centrifugation for at 12,000 × g for 1 min, and 2.5 µL of the supernatant was used for PCR. The remainder was stored at −20 °C for further experiments.
The downstream biosynthesis genes of G10H and SLS involved in the gentiopicroside biosynthesis pathway were selected as molecular markers. Based on the conserved protein sequence of G10H, the degenerate primers G10H-F: 5’-CCAAGGAGGTGCTGCAGAARCANGAYYT-3’ and G10H-R: 5’-CCGGCCCACGTCCACNGCYTCNCC-3’ were designed and synthesized using COnsensus-DEgenerate Hybrid Oligonucleotide Primers (CODEHOP).[Citation18] Similarly, the degenerate primers SLS-F: 5’-GAGAGGGCCAGGGAGGARGTNYTNCA-3’ and SLS-R: 5’-TCTGGCCCAGGCACACNCKNGGNCCCCA-3’ were designed and synthesized according to the conserved protein sequence of SLS.
The yeast transformants were first screened by PCR for the presence of the G10H gene. PCR amplification was performed using the primer pair G10H-F and G10H-R in a typical 25 μL reaction mixture (TIANGEN Biotech (Beijing) Co., Ltd, China) containing 2 U of Taq DNA polymerase. The cycling parameters were 35 cycles, which consisted of 95 °C for 30 s, 56 °C for 30 s and 72 °C for 60 s. The products were stained with ethidium bromide using electrophoresis in a 1% Tris-acetate-EDTA (TAE) agarose gel and visualized under ultraviolet (UV) light. The PCR-positive yeast transformants were further selected for the next screening.
The yeast transformants with the G10H gene were further screened by PCR screening for the SLS gene. PCR amplification of the SLS gene was carried out using the primers SLS-F and SLS-R in a 25 μL reaction mixture (TIANGEN Biotech (Beijing) Co., Ltd, China) containing 2 U of Taq DNA polymerase the cycling parameters were the same as above. The amplified DNA fragments were analysed by agarose gel electrophoresis.
Qualitative screening of gentiopicroside-producing recombinant H. polymorpha yeasts
Fehling's test for qualitative screening of target recombinant H. polymorpha yeast was performed as follows: in a test tube, 3 mL of culture supernatant was mixed with 1.5 mL of freshly prepared Fehling's solution. Then, the test tubes were heated in a vigorously boiling water bath for 5 min. After they were cooled, a positive result was indicated by the formation of a brick red precipitate. Similarly, thin-layer chromatography (TLC) was utilized to further qualitatively analyse the culture supernatants of the recombinant pre-screened yeast using chloroform: methanol (4:1, v/v), a developing agent. For TLC, 1 mg of gentiopicroside was dissolved in 1 mL of methanol and was used as the standard solution. For each sample solution and the standard solution, 10 μL was spotted on a plate of silica gel with a fluorescent indicator, respectively. Furthermore, to analyse the genetic stability of gentiopicroside-producing recombinant yeast, the screened recombinant yeast was subcultured for 20 generations in YPD liquid medium and the gentiopicroside yield was measured in 96-h cultures each time.
High performance liquid chromatography (HPLC) analysis of the target recombinant transformant
The target recombinant yeast clone was inoculated in test tubes and incubated for 16 h at 37 °C, and then transferred into 500 mL flasks containing basal liquid medium. After further 96 h at 37 °C, the culture supernatants were collected by centrifugation (8000 × g, 10 min) and assayed for gentiopicroside using reverse-phase HPLC. The reverse-phase HPLC system consisted of a Waters 600E pump, a Waters 2487 UV tunable absorbance detector and an Empower data-processing system. The chromatographic method was carried out on a Diamonsil C18 column (4.6 × 250 mm ID, 5 μm) at 30 °C and the column eluents were monitored at 272 nm. The mobile phase consisted of methanol/H2O (30:70, by vol.). The injection volume of sample was 20 μL and the flow rate of the mobile phase was 1.0 mL/min. Standard samples were used for instrument calibration, which was done one or two times every three to five samples.
