ABSTRACT
The HSP90 is an abundant chaperone protein that is conserved in all eukaryotes. The main function of HSP90 is to assist other proteins to fold properly. In this study, we uncovered and analysed nine OsHSP90 (OsHSP90-1--OsHSP90-9) family members in rice Nipponbare, in which three distinct motifs were identified. All the HSP90 proteins were classified into three major groups (I, II, III) by phylogenetic analysis. The expression of OsHSP90 family in 10 tissues was examined by real-time polymerase chain reaction (PCR). OsHSP90-4, OsHSP90-6 and OsHSP90-7 had high expression, while OsHSP90-5 and OsHSP90-8 had very low expression across almost all 10 samples. The gene that encodes OsHSP90-1 was preferentially expressed in embryo at 14 days after flowering. It has been reported that some heat shock proteins were up-regulated in response to heat or other stresses. However, in our study the expression pattern of OsHSP90 genes is heterogeneous under a range of stress conditions. The expression of OsHSP90-2 and OsHSP90-4 was up-regulated under drought, salt, cold and heat conditions, while the expression of OsHSP90-3 and OsHSP90-5 was down-regulated under salt and drought conditions. OsHSP90-7 and OsHSP90-9 were down-regulated only under drought conditions. OsHSP90-6 did not change its expression across all conditions compared to control. Overexpression of OsHSP90-2 in E. coli could enhance cell viability and significantly improved resistance to heat, high salinity and drought stress conditions. The results presented here may provide new insights into the function of OsHSP90 family in rice.
KEYWORDS:
Introduction
HSP90 is a well-recognized chaperone protein, and is one of the most abundant proteins in cells, accounting for 1%–2% of all cellular proteins.[Citation1] It is considered as an essential protein that is conserved in all eukaryotes studied thus far.[Citation2–4] The main function of HSP90 is to assist other proteins to fold properly. Abnormal protein folding can lead to many problems in a cell, therefore all organisms have developed highly specialized mechanisms to maintain proteostasis and mitigate the life-threatening effects of various stresses.[Citation5,Citation6] HSP90 can help newly synthesized proteins to adopt their active conformations, mediate refolding of misfolded proteins and get rid of protein aggregates.[Citation6,Citation7] HSP90 usually exists as a dimmol/Ler as in most cases it needs to interact with a client protein and various numbers of co-chaperone proteins simultaneously. Each monomer of the HSP90 dimer comprises an N-terminal ATP-binding domain, a C-terminal dimerization domain and a middle domain that contains an amphipathic loop implicated in client protein-binding.[Citation8] There are more than 20 co-chaperones that HSP90 can interact with, which can guide HSP90 to recognize its client proteins and modulate the biochemical activities of HSP90.[Citation9] The client proteins of HSP90 include hormone receptors, protein kinases and transcription factors.[Citation10–12] HSP90 uses the energy generated by ATP-binding and hydrolysis to fold proteins involved in signal transduction, protein trafficking, receptor maturation and innate and adaptive immol/Lunity.[Citation2,Citation9,Citation13,Citation14]
Although HSP90 is constitutively expressed in unstressed cells, its expression increases in response to various stresses.[Citation15,Citation16] Plants possessed a complex heat stress response (HSR) network, in which the transcription factor heat stress factor (Hsf) regulates the expression of heat shock protein (HSP).[Citation17,Citation18] Plant Hsfs can be divided into three classes (class A, B and C) based on their specific domain.[Citation19] In particular, the three Hsfs in tomato (HsfA1a, HsfA2 and HsfB1) are considered to be among the most well-characterized Hsfs in plants with regard to their role in the HSR network.[Citation20,Citation21]
HSP90 plays an important role in plant disease resistance by forming a complex with another co-chaperones: SGT1 (suppressor of G2 allele of suppressor of kinetochore protein1) and RAR1 (required for MLA12 resistance1).[Citation22–24] It was speculated that SGT1 can mediate R-protein degradation. When SGT1 is part of the HSP90–RAR1–SGT1 complex, SGT1-mediated R-protein degradation might be weakened to allow disease resistance signal transduction to carry on.[Citation25,Citation26]
In Drosophila, it has been observed that decreasing the HSP90 level by genetic mutation or by treatment with the HSP90 inhibitor geldanamycin could cause developmental abnormalities and morphological changes.[Citation27] In Arabidopsis, reducing HSP90 function by treatment with geldanamycin gave rise to various morphological phenotypes.[Citation28] HSP90 was thus considered as a capacitor of phenotypic variation.[Citation28] HSP90 acts as a ‘buffer’ to sustain the function of those mutated proteins that participate in the signalling pathways of development and morphogenesis.[Citation29]
Recently, several successful studies of HSP90 have been reported in Nematodes, [Citation30] Arabidopsis, [Citation15,Citation16] human, [Citation31] wheat [Citation32] and model plants, respectively. Here, we analysed the protein sequence of the HSP90-encoding genes (the OsHSP90 family) in rice, their spatio-temporal expression profile, their expression pattern under various stresses and abscisic acid (ABA) and resistance to heat, high salinity and drought stress conditions in E. coli. The results may provide new insights into the function of the OsHSP90 family in rice.
