ABSTRACT
We have developed a technique for efficient transformation of hairy roots of Arachis hypogaea L. using Agrobacterium rhizogenes K599, and have validated this approach for the investigation of gene function. As a model transgene, AhAREB1, a drought-resistance gene from peanut, was fused to green fluorescent protein, and four parameters that might influence the transformation efficiency were tested. The optimal procedure involved the use of petioles with four expanded leaves as explants, infection by K599 at optical density (OD600) of 0.6 for 15 min and co-cultivation for 2 d, giving transformation efficiencies of up to 91%. Hairy roots from transgenic peanut plants overexpressing AhAREB1 were unaffected by treatment with polyethylene glycol (PEG), demonstrating increased drought tolerance, whereas control roots showed clear signs of plasmolysis. Transgenic roots accumulated less superoxide anion (O2 −) than control roots under drought conditions. Additionally, transgenic roots displayed upregulation of four stress-response genes encoding WRKY transcription factor (WRKY33), MYB transcription factor (MYB92), abscisic acid receptor (PYL5) and dehydrin 2 (DHN2).
Introduction
Peanut (Arachis hypogaea L.) is widely grown in more than 100 countries and it is one of the most economically important crops in the world. Cultivated peanuts are used as food for human consumption, as forage for animal production and for the production of edible oil. In China, 70% of the peanut production areas suffer from varying degrees of water deficit and this seriously affects the peanut yield and quality.[Citation1,Citation2] Therefore, research into enhancement of drought resistance in peanut plants is necessary and urgent. However, because of the complicated peanut genome (tetraploid) and lack of effective transgenic techniques for this species, little is known about how it responds to drought stress at the molecular level.
Genetic transformation is a powerful tool for the investigation of gene function in plants, but in some systems its impact is reduced by limited transformation capability.[Citation3] With legumes, for example, Agrobacterium tumefaciens-mediated transformation has been used for the study of gene function,[Citation4] but many species are either resistant to this technology or display low transformation efficiency.[Citation5]
An alternative to A. tumefaciens-mediated transformation involves Agrobacterium rhizogenes, a soil-borne Gram-negative bacterium that induces hairy roots by infection of wounds in both monocotyledons and dicotyledons. Genetic material can be readily introduced into the host cell via A. rhizogenes, because its root-inducing plasmid (Ri plasmid) includes transfer DNA (T-DNA), which encodes the root locus (rol) gene loci, rolA, rolB and rolC.[Citation6,Citation7] Hairy roots grow rapidly and plagiotropically and develop many branches without the need for exogenous plant growth regulators in vitro.[Citation8] Hairy roots can also be propagated in axenic culture,[Citation9] with each transgenic hairy root representing an independent transformation event.[Citation10] Compared to A. tumefaciens-mediated transformation, one significant advantage of A. rhizogenes-mediated transformation is the relatively short time required to identify the transgene in transformants.[Citation11] Therefore, A. rhizogenes-mediated transformation constitutes a simple, rapid and efficient method for research into gene function and root biology.[Citation12]
Over 100 species of plants have been transformed using A. rhizogenes,[Citation13] and it has been of particular value for those species that are recalcitrant to other methods, such as Pogostemon cablin [Citation14] and Phtheirospermum japonicum.[Citation15] In legumes like soybean, transgenic hairy roots have been used to study gene function.[Citation16,Citation17] There are also reports on A. rhizogenes-mediated transformation of peanut,[Citation18,Citation19] but little information on the factors that influence the transformation efficiency. In the present study, we establish a more efficient transformation system by optimizing parameters such as A. rhizogenes infection time [Citation20] and co-cultivation time.[Citation21] To further identify whether this system can be used to investigate gene function, the peanut gene AhAREB1 was introduced into the hairy roots of A. hypogaea to evaluate drought tolerance. AhAREB1 encodes a basic-domain leucine zipper (bZIP) transcription factor that acts as a key positive regulator of the abscisic acid (ABA) signal transduction pathway involved in plant tolerance to drought.[Citation22–24] The effects of this transgene were assessed by phenotypic observation of plasmolysis, histochemical staining and real-time polymerase chain reaction (PCR) analysis of four functional genes, i.e. the stress-related transcription factor genes, WRKY33 and MYB92, the abscisic acid receptor gene PYL5 and the late embryogenesis abundant (LEA) protein gene DHN2,[Citation25,Citation26] in hairy roots under water stress conditions.