To analyse the yield of gentiopicroside, the target recombinant yeast clone was cultured for 120 h in glycerol-containing YNB medium at 37 °C and 180 r/min in 500 mL flasks and the biomass (dry cell weight, DCW) and the gentiopicroside yield was analysed using a linear regression equation.
High performance liquid chromatography-mass spectrometry (HPLC-MS) analysis
Culture supernatants of the potential gentiopicroside-producing recombinant yeast clone were analysed using HPLC-MS (Bruker Agilent1100-Esquive 6000, Waldbronn, Germany) coupled with an electro spray ionization (ESI) source. The chromatographic conditions were as follows: Diamonsil C18 column (250 mm × 4.6 mm, 5 μm), mobile phase of methanol/H2O (30:70, by vol.), flow rate of 0.6 mL/min, column temperature of 30 °C and injection volume of 10 μL. The mass-spectrometric conditions were as follows: negative ion detection mode, capillary spraying voltage of 4000 V, nebulizer pressure of 0.3 bar, dry gas airflow velocity of 4.0 L/min and scan range from 50 to 800 m/z.
Detection of G10H and SLS gene expression using quantitative reverse transcription PCR (qRT-PCR)
To test how the G10H and SLS genes play a role in the synthesis of gentiopicroside at different incubation time, we determined the mRNA levels for the G10H and SLS genes via quantitative real-time PCR (qPCR). Cultures of H. polymorpha wild-type strain DL-1 and the gentiopicroside-producing recombinant H. polymorpha strain DL67 were grown on YPD medium for 120 h at 37 °C. Cells of H. polymorpha strain DL67 and the wild-type strain DL-1 were inoculated on YPD medium. Cells were recovered from the medium surface at 24, 48, 72, 96 and 120 h post-inoculation. Total RNAs were purified, and first-strand cDNAs were synthesized. Each cDNA sample was used as a template to amplify the G10H and SLS genes, using gene-specific primers in a qRT-PCR, respectively. For total RNA extraction, the collected cells were first washed with distilled water. Thereafter, total RNAs were extracted using Trizol reagents (Invitrogen) and purified with a Qiagen RNeasy Cleanup Kit (Qiagen), according to the manufacturer's instructions. Purified RNAs were quantified using a Nanodrop instrument (Thermo Scientific). First-strand cDNAs were synthesized using a Superscript III cDNA synthesis Kit (Invitrogen), following the instructions provided by the manufacturer. The expression level of the G10H gene (G10H-qF: 5’-GAATATCCCAAGGAGGCGTA-3’; G10H-qR: 5’-GCTGCAAATAGATCCAAGCA-3’), the SLS gene (SLS-qF: 5’-AGAGAGAAGAGCATTTGG-3’; SLS-qR: 5’-CATGAACATAGGATGGAG-3’) and the ACTIN gene as an endogenous reference (ACT-qF: 5’-TTACGTGGCTCTTGACTTCG-3’; ACT-qR: 5’-GACCTGGGTGGAACAGAACT-3’) was measured with the comparative threshold cycle (CT) method, using Brilliant SYBR green qPCR reagents (Stratagene) as previously described.[Citation19] Real-time PCR was performed using a Mx4000 quantitative PCR system (Stratagene), with an initial denaturation step at 95 °C for 10 min, followed by 40 PCR cycles in which each cycle consisted of 95 °C for 30 s, 60 °C for 30 s and 72 °C for 30 s.
Results and discussion
Screening and identification of the gentiopicroside-producing yeast strain
Engineering yeasts for production of natural products using ion implantation-mediated DNA macromolecule transformation is a genetic transformation technique based on non-selectable markers.[Citation16] In addition, the introduction of foreign genetic material into yeasts by ion implantation is a random process, which generates a variety of individual transformants containing one to several genes involved in secondary metabolite biosynthesis or its regulatory genes in random or tandem arrangements after transformation. These features pose potential complications in the high-efficiency selection of the target recombinant yeast. As a result, a practicable and inexpensive screening method is required.