Materials and methods
Database searches
The sequences of putative OsHSP90 family proteins in rice genome were obtained by searching the rice genome annotation project (RGAP version 7) database (http://rice.plantbiology.msu.edu/) with domain number PF00183. All the corresponding protein sequences of the putative HSP90 family members were then downloaded from RGAP and confirmed with the SMART database (http://smart.embl-heidelberg.de/smart/batch.pl). If there were several gene models at one locus, only the complete gene model was selected for further sequence analysis.
Sequence analysis
Information about the chromosomal localization, amino acid (aa) length, full-length cDNA accessions, and protein localization was obtained for each OsHSP90 gene from RGAP and the Knowledge-Based Oryza Molecular Biological Encyclopedia (KOME) (http://cdna01.dna.affrc.go.jp/cDNA). MEME version 4.9.1 (http://meme.nbcr.net/meme/) was used to predict motifs. Alignment of the protein sequences was performed using CLUSTAL_X version 1.83.[Citation33] A phylogenetic tree was constructed using MEGA4.[Citation34] Bootstrap testing was performed with 1000 resampling.
Plant growth and various stress and ABA treatment
Rice (Oryza sativa L. subsp. japonica cv. Nipponbare) seeds were germinated for three days, before seedlings were grown hydroponically in a 30 L vessel containing a nutrient solution consisting of 1.44 mmol/L NH4NO3, 0.3 mmol/L NaH2PO4, 0.5 mmol/L K2SO4, 1.0 mmol/L CaCl2, 1.6 mmol/L MgSO4, 0.17 mmol/L NaSiO3, 50 μM Fe-EDTA, 0.06 μM (NH4)6Mo7O24, 15 μmol/L H3BO3, 8 mmol/L MnCl2, 0.12 μmol/L CuSO4, 0.12 μmol/L ZnSO4, 29 μmol/L FeCl3, 40.5 μmol/L citric acid, pH 5.5.[Citation35] At the emergence of the fourth leaf, the seedlings of rice Nipponbare were subjected to various stresses and ABA treatment. For high salinity treatment, NaCl solution was added to achieve a final concentration of about 200 mmol/L. Drought stress was realized by putting intact plants in the air without water supply. For cold stress, the vigorous seedlings were transferred to a growth chamber at 4 °C (12-h-light/12-h-dark cycle). For heat stress, the seedlings of rice Nipponbare were subjected to 42 °C heat shock treatments. For ABA treatment, ABA was diluted to 100 μmol/L and sprayed onto the leaves of seedlings.