Materials and methods
Plant materials and growth conditions
Peanuts (Arachis hypogaea L. cv Yueyou 7) were purchased from Crops Research Institute Guangdong Academy of Agricultural Sciences. The seeds were soaked in water for 12 h. They were then surface-sterilized with 10% (v/v) sodium hypochlorite for 7 min and with 70% ethanol for 1 min. After three washes with sterile water, the cotyledons of the sterilized seeds were separated with a scalpel and those with an intact embryo were sown on solid hormone-free Murashige and Skoog (MS) [Citation27] medium supplemented with 2% (w/v) sucrose and 0.8% (w/v) agar. The seeds were allowed to germinate and were grown at 28 °C with a 16 h light/8 h dark photoperiod in a growth room. For transformation experiments, explants were excised from sterile seedlings.
Agrobacterium strain and binary vector
The cucumopine-type A. rhizogenes strain K599 was used to induce transgenic hairy roots in peanut. The AhAREB1 cassette was released from pBI121-AhAREB1, which was previously constructed by the pBI121-vector with AhAREB1 (Gene ID: JF766570.1), by double digestion with Xba I and Sma I and cloned into the Xba I/Sma I sites of the binary vector pGFP, under the control of the cauliflower mosaic virus (CaMV) 35S promoter. The resulting binary construct 35S::AhAREB1-GFP was transformed into A. rhizogenes strain K599 by the freeze-thaw method. The neomycin phosphotransferase gene (NPTII), under the control of the nopaline synthase (NOS) promoter, located within T-DNA enables positive transformants to be selected by kanamycin. A. rhizogenes K599 without the binary vector was used as a negative control.
Induction of peanut hairy roots
A. rhizogenes bacteria were prepared for peanut infection using a protocol modified from that originally developed for soybean transformation.[Citation10] Briefly, a single colony of A. rhizogenes K599 carrying 35S::AhAREB1-GFP was inoculated in 1 mL of liquid yeast extract peptone (YEP) medium with 50 mg/L kanamycin, then cultured overnight and diluted in 100 mL of liquid YEP medium [Citation28] at 28 °C with shaking at 180 r/min. After growth to the desired concentration, bacteria were centrifuged at 1500 × g and resuspended in 50 mL of half-strength MS medium for infection.
Segments of different explants from 10- to 12-day-old A. hypogaea plants were cut with a scalpel to generate several 0.5-cm-long wounds. They were then immersed in A. rhizogenes suspensions and incubated for a certain time (see below). The infected explants were dried on sterile 240 mm × 170 mm filter paper and transferred to solid MS medium without hormone. After co-cultivation for various periods (see below) in the dark, these explants were immersed in sterile water with 250 mg/L cefotaxime for 5 min and washed three times with sterile water. The dried explants were then transferred to solid MS medium containing 250 mg/L cefotaxime for hairy root induction. After about four weeks, hairy roots growing at the wound sites were cut off and transferred to solid half-strength MS medium with 250 mg/L cefotaxime (Gen-View Scientific, Inc., Florida, USA), 100 mg/L kanamycin (Gen-View Scientific, Inc.) and 1% activated carbon (to prevent browning) for root propagation.
Confocal microscopy
Green fluorescent protein (GFP) fluorescence in the hairy root tips was observed with a confocal laser scanning microscope (LSM710, Carl Zeiss, Germany). The cells of hairy roots were stained with propidium iodide.
Detection of GFP in transgenic hairy roots by PCR
Total genomic DNA was extracted from 100 mg of hairy roots [Citation29] and GFP sequences were amplified by PCR (MJ Mini Thermal Cycler, BIO-RAD) using the specific primer (Table S1 in the Online Supplementary Appendix). Amplification was performed with a hot start at 94 °C for 2 min, then 36 cycles of denaturation (98 °C, 10 s), annealing (55 °C, 30 s) and extension (68 °C, 1 min), and a final extension for 5 min at 68 °C. The products were visualized by 1% agarose gel electrophoresis (BG-POWER300, BAYGENE, Beijing, China).