It is well known that IPP, a precursor of terpenoids, is formed through either the plastidial 2-C-methyl-D-erythritol-4-phosphate (MEP) pathway, or the cytosolic mevalonic acid (MVA) pathway in higher plants, whereas the MVA pathway is present in yeast.[Citation20] Therefore, we used the downstream genes of gentiopicroside biosynthesis that code for G10H and SLS as molecular markers to screen potential gentiopicroside-producing yeast strains. G10H is one of the key enzymes of the monoterpene indole alkaloid pathway, which catalyses the formation of 10-hydroxygeraniol. SLS catalyses the oxidative cleavage of the cyclopentane ring in loganin to form secologanin.[Citation21]
A total of 653 yeast transformants were screened for the presence of the G10H gene. Of these, 167 transformants had approximately 258 bp fragments of the G10H gene (). Then, 79 out of the 167 transformants had about 208 bp fragments of the SLS gene (). The 79 potential gentiopicroside-producing transformants were further analysed.
Figure 1. Representative PCR analysis of the G10H gene in yeast transformants. Lane M: 100 bp DNA Ladder Marker (Trans DNA Marker, TransGen Biotech, Inc., Beijing, China); Lane 1–7: genomic DNA of yeast transformants as the template; Lane 8: genomic DNA of G. macrophylla as the template; Lane 9: genomic DNA of control yeast cells treated with ion implantation and incubated in TE buffer without G. macrophylla genomic DNA as the template.
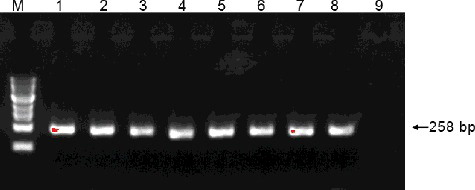
Figure 2. PCR analysis of the SLS gene in G10H-positive yeast transformants. Lane M: 100 bp DNA Ladder Marker (Trans DNA Marker, TransGen Biotech, Inc., Beijing, China); Lane 1: genomic DNA of the control yeast cells treated with ion implantation and incubated in TE buffer without G. macrophylla genomic DNA as the template; Lane 2: genomic DNA of G. macrophylla as the template; Lanes 3–9: genomic DNA of G10H-positive yeast transformants as the template.
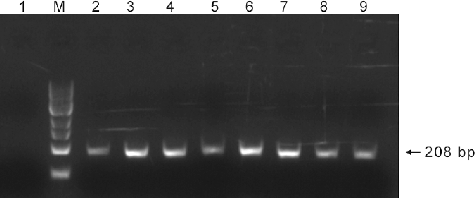
Since gentiopicroside is a secoiridoid glycoside including a normal glucose group, Fehling's test was employed to screen gentiopicroside-producing yeast strains. Meanwhile, to avoid the interference resulting from glucose, glycerol can be utilized as a carbon source in yeast cultivation. By screening with Fehling's test, three clones were screened from the 79 original PCR-positive transformants after exposure to ion implantation and incubation in TE buffer containing genomic DNA of G. macrophylla. Figure S1 (in the Online Supplementary Appendix) shows that after culture supernatants of these clones were treated with Fehling's solution, three positive results were indicated by the formation of a brick red precipitate, which is consistent with that of the gentiopicroside standard. In contrast, a negative result was obtained from the culture supernatants of both the yeast cells under vacuum conditions treated without ion implantation and incubated in TE buffer containing genomic DNA of G. macrophylla, and the control cells treated with ion implantation and incubated in TE buffer. In addition, these three screened clones were further subcultured for 20 generations in glycerol-containing YNB liquid medium in order to remove the unstable clones (data not shown). Consequently, one stable clone capable of maintaining a constant yield of gentiopicroside throughout successive generations was obtained and designated as DL67.
Accordingly, demonstrates that one spot from the sample solution of DL67 and a dark purple spot from the standard solution show the same colour tone and the same Rf of 0.61, whereas no similar spot from the sample solution of the control was observed. These results indicated that a novel secondary metabolite was produced by the DL67 strain.