RNA extraction and real-time PCR
Samples were collected and ground to fine powder in liquid nitrogen. Total RNA was extracted with Trizol reagent (GIBCO/BRL) according to the manufacturer’s instructions. In the next step, the total RNA was treated with RNase-free DNase I (Invitrogen) for 15 min to degrade any potentially contaminated DNA. Real-time polymerase chain reaction (PCR) was performed on an optical 96-well plate with a Bio-Rad CFX96 real-time PCR system. Each reaction contained 5 µL of 2 X SYBR Green Master Mix Reagent (Roche), 1 µL of cDNA samples and 200 nM gene-specific primer in a final volume of 10 µL. The thermal cycle used was as follows: 95 °C for 10 min, followed by 39 cycles of 95 °C for 10 s, and then 60 °C for 30 s. The rice β-actin gene was used as an internal control in real-time PCR. The primers used in the OsHSP90 gene expression analysis are listed in Supplementary Table 1. The relative expression levels were determined as described previously.[Citation36]
Assay for abiotic stress tolerance of E. coli transformants
In order to construct the expression vector pET32a-OsHSP90-2, specific primers were designed as follows: sense, 5'-GGATCCGATGCGCAAGTGGGCGCTCTC-3' (BamHI site underlined) and antisense, 5'-AAGCTTCTACAGCTCGTCCTTATCAT-3' (HindIII site underlined). The amplified products were cloned into pET32a vector at BamHI--HindIII site to express the pET32a-OsHSP90-2 fusion protein, which has a Trx•Tag™ thioredoxin at the N-terminus.[Citation37] The transformed E. coli Rosetta cells were grown in Luria--Bertani (LB) liquid medium containing 100 µg /mL of ampicillin at 37 ℃ overnight, which were then inoculated into fresh LB medium (1:100 dilution) supplemented with ampicillin (100 µg /mL) to incubate until exponential growth phase (OD600 = 0.5–0.6). In order to induce the expression of the transformed gene OsHSP90-2, Isopropylthio-β-D-galactoside (IPTG) was added into the cultures. The concentrations of all induced cell cultures were adjusted to OD600 value of 1.0. For the assay of the heat tolerance, 2 mL samples were placed into 50 °C water bath. At 0, 1.5, 3 h after heat shock respectively, 10 μL of dilutions (1:10, 1:50, 1:100 and 1:200) was spotted onto LB agar plates with 1 mmol/L IPTG. For the assay of the salt tolerance, the samples were diluted 100-fold, 200-fold, 500-fold and 1000-fold with fresh LB medium containing ampicillin (100 µg/mL) and then 10 μL of the diluted samples was spotted onto LB agar plates containing 1 mmol/L IPTG supplemented with additional 200 and 400 mmol/L concentration gradient NaCl, respectively. For the assay of the drought tolerance, the samples were diluted by 100-fold, 200-fold, 500-fold, 1000-fold, and then 10 μL of the diluted samples was spotted onto LB agar plates containing 1 mmol/L IPTG supplemented with 300 and 600 mmol/L concentration gradient of mannitol, respectively.
Results and discussion
Identification of OsHSP90 family members in rice
We identified nine OsHSP90 gene family members () by searching the rice genome annotation project with domain number PF00183. The presence of these OsHSP90 genes in rice genome was then confirmed with the SMART database. The genes were named as OsHSP90-1–OsHSP90-9 according to their positions on pseudomolecules (). The nine members of the rice OsHSP90 are distributed on chromosome 4 (OsHSP90-1), 6 (OsHSP90-2), 8 (OsHSP90-3 and OsHSP90-4), 9 (OsHSP90-5, OsHSP90-6, OsHSP90-7 and OsHSP90-8) and 12 (OsHSP90-9), respectively.
Table 1. OsHSP90 gene family and their predicted protein structure information.
The nine members of this OsHSP90 family are distributed on 5 chromosomes. It has been reported that the rice genome includes 10 duplicated blocks accounting for 45% of its total size.[Citation38] Therefore, genome duplication might be the mechanism that underlies the distribution of OsHSP90 family members in different chromosomes. It is interesting that there are four OsHSP90 members (OsHSP90-5, OsHSP90-6, OsHSP90-7 and OsHSP90-8) are located in chromosome 9. Gene duplication events including segmental duplication, tandem duplication and transposition, are all important to the evolution of gene families.[Citation39] As OsHSP90-6 and OsHSP90-7 are adjacent to each other in terms to their genomic location (no more than 20 kb), and they are quite similar in length, it is likely that these two genes were the results of a segmental duplication event in the evolution of the genome.