Protein extraction and Western blot for GFP detection
Hairy roots were ground into homogenized powder in liquid N2 and the powder was quickly transferred into 1 mL extraction buffer (KGP750, KeyGEN, China) comprising 1 mL lysis buffer, 1 μL protease inhibitor, 10 μL dithiothreitol DTT (1 mol/L) and 10 μL phenylmethanesulfonyl fluoride PMSF (100 mmol/L). Then the tube was shaken vigorously three times every 10 min at 4 °C. After homogeneous mixing, the sample was centrifuged at 13,000 r/min for 10 min and its supernatant used in Western blot assays by first boiling for 10 min with 5× Dual Color Protein Loading Buffer, and separating in 12% sodium dodecyl sulphate (SDS)-polyacrylamide gels (BIO-RAD, USA). Proteins were then transferred to a polyvinylidene fluoride (PVDF) membrane (Millipore Immobilon®-P, USA) and GFP was detected by polyclonal anti-GFP antiserum (Abcam, UK).
Evaluation of parameters influencing the transformation efficiency
Four parameters were assessed: type of explant (hypocotyl, leaf, petiole); optical density (OD600) value of the A. rhizogenes cell culture (0.2, 0.4, 0.6, 0.8 and 1.0); infection time (5, 10, 15, 20 min) and duration of co-cultivation (1, 2, 3 and 4 d). The protocol for hairy root induction was followed in all optimization assays as described above and the only variable was the parameter to be optimized. The transformation efficiency was given by the percentage of GFP-positive hairy roots. All parameters were tested in three independent transformation experiments and each experiment included a minimum of 20 explants. Data are presented as means with standard deviation (±SD). The effects of the studied parameters on the transformation efficiency were verified by Fisher's LSD (least significant difference) test at the P < 0.05 level.
RNA isolation and reverse transcriptase PCR for AhAREB1 detection
Transgenic AhAREB1 A. hypogaea hairy roots were obtained using the improved transformation system. To confirm the transgenic event, the expression levels of AhAREB1 were tested by reverse transcriptase PCR (RT-PCR) using 18S RNA as an internal reference. Total RNA was extracted from four randomly selected AhAREB1 transgenic roots identified as GFP-positive and one control hairy root using TRIzol Reagent (Takara, Japan), according to the manufacturer's instructions. After extraction, DNase-treated RNA was used to carry out cDNA synthesis using the Reverse Transcriptase murine leukemia viruses (MLV) system (Takara, Japan) with oligo(dT)12-18 primer. The cDNA sequences of 18S and AhAREB1 were detected by PCR using two gene-specific primers, 18S-F and -R, AhAREB1-RT-F and -R (Table S1 in the Online Supplementary Appendix). We used 30 PCR cycles for 18S and 36 cycles for AhAREB1.
Real-time quantitative PCR (RT-qPCR)
We identified transgenic peanut hairy roots overexpressing AhAREB1 by monitoring the AhAREB1 expression levels using RT-qPCR with ACTIN as an internal reference. RNA was isolated as described above and 500 ng of total RNA was DNaseI-treated and used for reverse transcription with the PrimeScript RT reagent Kit with gDNA Eraser (Perfect Real Time, TaKaRa, Japan) for the first-strand cDNA synthesis, according to the manufacturer's instructions. RT-qPCR was performed by an Optical 96-well Fast Thermal Cycling Plate with an ABI 7500 real-time PCR system (Applied Biosystems, USA) using two gene-specific primers, ACTIN-F and -R, AhAREB1-RealT-F and -R (Table S1 in the Online Supplementary Appendix). ACTIN was used for an internal control gene. Each reaction contained 10 µL of 2× SYBR® Premix DimerEraser and 0.4 µL of ROX Reference Dye II (TakaRa, Japan), 2 µL of cDNA and 1.2 µL of primers in a final volume of 20 µL. The thermal cycle used was 95 °C for 30 s, then 40 cycles at 95 °C for 5 s and 60 °C for 34 s. Four functional genes, WRKY33, MYB92, PYL5 and DHN2,[Citation23,Citation24] were used for quantitative tests with gene-specific primers (Table S1 in the Online Supplementary Appendix). The relative expression levels were calculated using the 2−ΔΔ CT equation.[Citation30] Three replicates were performed for each experiment. All RT-qPCR data were expressed as means ± SD.
Phenotype observation in hairy roots treated with polyethylene glycol
To evaluate the drought resistance of transgenic peanut hairy roots overexpressing AhAREB1, transgenic and control roots were immersed in 10% PEG containing methylene blue for 1.5 h. Plasmolysis phenotypes were assessed using an inverted microscope (Leica DMI3000 B, Germany).