Figure 3. Qualitative screening of the target gentiopicroside-producing transformant using TLC. (A) sample solution of gentiopicroside standard; (B) culture supernatants of the control yeast cells treated with ion implantation and incubated in TE buffer without G. macrophylla genomic DNA; (C) culture supernatants of strain DL67.
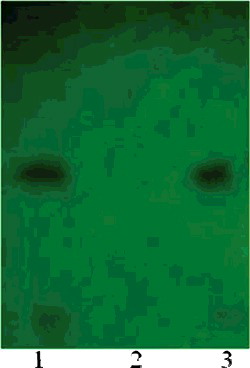
To further analyse the culture supernatants of strain DL67, the sample was analysed by HPLC-ESI-MS. The chemical molecular formula of gentiopicroside is C16H20O9 and its molecular weight is 356.32. (A,B) shows that the authentic gentiopicroside sample demonstrated a molecular ion peak at m/z 356.2, and the sample from strain DL67 also gave an ion fragment peak at m/z 356.2, which was identical with the [M-H]- of the gentiopicroside standard. In contrast, no corresponding molecular ion peak was observed in the HPLC-MS spectra of the sample of the control yeast cells treated with ion implantation and incubated with TE buffer without genomic DNA of G. macrophylla ((C)). In addition, the results showed that strain DL67 produced 8.41 mg/g DCW of extracellular gentiopicroside.
Figure 4. HPLC-MS spectra of the sample from authentic gentiopicroside. Sample solution of gentiopicroside standard (A); culture supernatants of strain DL67 (B); culture supernatants of the control yeast cells treated with ion implantation and incubated with TE buffer without G. macrophylla genomic DNA (C).
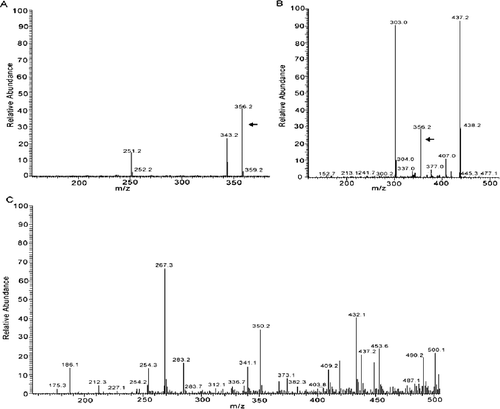
To further investigate the stability of gentiopicroside production by strain DL67, the strain was cultivated by flask fermentation for successive generations. shows that despite a slight reduction in gentiopicroside production over the first four generations, the gentiopicroside production of subsequent generations remained stable. The results demonstrated that strain DL67 is capable of maintaining a constant yield of gentiopicroside throughout successive generations.
Expression pattern of G10H and SLS genes of DL67 during growth
To understand how G10H and SLS are involved in gentiopicroside biosynthesis in the recombinant yeast, qRT-PCR was performed to quantify the expression of the G10H and SLS genes during growth. In this study, we designed specific primers for the G10H and SLS genes based on the resulting fragment identified by colony PCR, which was used to screen for the potential gentiopicroside-producing recombinant yeasts. Similarly, HPLC was performed to detect the gentiopicroside yield during growth. Our qRT-PCR results showed that the G10H and SLS genes were differentially expressed during growth (). Within the first 72 h of incubation, the G10H and SLS genes were found to be repressed. Expression was observed when the incubation was continued for 96 h, when the highest expression during the growth was observed. The gentiopicroside yield produced by DL67 was also shown to be the highest when DL67 was cultured for 96 h, and the corresponding G10H and SLS gene expression level reached a peak. Thus, these results suggest that the accumulation of gentiopicroside followed a trend that paralleled the regulation of G10H and SLS gene expression, thereby suggesting that the G10H and SLS genes may play important roles in gentiopicroside biosynthesis (). This also indicates that genomic DNA segments of G. macrophylla had been inserted into the genomic DNA of strain DL67.
Figure 6. Relationship between the expression of the G10H and SLS genes and the gentiopicroside yield in strain DL67 during the growth stage.