Sequence analysis of OsHSP90 family
Nine full-length cDNAs corresponding to the nine OsHSP90 gene family members were found in KOME database (). The length of OsHSP90 proteins varies from 703 (OsHSP90-1) to 1046 (OsHSP90-8) amino acids. XPASY ProtParam analysis (http://web.expasy.org/protparam/) suggested that the OsHSP90 protein sequences have distinct theoretical isoelectric point (PI) values (ranging from 4.89 to 5.91 in), and molecular weights (ranging from 80.19 to 117.83 kDa) (Supplementary Table 2). Among the nine putative OsHSP90 genes, OsHSP90-1, OsHSP90-4, OsHSP90-6 and OsHSP90-7 were predicted to encode stable proteins while the product of the remaining genes was predicted to be unstable. XPASY ScanProsite analysis (http://prosite.expasy.org/prosite.htmL) revealed that eight of the nine OsHSP90 protein members harbour PS00298 (a signature sequence of the heat shock HSP90 protein family), whereas OsHSP90-8 was not found to bear this signature sequence.
The MEME motif search tool (http://meme.sdsc.edu/meme/) was used to examine the nine putative OsHSP90 gene sequences and three distinct motifs were identified (Supplementary Figure 1(A–C)). The conserved regions revealed by multiple sequence alignment analysis are in accordance with the locations of the three motifs. Notably, motifs 1 and 2 were found in all the OsHSP90 members. Motif 3 was also found in all OsHSP90 members except for OsHSP90-8 (Supplementary Figure 1(D)).
Phylogenetic analysis of OsHSP90 family
To examine the evolutionary relationships among the putative OsHSP90 family members in rice and Arabidopsis, a phylogenetic analysis was performed using MEGA4.[Citation34] Here four Arabidopsis HSP90 members, AT2G04030 (located in chloroplast), AT4G24190 (located in endoplasmic reticulum), AT5G52640 (located in cytosolic) and AT5G56030 (located in all tissues), were included as reference sequences. The results indicated that the OsHSP90 proteins could be classified into three major groups (I, II, III) (). Group I contained six members (OsHSP90-2, OsHSP90-3, OsHSP90-5, OsHSP90- 9, AT2G04030 and AT4G24190), group II contained six members (OsHSP90-1, OsHSP90-4, OsHSP90-6, OsHSP90-7, AT5G52640 and AT5G56030), whereas group III contained only one member (OsHSP90-8). Interestingly, genes belonging to group I are characterized by large number of introns (from 14 to 19), as opposed to genes belonging to group II that have small number of introns (from 1 to 3) (). It is also worth noting that all stable proteins, including OsHSP90-1, OsHSP90-4, OsHSP90-6 and OsHSP90- 7, fell into group II, a phenomena that was unexpected. The only member in group III is OsHSP90-8 that does not harbour motif 3 or the signature sequence PS00298.
Figure 1. Phylogenetic relationship of rice nine OsHSP90 members and Arabidopsis four HSP90 members. The unrooted tree was generated using MEGA4.0 program by maximum-likelihood method.
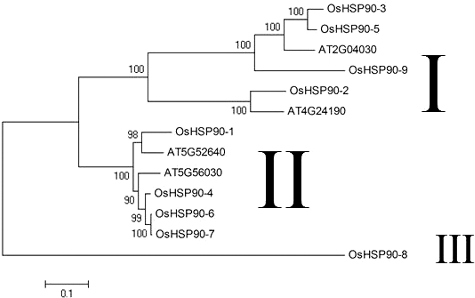
We further examined the subcellular localization of the nine rice OsHSP90 members with Softberry ProtComp (http://linux1.softberry.com/berry.phtmL). The results showed that OsHSP90-2, OsHSP90-3 and OsHSP90-5 were located in endoplasmic reticulum, while OsHSP90-1, OsHSP90-4, OsHSP90-6, OsHSP90-7 and OsHSP90-9 were located in cytoplasmic (). Interestingly, we found proteins with close phylogenetic relationships (OsHSP90-3 and OsHSP90-5, OsHSP90-2 and AT4G24190, OsHSP90-1 and AT5G52640, OsHSP90-6 and OsHSP90-7) tend to have the same subcellular localization. For example, both OsHSP90-3 and OsHSP90-5 were located in endoplasmic reticulum in group I, while both OsHSP90-6 and OsHSP90-7 were located in cytoplasmic in group II.