Nitro blue tetrazolium staining in hairy roots treated with PEG
In situ accumulation of superoxide (O2 –) was detected by histochemical staining with NBT. Three-centimetre-long samples of transgenic hairy roots treated with 20% PEG for 3 h were immersed and incubated in 1 mg/mL of fresh NBT solution (prepared in 10 mmol/L phosphate buffer, pH 7.8) at 25 °C until deep blue spots appeared. Samples were then washed in 70% ethanol [Citation23] and viewed under a stereoscopic microscope (SteREO Discovery V8, Carl Zeiss, Germany).
Results and discussion
Transgenic hairy roots induced by A. rhizogenes with high efficiency
Petioles with four expanded leaves were cut after pre-culture and incubated with A. rhizogenes (OD600 0.6) for 15 min to induce infection ((A)); bacteria containing the binary vector 35S::AhAREB1-GFP were used to create transgenic hairy roots, while control bacteria lacked the vector. After co-cultivation for 2 d, the explants were removed and placed on solid MS medium containing 250 mg/L cefotaxime. After 17–20 d, white tips appeared at the wound sites of the explants ((B) and (C)), which grew into hairy roots 2–3 cm in length ((D)). An approximately 100 μg fragment was cut from these hairy roots and tested for the presence of GFP by fluorescence microscopy and genomic PCR: transgenic roots should contain an AhAREB1-GFP fusion protein. GFP-positive transgenic hairy roots were then excised at a point 4–5 cm from the main plant ((E)) and transferred to propagation medium ((F)). Transgenic A. hypogaea hairy roots were ready for analysis after about two months.
Figure 1. Transgenic A. hypogaea hairy roots obtained by A. rhizogenes-mediated transformation. Co-cultivation (A) of explants after infection with A. rhizogenes. Hairy roots (B) began to appear at explant wound sites, (C) about 17–20 d after transfer onto MS medium. Image (D) 10 d after the first appearance of hairy roots. Composite plant (E) 30 d after the first appearance of hairy roots. Hairy roots (F) were cut and transferred onto solid half-strength MS medium for propagation over about three weeks.
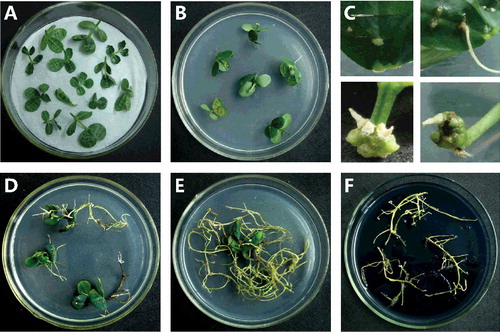
Molecular identification of transgenic hairy roots
(A) shows the binary vector 35S::AhAREB1-GFP. Representative GFP-positive and control hairy roots are shown in (B) and (C), respectively. As AREB1 is a nuclear protein, the GFP fluorescence of the fusion protein can be seen in the nuclei of transgenic root tip cells. The presence of the GFP gene in transgenic roots was confirmed by PCR ((D)).To further verify that transgenic hairy roots contain the AhAREB1-GFP fusion protein, we carried out Western blot analysis using GFP-specific antibodies. Bands representing the fusion protein (about 75 kDa (GFP: 27 kDa; AhAREB1: 48 kDa)) were detected in six independent transgenic hairy roots, but not in the control ((E)). We were able to obtain positive transgenic hairy roots of A. hypogaea through these identification methods.
Figure 2. GFP detection of transgenic AhAREB1 hairy roots. Schematic representation (A) of the T-DNA region of 35S::AhAREB1-GFP. GFP-derived fluorescence detected by laser scanning confocal microscopy, (B) in a transgenic hairy root, and (C) in a negative control hairy root; red bars correspond to 50 μm. PCR amplification of GFP, (D) in hairy roots; M, 2 kb DNA marker (Takara); −, negative control; +, plasmid DNA; 1–10, transgenic hairy roots. Western blot assay (E) for detection of GFP-AREB1 fusion protein in independent transgenic hairy roots, using anti-GFP antibodies; -, negative control root; 1–7, transgenic hairy roots.