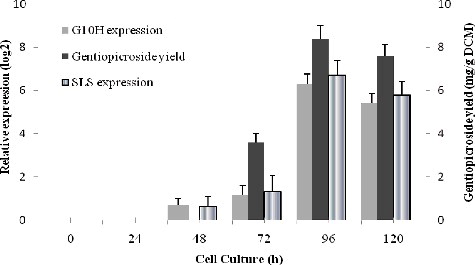
Overall, one stable gentiopicroside-producing recombinant yeast strain was obtained by ion implantation. However, previous research on heterologous gene expression in yeasts has shown that yeasts are incapable of correctly removing intervening sequences from transcripts of plants due to the differences between plant and yeast intron splicing.[Citation22] Interestingly, in the circumstances of ion beam-induced exogenous DNA transfer, the relevant genes, or gene clusters, involved in the biosynthesis of gentiopicroside in plants must have been successfully expressed in H. polymorpha. This phenomenon might be explained either by yeast alternative splicing,[Citation23] or by the biological effects induced by ion implantation, including energy absorption, mass deposition and charge transfer of energetic ions in organisms.[Citation16] A deeper insight into the molecular mechanism by which plant genes were correctly spliced in recombinant yeasts obtained by ion implantation is required to validate the method, which is currently under investigation.
In addition, the use of yeasts as host cells for production of medicinal plant secondary metabolites by conventional metabolic engineering remains challenging when the biosynthesis pathway and its regulatory genes are unknown. In addition, there are many ‘-omics’ level tools, such as transcriptomics and metabolomics, for studying the native physiology and genetics of yeast cells that can be used to investigate the global impact of the introduced enzymes on the cellular processes.[Citation24] Therefore, we strongly believe that once the construction of plant secondary metabolite biosynthesis in yeasts is achieved by ion-beam-induced gene transfer, the corresponding strains can be further employed to generate high-yield recombinant strains, using the tools available for construction and control of secondary metabolic pathways in yeasts. This would have a valuable application for low-cost production of natural compounds. Meanwhile, the recombinant strain obtained in this study can also be used for cloning and identifying enzyme genes related to metabolite biosynthesis by means of yeast genetic engineering systems.
Data analysis
Data are presented as means with standard deviations (±SD). Data analysis was performed using IBM SPSS Statistics 20.0 (IBM Corp., Armonk, NY, USA).
Conclusions
This study presents a novel-breeding strategy to engineer yeasts that produce gentiopicroside. One genetically stable gentiopicroside-producing recombinant yeast strain with a stable yield of 8.41 mg/g DCW of extracellular gentiopicroside was obtained at an energy level of 25 KeV and a dose of 2.5 × 1016 ions/cm2 using nitrogen ion beam radiation. This novel-breeding strategy could prove extremely effective for the production of commercially important gentiopicroside in terms of lower labour and production costs.
Disclosure statement
The authors declare that they have no conflict of interests concerning this paper.
Additional information
Funding
References
- Van Der Sluis WG, Van Der Nat JM, Labadie RP. Thin-layer chromatographic bioassay of iridoid and secoiridoid glucosides with a fungitoxic aglucone moiety using b-glucosidase and the fungus Penicillium expansum as a test organism. J Chromatogr. 1983;259:522–525.
- Ishiguro K, Yamaki M, Takagi S. Studies on the iridoid related compounds. I. On the antimicrobial activity of aucubigenin and certain iridoid aglycones. Yakugaku.Zasshi. 1982;102:755–759.
- Bhattacharya SK, Ghosal S, Chaudhuri RK, et al. Chemical constituents of Gentianaceae XI: antipsychotic activity of gentianine. J Pharm Sci. 1974;63:1341–1342.
- Kondo Y, Takano F, Hojo H. Suppression of chemically and immunologically induced hepatic injuries by gentiopicroside in mice. Planta Med. 1994;60:414–416.
- Jia N, Li Y, Wu Y, et al. Comparison of the anti-inflammatory and analgesic effects of Gentiana macrophylla Pall. and Gentiana straminea Maxim., and identification of their active constituents. J Ethnopharmacol. 2012;144:638–645.