Expression patterns of OsHSP90 family members in various tissues
So far little is known about the expression patterns of OsHSP90 family members in various tissues of rice at different stages. To acquire more information in this regard, we examined the expression of the OsHSP90 family members in 10 tissues by real-time PCR. The results indicated that OsHSP90-4, OsHSP90-6 and OsHSP90-7 were consistently highly expressed, where OsHSP90-5 and OsHSP90-8 displayed extremely low expression level in almost all 10 samples (). It was suggested that OsHSP90-4, OsHSP90-6 and OsHSP90-7 play vital roles in all tissues at the stage of growth, development and maturation in rice. Furthermore, some lines that have T-DNA insertions in individual cytosolic HSP90 have been shown to still maintain a wild phenotype, indicating some degree of isoform redundancy.[Citation22,Citation25] Therefore, it is possible that OsHSP90-5 and OsHSP90-8 are two genes with redundant function. They may only function in the cases when the function of other OsHSP90 family members is compromised (e.g. by mutation). Furthermore, OsHSP90-1 was specifically expressed in embryo at 14 days after flowering as its expression was undetectable in other tissues or at other time points. It was speculated that the OsHSP90-1 was involved in rice reproduction development. The expression level of OsHSP90-3 and OsHSP90-9 was higher in tissues at ripening stage, such as embryo, endosperm and leaf blade at 14 days after flowering. The expression level of OsHSP90-2 was varied across tissues, high in leaf blade, root from plants with four tillers and in embryo and leaf blade at 14 days after flowering, but low in other tissues.
Figure 2. Real-time PCR analysis of OsHSP90 gene family members in rice Nipponbare different tissues. Ten representative tissues are as follows: Sd, three-leave seedlings; Lb, leaf blade from plants with four tillers; Ls, Leaf sheath from plants with four tillers; Stm, stem from plants with four tillers; Rt, root from plants with four tillers; at, 1.2--1.5 mmol/L anther; Pl, 5--10 cm panicle pistil; Eb, embryo 14 days after flowering; Esp, endosperm 14 days after flowering; Lbd, leaf blade 14 days after flowering. The rice β-actin gene transcript levels were used as internal controls. Error bars indicate SE based on three biological replicates.
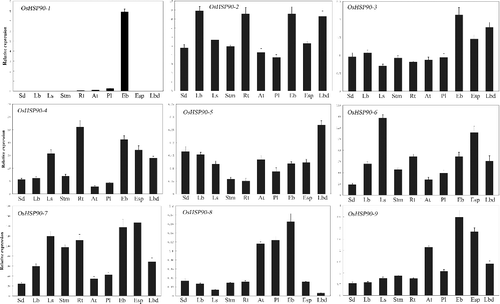
Expression analysis of OsHSP90 genes under various stress conditions
Some heat shock proteins were generally up-regulated under heat and other stresses.[Citation40] To check whether it is the case in rice, we performed real-time PCR to examine the expression of OsHSP90 family under heat and other stress conditions (). Note in this experiment, the seedlings of rice Nipponbare were subjected to various stresses and ABA treatment at the emergence of the fourth leaf. The results showed that the expression of OsHSP90-1 and OsHSP90-8 was consistently poor at the sampling time, under both normal and stress conditions, which was in line with the expression of Sd in . On the contrary, the expression level of OsHSP90-2 was significantly elevated under drought, salt, cold and heat conditions. The expression level of OsHSP90-3 and OsHSP90-5 displayed significant decrease under drought and salt conditions. The expression level of OsHSP90-4 was consistently increased under all conditions, including ABA. The expression level of OsHSP90-7 and OsHSP90-9 showed a significant drop under drought conditions. Interestingly, the expression level of OsHSP90-6 was approximately constant under various stress and ABA conditions. OsHSP90-6 could be considered as a kind of housekeeping gene and it might play an important role in maintaining the basic cellular function.
Figure 3. Relative expression levels of OsHSP90 gene family members in rice Nipponbare under various stress and ABA conditions detected by real-time PCR. At the emergence of the fourth leaf, the seedlings of rice Nipponbare were treated with drought, high salt, cold, heat and ABA. For salt stress and drought stress, seedlings were sampled at 0, 4, 8 and 16 h, respectively. For ABA, heat and cold stress, seedlings were sampled at 0, 1, 3 and 8 h, respectively. D, drought; S, salt; C; cold; H, heat; A, ABA. The rice β-actin gene transcript levels were used as internal controls. Error bars indicate SE based on three biological replicates.