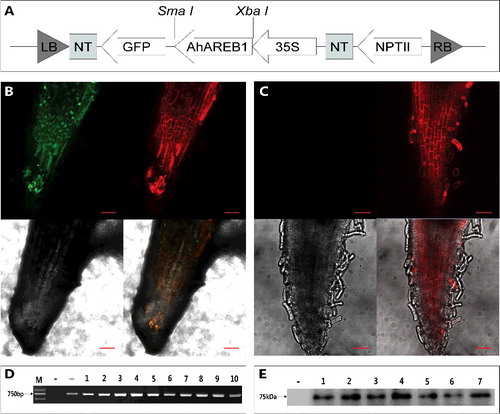
Effects of parameters on transformation efficiency
The transfer of T-DNA from A. rhizogenes into plant cells with high efficiency is a key procedure in the production of transgenic hairy roots.[Citation31] This process is complex and can be affected by various parameters such as A. rhizogenes strain,[Citation32] infection time [Citation20] and duration of co-cultivation.[Citation21]
The A. rhizogenes transformation efficiency varied with the type of explant used ((A)) and was highest (90.46%) with petioles (P < 0.05). The transformation efficiency was just 14.49% when hypocotyls were used. This suggests that the petiole with four expanded leaves gave the highest transformation efficiency and is the most appropriate explant of those tested for A. rhizogenes-mediated transformation of peanut. This was used subsequently to measure the effects of other parameters on efficiency. As the infection time was increased from 5 to 15 min, the transformation efficiency increased accordingly ((B)). Explants infected for 15 min gave a transformation rate of 89.77%, and this was significantly different from other infection times (P < 0.05). When the infection time was increased further to 20 min, however, there was a slight reduction in the transformation efficiency to 77.96%. Thus, we used a 15 min infection time in subsequent experiments. The density of the A. rhizogenes culture may also affect its virulence, thus influencing the transformation efficiency. To evaluate this, petioles with four expanded leaves were infected for 15 min with different concentrations of A. rhizogenes. As shown in (C), the highest transformation efficiency (89.50%) was achieved when the OD600 of the infecting bacterial culture was around 0.6 (P < 0.05). After infection, the explants were placed on solid MS medium without hormone to allow transfer of T-DNA into explant cells and were co-cultivated for up to 4 d. Co-cultivation for 2 d gave the highest transformation efficiency (91.41%), whereas the efficiencies were lower with both shorter and longer periods of co-cultivation (P < 0.05) ((D)).
Figure 3. Factors influencing the efficiency of A. rhizogenes-mediated transformation of peanut. Effect of: explant type (A); infection time (B); A. rhizogenes cell density (OD600) (C); duration of co-cultivation (D).
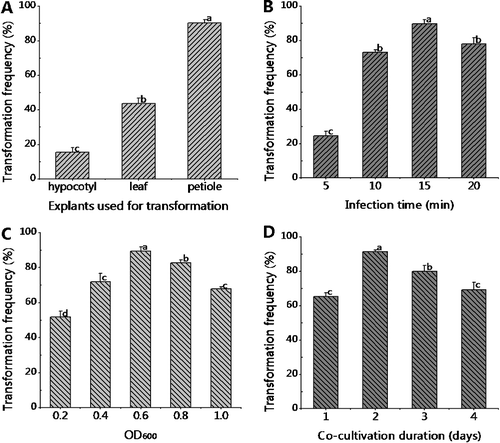
In the present study on the application of this technique to peanut (A. hypogaea), petioles with four expanded leaves were therefore selected as the preferred type of explant ((A)). This suggests that the choice of explant is also a critical parameter in optimizing T-DNA transfer from A. rhizogenes, perhaps because the physiological status of different explants influences the transformation efficiency. An infection time of 15 min was found to be optimal for transformation, although 10 min also gave appreciable success ((B)). A long infection time allows more bacteria to adhere to the surface of wound sites, thereby improving the chance of transgene insertion into the explant genome. However, increasing the immersion to 20 min resulted in a decrease in efficiency, perhaps because of a hypersensitive response of explants to bacteria.[Citation33] Bacterial concentration plays a role in transformation systems. In A. rhizogenes-mediated transformation, target tissues are infected with a fresh suspension culture of bacteria. Our results showed that treatment of petioles with A. rhizogenes suspension at an OD600 of 0.2, 0.4 and 0.6 generated a steadily increasing transformation efficiency ((C)). Although denser A. rhizogenes suspensions (OD600 = 0.8 and 1.0) should increase bacterial attachment further, it may cause contamination of the explant by A. rhizogenes itself. Exposure to high levels of bacteria can cause irreversible physiological perturbation resulting in browning of tissues and unsuccessful recovery of transformed cells.[Citation34] At the other extreme, the transformation efficiency was low when suspensions with OD600 0.2 were used because there are insufficient A. rhizogenes cells to infect and transfer T-DNA into explant cells.[Citation35] The duration of co-cultivation can also have a significant effect on transformation efficiency. In the present study, 2 d was identified as the optimal co-cultivation time. With a longer period of 3 or 4 d, A. rhizogenes may overgrow, leading to explant cell damage and, consequently, to low transformation efficiency ((D)). In contrast, a shorter co-cultivation time, such as 1 d, may not allow sufficient A. rhizogenes cell proliferation, resulting in reduced virulence and low transformation efficiency. These results are in accordance with reports in other types of legume, such as Vigna mungo [Citation36] and Lathyrus sativus.[Citation21] Thus, the A. rhizogenes-mediated transformation efficiency of peanut hairy roots reached as high as 91% under optimal conditions.