- Tiwari RK, Trivedi M, Guang ZC, et al. Genetic transformation of Gentiana macrophylla with Agrobacterium rhizogenes: growth and production of secoiridoid glucoside gentiopicroside in transformed hairy root cultures. Plant Cell Rep. 2007;26:199–210.
- Bourgaud F, Gravot A, Milesi S, et al. Production of plant secondary metabolites: a historical perspective. Plant Sci. 2001;161:839–851.
- Baque MA, Moh SH, Lee EJ, et al. Production of biomass and useful compounds from adventitious roots of high-value added medicinal plants using bioreactor. Biotechnol Adv. 2012;30:1255–1267.
- Ro DK, Paradise EM, Ouellet M, et al. Production of the antimalarial drug precursor artemisinic acid in engineered yeast. Nature. 2006;440:940–943.
- Brown S, Clastre M, Courdavault V, et al. De novo production of the plant-derived alkaloid strictosidine in yeast. Proc Natl Acad Sci USA. 2015;112:3205–3210.
- Miettinen K, Dong L, Navrot N, et al. The seco-iridoid pathway from Catharanthus roseus. Nat Commun [ Internet]. 2014 [ cited 2015 Dec 26];5:3606. Available from: http://dx.doi.org/10.1038/ncomms4606
- De Luca V. Monoterpenoid indole alkaloid biosynthesis. In: Ashihara H, Crozier A, Komamine A, editors. Plant metabolism and biotechnology. New York (NY): Wiley; 2011. p. 263–292.
- Laule O, Fürholz A, Chang HS, et al. Crosstalk between cytosolic and plastidial pathways of isoprenoid biosynthesis in Arabidopsis thaliana. Proc Natl Acad Sci USA. 2003;100:6866–6871.
- El-Sayed M, Verpoorte R. Catharanthus terpenoid indole alkaloids: biosynthesis and regulation. Phytochem Rev. 2007;6:277–305.
- Tai HM, Huang MH, Yang CC. Formal total synthesis of (±)-dimethyl secologanoside. J Chin Chem Soc. 2003;50:441–444.
- Feng H, Yu Z, Chu P. Ion implantation of organisms. Mater Sci Eng R: Rep. 2006;54:49–120.
- Lü J, Jin X, Mao PH, et al. Transfer of Ephedra genomic DNA to yeasts by ion implantation. Appl Biochem Biotechnol. 2009;158:571–581.
- Rose TM, Henikoff JG, Henikoff S. CODEHOP(COnsensus-Degenerate Hybrid Oligonucleotide Primer) PCR primer design. Nucleic Acids Res. 2003;31:3763–3766.
- Manabe K, Kageyama Y, Morimoto T, et al. Combined effect of improved cell yield and increased specific productivity enhances recombinant enzyme production in genome-reduced Bacillus subtilis strain MGB874. Appl Environ Microbiol. 2011;77:8370–8381.
- Hua W, Zheng P, He Y, et al. An insight into the genes involved in secoiridoid biosynthesis in Gentiana macrophylla by RNA-seq. Mol Biol Rep. 2014;41:4817–4825.
- Yamamoto H, Katano N, Ooi A, et al. Secologanin synthase which catalyzes the oxidative cleavage of loganin into secologanin is a cytochrome P450. Phytochemistry. 2000;53:7–12.
- Langford C, Nellen W, Niessing J, et al. Yeast is unable to excise foreign intervening sequences from hybrid gene transcripts. Proc Natl Acad Sci USA. 1983;80:1496–1500.
- Sarmah B, Chakraborty N, Chakraborty S, et al. Plant pre-mRNA splicing in fission yeast, Schizosaccharomyces pombe. Biochem Biophys Res Commun. 2002;293:1209–1216.
- Michael S, Kate T Isis, Christina D. Advancing secondary metabolite biosynthesis in yeast with synthetic biology tools. FEMS Yeast Res. 2011;12:144–170.