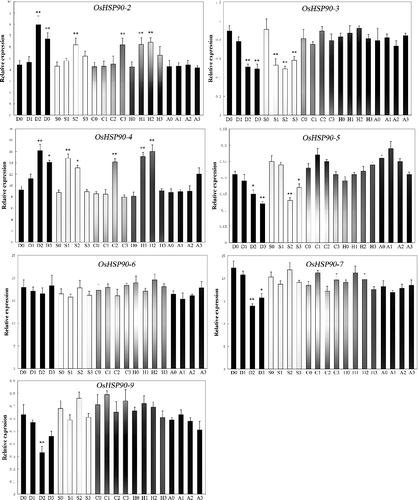
OsHSP90-2 improved stress resistance in transgenic E. coli
Based on the expression analysis of OsHSP90-2 under various stresses, we speculated that this protein might be involved in response to stress resistance. To test this hypothesis, we overexpressed this gene in E. coli and tested the resistance of this bacterium to stress. We determined the growth effect of E. coli recombinants overexpressing OsHSP90-2 under different abiotic stress (). Under normal conditions, the growth rate in E. coli transformed with pET32a-OsHSP90-2 was the same as E. coli transformed with pET-32a, whereas under heat conditions, the number of transgenic colonies (Rosetta/OsHSP90-2) was much higher than the numbers in the control (Rosetta/pET-32a) at 1.5, 3 h after treatment, respectively. In particular, at 3 h under heat conditions (200-fold), almost, there was no colony in the control cultures (5A). Under salt conditions, the number of transgenic colonies was significantly greater than that of control under various NaCl concentrations ((B)). Under mimic drought conditions, the number of transgenic colonies was also significantly greater than that of the control under various mannitol concentrations ((C)). There was no evident difference in the number of colonies between transgenic lines and the control under cold conditions (data not shown).
Figure 4. Growth effect of E. coli recombinants overexpressing OsHSP90-2 under different abiotic stresses. The concentrations of all induced cell cultures were adjusted to OD600 value of 1.0. The samples were diluted by 10-fold, 50-fold, 100-fold and 200-fold in heat test assay and by 100-fold, 200-fold, 500-fold and 1000-fold in mimic drought and salt test assay. Ten microliters of dilutions was spotted onto LB agar plates with 1 mmol/L IPTG. C indicated control, and here Rosetta/pET32a was used the control. O indicated Rosetta/OsHSP90-2 overexpressor. (A) At 0, 1.5 and 3 h after heat shock, respectively, (B) 200 and 400 mmol/L concentration gradient NaCl treatments, (C) 300 and 600 mmol/L concentration gradient of mannitol treatments (mimic drought). The experiment was repeated three times.
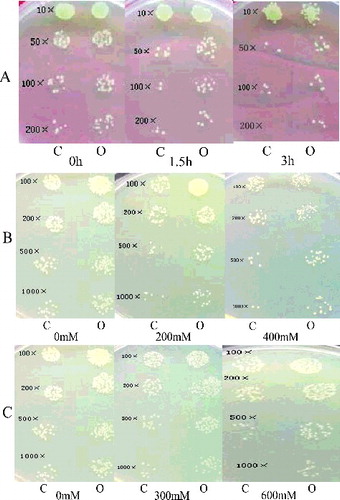
This indicates overexpression of OsHSP90-2 in E. coli may enhance cell viability. The expression level of OsHSP90-2 in rice was steady under ABA compared to normal conditions, which did not support a role of OsHSP90-2 in participating stress signalling. HSP90 was considered as a capacitor of phenotypic variation in Drosophila and Arabidopsis.[Citation27,Citation28] HSP90 acts as a ‘buffer’ to sustain the function of those mutated proteins.[Citation29] OsHSP90-2 might also act as a ‘buffer’ to counteract heat, high salinity and drought stresses in E. coli. Under the stress conditions, bacteria increased reactive oxygen species (ROS) generation, which could induce cell death.[Citation41,Citation42] It is therefore tempting to speculate that the overexpression of OsHSP90-2 resulted in the up-regulated expression of ROS scavenging enzyme genes, which lead to the enhanced ability of scavenging ROS.