Evaluation of the transformation system for the study of gene function
To evaluate the suitability of the A. rhizogenes-mediated transformation system for research into gene function, we created transgenic hairy roots from peanut that overexpress AhAREB1. AhAREB1 is a peanut gene encoding a transcription factor that operates as a key positive regulator of the ABA signal transduction pathway, which regulates plant tolerance to drought.[Citation22,Citation23] In this study, A. rhizogenes K599 [Citation5,Citation8,Citation10] harbouring 35S::AhAREB1-GFP was used, allowing simple validation of transgenic hairy roots by screening for a functional GFP reporter gene. Using the optimum conditions outlined above for the four parameters investigated, a transformation efficiency of 91% could be achieved, yielding transgenic A. hypogaea hairy roots in two and a half months. Eight GFP-positive transgenic hairy roots were randomly selected and the expression of AhAREB1 was tested by RT-PCR, with the 18S rRNA gene as a reference gene. As shown in (A), all eight transgenic lines expressed AhAREB1. To identify hairy roots overexpressing AhAREB1, RT-qPCR was used to quantitate the expression levels of the transgene, with peanut ACTIN mRNA used as an internal reference for normalization. The expression of AhAREB1 was higher than the control in four transgenic lines, of which line 4 showed the highest level ((B)). Roots overexpressing AhAREB1 could be identified and obtained by RT-PCR and RT-qPCR.
Figure 4. Production of transgenic AhAREB1 roots. Expression analysis (A) of AhAREB1 in different peanut transgenic lines by RT-PCR. A specific PCR product of 1338 bp (upper panel) was detected in eight randomly selected AhAREB1 (1–8) transgenic lines identified as GFP-positive; +, plasmid DNA. An 18S fragment was amplified as an internal control (lower panel). Real-time PCR analysis (B) of expression of AhAREB1 in negative control (CK) and transgenic lines (AhAREB1-3, 4, 5, 7), selected by RT-PCR analysis above, under normal conditions.
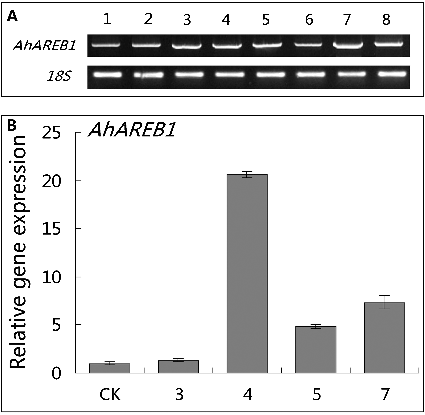
To test the drought tolerance of overexpressing transgenic roots, 10 samples of each independent line, together with the negative control, were examined under water stress conditions. An example is shown in (A), where after about 1.5 h plasmolysis is apparent in the cells of control root tips treated with 10% PEG. In contrast, the transgenic AhAREB1 hairy roots appeared normal under water stress. We also used NBT staining to examine the levels of O2 –, an important reactive oxygen species (ROS), in these roots under regular growth and drought stress conditions. There was no difference in O2 – levels (visualized as deep blue spots) between transgenic and control roots under control conditions. However, after treatment with 10% PEG for 3 h, the levels of O2 – increased in both control and transgenic roots, but to a greater extent in controls ((B)). The level of superoxide anion (O2 –) directly reflects the oxidative stress-resistance of various crops, and therefore it can be used as an index to assess drought tolerance.[Citation37,Citation38] Appropriate regulation of ROS levels is significant to enhance the resistance to abiotic stress. In transgenic Arabidopsis, AhAREB1 can modulate ROS accumulation levels and thereby improve drought tolerance, as shown in our previous study.[Citation23] Here, the antioxidative capacity of AhAREB1-overexpressing transgenic hairy roots, reflected by decreased accumulation of O2–, was stronger than in controls during water stress ((B)). These results suggested that overexpression of AhAREB1 has a protective effect in transgenic hairy roots.
Figure 5. Effect of water stress on transgenic AhAREB1 (35S::AhAREB1) and negative control (CK) hairy roots. Plasmolysis (A) of CK hairy root tips (arrows), but not of normal transgenic hairy root tips after treatment with 10% PEG containing methylene blue for 1.5 h; white bars correspond to 50 μm. Histochemical staining (B) by NBT to reveal accumulation of O2 – after treatment with 10% PEG for 3 h; red bars correspond to 250 μm. RT-qPCR expression analysis (C) of AhAREB1 and stress-response genes (WRKY33, MYB92, PYL5, DHN2) after treatment with 20% PEG for 0 h or 2 h; numbers 1–5 represent different lines.
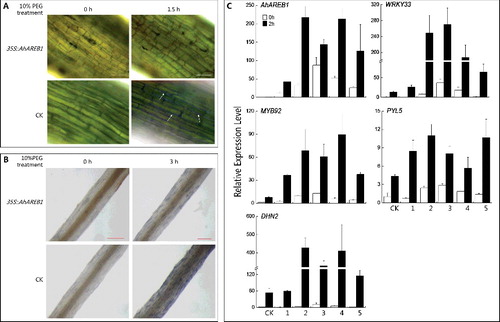
To identify the impact of AhAREB1 overexpression on gene regulation in response to drought, the expression of AhAREB1 and four functional genes whose expression is associated with drought tolerance in plants, i.e. WRKY transcription factor (WRKY33), MYB transcription factor (MYB92), abscisic acid receptor (PYL5) and dehydrin 2 (DHN2),[Citation25,Citation26] were examined in these roots, using RT-qPCR. Treatment with 20% PEG for 2 h induced upregulation of all five genes in both control and transgenic roots, but the mRNA levels of the five genes were higher in the five AhAREB1-overexpressing transgenic roots than in controls ((C)), indicating that these genes were strongly induced in transgenic roots under drought stress. These results demonstrated that overexpression of AhAREB1 in transgenic peanut hairy roots can modulate transcription levels of resistance-related genes.
A. rhizogenes-mediated hairy roots have been used for studies in physiology, secondary metabolism and root biology.[Citation16–18] The development of an effective transgenic technique will promote physiological and molecular biology research in A. hypogaea.[Citation19] Compared to previous studies by microinjected embryonic axes to induce hairy roots,[Citation39,Citation40] our technique enhanced the rooting rate by infection of several long wounds of the petiole with four expanded leaves which had higher ability for tissue differentiation. In this study, we have developed a fast and efficient platform for the study of peanut gene function in transgenic hairy roots (), and have validated the method by creating roots that overexpress AhAREB1. However, some challenges remain. For example, A. hypogaea is a heterozygous tetraploid, and therefore the regeneration of transgenic peanut plants from transgenic peanut hairy roots is a problem that requires further research in future.
Conclusions
In this study, a more efficient transformation system for the study of gene function in hairy roots of peanut using A. hypogaea was established by optimizing parameters. The four optimal parameters involved the use of petioles with four expanded leaves as explants, infection by K599 at OD600 of 0.6 for 15 min, and co-cultivation for 2 d. We identified this transformation system for research into peanut gene function in transgenic hairy roots by creating transgenic roots that overexpress AhAREB1. Transgenic roots demonstrated increased drought tolerance and displayed upregulation of four stress-response genes. These findings will help to provide a simple and rapid method for gene function analysis of peanut.
Supplementary Material
Download MS Word (42 KB)Acknowledgments
Dr Wang Jinxiang at South China Agricultural University is thanked for providing A. rhizogenes strain K599.
Disclosure statement
No potential conflict of interest was reported by the authors.
Additional information
Funding
References
- Wan XR , Li L . Regulation of ABA level and water-stress tolerance of Arabidopsis by ectopic expression of a peanut 9-cisepoxycarotenoid dioxygenase gene. Biochem Bioph Res Co. 2006;347(4):1030–1038.
- Huang L , Jiang HF , Ren XP , et al. Abundant microsatellite diversity and oil content in wild Arachis species. PLoS ONE [Internet]. 2012 [cited 2015 Jun 10];7(11):e50002. Available from: http://dx.doi.org/10.1371/journal.pone.0050002.