Conclusions
This study provides not only important information regarding the OsHSP90 family members, but also showed the expression patterns of OsHSP90 family members in different tissues, and under various abnormal conditions including stress and ABA. Furthermore, this study suggested that OsHSP90-2 could improve resistance to various abiotic stresses in transgenic E. coli. It is possible that OsHSP90-2 might act as a ‘buffer’ to counteract heat, high salinity and drought stresses in E. coli. These data can provide important reference for further studies of the functions of OsHSP90 family and contribute to the genetic improvement of rice.
Suppl_mat_xu_Molecular_characterization.doc
Download MS Word (2.8 MB)Disclosure statement
No potential conflict of interest was reported by the authors.
Additional information
Funding
References
- Frydman J. Folding of newly translated proteins in vivo: the role of molecular chaperones. Annu Rev Biochem. 2001;70:603–647.
- Young JC, Moarefi I, Hartl FU. Hsp90: a specialized but essential protein-folding tool. J Cell Biol. 2001;154:267–273.
- Picard D. Heat-shock protein 90, a chaperone for folding and regulation. Cell Mol Life Sci. 2002;59:1640–1648.
- Wegele H, Muller L, Buchner J. Hsp70 and Hsp90–a relay team for protein folding. Rev Physiol Biochem Pharmacol. 2004;151:1–44.
- Balch WE, Morimoto RI, Dillin A, et al. Adapting proteostasis for disease intervention. Science. 2008;319:916–919.
- Hartl FU, Hayer-Hartl M. Converging concepts of protein folding in vitro and in vivo. Nat Struct Mol Biol. 2009;16:574–581.
- Doyle SM, Shorter J, Zolkiewski M, et al. Asymmol/letric deceleration of ClpB or Hsp104 ATPase activity unleashes protein-remodeling activity. Nat Struct Mol Biol. 2007;14:114–122.
- Pearl LH, Prodromou C. Structure and mechanism of the Hsp90 molecular chaperone machinery. Annu Rev Biochem. 2006;75:271–294.
- Taipale M, Jarosz DF, Lindquist S. HSP90 at the hub of protein homeostasis: emerging mechanistic insights. Nat Rev Mol Cell Biol. 2010;11:515–528.
- Pratt WB, Morishima Y, Osawa Y. The Hsp90 chaperone machinery regulates signaling by modulating ligand binding clefts. J Biol Chem. 2008;283:22885–22889.
- Riggs DL, Cox MB, Cheung-Flynn J, et al. Functional specificity of co-chaperone interactions with Hsp90 client proteins. Crit Rev Biochem Mol Biol. 2004;39:279–295.
- Wandinger SK, Richter K, Buchner J. The Hsp90 chaperone machinery. J Biol Chem. 2008;283:18473–18477.
- Richter K, Buchner J. Hsp90: chaperoning signal transduction. J Cell Physiol. 2001;188:281–290.
- Pratt WB, Krishna P, Olsen LJ. Hsp90-binding immunophilins in plants: the protein movers. Trends Plant Sci. 2001;6:54–58.
- Krishna P, Gloor G. The Hsp90 family of proteins in Arabidopsis thaliana. Cell Stress Chaperones. 2001;6:238–246.
- Milioni D, Hatzopoulos P. Genomic organization of hsp90 gene family in Arabidopsis. Plant Mol Biol. 1997;35:955–961.
- Mishra SK, Tripp J, Winkelhaus S, et al. In the complex family of heat stress transcription factors, HsfA1 has a unique role as master regulator of thermotolerance in tomato. Genes Dev. 2002;16:1555–1567.
- Kotak S, Port M, Ganguli A, et al. Characterization of C-terminal domains of Arabidopsis heat stress transcription factors (Hsfs) and identification of a new signature combination of plant class A Hsfs with AHA and NES motifs essential for activator function and intracellular localization. Plant J. 2004;39:98–112.
- Nover L, Bharti K, Doring P, et al. Arabidopsis and the heat stress transcription factor world: how many heat stress transcription factors do we need? Cell Stress Chaperones. 2001;6:177–189.
- Baniwal SK, Bharti K, Chan KY, et al. Heat stress response in plants: a complex game with chaperones and more than twenty heat stress transcription factors. J Biosci. 2004;29:471–487.
- Hahn A, Bublak D, Schleiff E, et al. Crosstalk between Hsp90 and Hsp70 chaperones and heat stress transcription factors in tomato. Plant Cell. 2011;23:741–755.
- Takahashi A, Casais C, Ichimura K, et al. HSP90 interacts with RAR1 and SGT1 and is essential for RPS2-mediated disease resistance in Arabidopsis. Proc Natl Acad Sci USA. 2003;100:11777–11782.
- Hubert DA, Tornero P, Belkhadir Y, et al. Cytosolic HSP90 associates with and modulates the Arabidopsis RPM1 disease resistance protein. EMBO J. 2003;22:5679–5689.
- Liu Y, Burch-Smith T, Schiff M, et al. Molecular chaperone Hsp90 associates with resistance protein N and its signaling proteins SGT1 and Rar1 to modulate an innate immune response in plants. J Biol Chem. 2004;279:2101–2108.
- Sangster TA, Queitsch C. The HSP90 chaperone complex, an emerging force in plant development and phenotypic plasticity. Curr Opin Plant Biol. 2005;8:86–92.
- Kitagawa K, Skowyra D, Elledge SJ, et al. SGT1 encodes an essential component of the yeast kinetochore assembly pathway and a novel subunit of the SCF ubiquitin ligase complex. Mol Cell. 1999;4:21–33.
- Rutherford SL, Lindquist S. Hsp90 as a capacitor for morphological evolution. Nature. 1998;396:336–342.
- Queitsch C, Sangster TA, Lindquist S. Hsp90 as a capacitor of phenotypic variation. Nature. 2002;417:618–624.
- Wang W, Vinocur B, Shoseyov O, et al. Role of plant heat-shock proteins and molecular chaperones in the abiotic stress response. Trends Plant Sci. 2004;9:244–252.
- Skantar AM, Carta LK. Molecular characterization and phylogenetic evaluation of the hsp90 gene from selected nematodes. J Nematol. 2004;36:466–480.
- Chen B, Piel WH, Gui L, et al. The HSP90 family of genes in the human genome: insights into their divergence and evolution. Genomics. 2005;86:627–637.
- Wang GF, Wei X, Fan R, et al. Molecular analysis of common wheat genes encoding three types of cytosolic heat shock protein 90 (Hsp90): functional involvement of cytosolic Hsp90s in the control of wheat seedling growth and disease resistance. New Phytol. 2011;191:418–431.
- Thompson JD, Gibson TJ, Plewniak F, et al. The CLUSTAL_X windows interface: flexible strategies for multiple sequence alignment aided by quality analysis tools. Nucleic Acids Res. 1997;25:4876–4882.
- Tamura K, Dudley J, Nei M, et al. MEGA4: Molecular Evolutionary Genetics Analysis (MEGA) software version 4.0. Mol Biol Evol. 2007;24:1596–1599.
- Yoshida S, Forno DA, Cook JH, et al. Laboratory manual for physiological studies of rice. Manila: International Rice Research Institute; 1976. pp. 61–67.
- Livak KJ, Schmittgen TD. Analysis of relative gene expression data using real-time quantitative PCR and the 2(-Delta Delta C(T)) ethod. Methods. 2001;25:402–408.
- LaVallie ER, DiBlasio EA, Kovacic S, et al. A thioredoxin gene fusion expression system that circumvents inclusion body formation in the E. coli cytoplasm. Biotechnology (N Y). 1993;11:187–193.
- Wang X, Shi X, Hao B, et al. Duplication and DNA segmental loss in the rice genome: implications for diploidization. New Phytol. 2005;165:937–946.
- Kong H, Landherr LL, Frohlich MW, et al. Patterns of gene duplication in the plant SKP1 gene family in angiosperms: evidence for multiple mechanisms of rapid gene birth. Plant J. 2007;50:873–885.
- Feder ME, Hofmann GE. Heat-shock proteins, molecular chaperones, and the stress response: evolutionary and ecological physiology. Annu Rev Physiol. 1999;61:243–282.
- Cabiscol E, Piulats E, Echave P, et al. Oxidative stress promotes specific protein damage in Saccharomyces cerevisiae. J Biol Chem. 2000;275:27393–27398.
- Kohanski MA, Dwyer DJ, Hayete B, et al. A common mechanism of cellular death induced by bactericidal antibiotics. Cell. 2007;130:797–810.