- Taylor CG , Fuchs B , Collier R , et al. Generation of composite plants using Agrobacterium rhizogenes . Methods Mol Biol. 2006;343:155–167.
- Somers DA , Samac DA , Olhoft PM . Recent advances in legume transformation. Plant Physiol. 2003;131(3):892–899.
- Estrada-Navarrete G , Alvarado-Affantranger X , Olivares JE , et al. Agrobacterium rhizogenes transformation of the Phaseolus spp.: a tool for functional genomics. Mol Plant Microbe Interact. 2006;19(12):1385–1393.
- Chilton MD , Tepfer DA , Petit A , et al. Agrobacterium rhizogenes inserts T-DNA into the genomes of the host plant root cells. Nature . 1982;295:432–434.
- Christey MC . Use of Ri mediated transformation for production of transgenic plants. In Vitro Cell Dev Biol Plant. 2001;37:687–700.
- Collier R , Fuchs B , Walter N , et al. Ex vitro composite plants: an inexpensive, rapid method for root biology. Plant J. 2005;43(3):449–457.
- Chabaud M , Boisson-Dernier A , Zhang J , et al. Agrobacterium rhizogenes-mediated root transformation. In: Mathesius U , Journet EP , Sumner LW , editors. Medicago truncatula handbook. 1st ed. Vol 1. Ardmore ( OK) : Samuel Roberts Noble Foundation; 2006.
- Kereszt A , Li D , Indrasumunar A , et al. Agrobacterium rhizogenes-mediated transformation of soybean to study root biology. Nat Protoc. 2007;2:948–952.
- Cho HJ , Farrand SK , Noel GR . High-efficiency induction of soybean hairy roots and propagation of the soybean cyst nematode. Planta. 2000;210:195–204.
- Cao D , Hou W , Song S , et al. Assessment of conditions affecting Agrobacterium rhizogenes-mediated transformation of soybean. Plant Cell Tiss Org Cult. 2009;96:45–52.
- Georgiev M , Pavlov A , Bley T . Hairy root type plant in vitro systems as sources of bioactive substances. Appl Microbiol Biot. 2007;74:1175–1185.
- Shi H , Long Y , Sun T , et al. Induction of hairy roots and plant regeneration from the medicinal plant Pogostemon cablin . Cell Tiss Organ Cult. 2011;107:251–260.
- Ishida JK , Yoshida S , Ito M , et al. Agrobacterium rhizogenes-mediated transformation of the parasitic plant Phtheirospermum japonicum . PLoS ONE [Internet]. 2011 [cited 2015 Jun 10]; 6(10):e25802. Available from: http://dx.doi.org/10.1371/journal.pone.0025802.
- Hao YJ , Wei W , Song QX , et al. Soybean NAC transcription factors promote abiotic stress tolerance and lateral root formation in transgenic plants. Plant J. 2011;68:302–313.
- Liu YF , Li QT , Lu X , et al. Soybean GmMYB73 promotes lipid accumulation in transgenic plants. BMC Plant Biol [Internet]. 2014 [cited 2015 Jun 10]; 14:73. Available from: http://dx.doi.org/10.1186/1471-2229-14-73.
- Akasaka Y , Mii M , Daimon H . Morphological alterations and root nodule formation in Agrobacterium rhizogenes-mediated transgenic hairy roots of peanut (Arachis hypogaea L.). Ann Bot. 1998;81:355–362.
- Kim JS , Lee SY , Park SU . Resveratrol production in hairy root culture of peanut, Arachis hypogaea L. transformed with different Agrobacterium rhizogenes strains. Afr J Biotechnol. 2008;7:3788–3790.
- Tao J , Li L . Genetic transformation of Torenia fournieri L. mediated by Agrobacterium rhizogenes . S Afr J Bot. 2006;72:211–216.
- Barik DP , Mohapatra U , Chand PK . Transgenic grasspea (Lathyrus sativus L.): factors influencing Agrobacterium-mediated transformation and regeneration. Plant Cell Rep. 2005;24(9):523–531.
- Fujita Y , Fujita M , Satoh R , et al. AREB1 is a transcription activator of novel ABRE-dependent ABA signaling that enhances drought stress tolerance in Arabidopsis. Plant Cell. 2005;17:3470–3488.
- Li XY , Liu X , Yao Y , et al. Overexpression of Arachis hypogaea AREB1 gene enhances drought tolerance by modulating ROS scavenging and maintaining endogenous ABA content. Int J Mol Sci. 2013;14:12827–12842.
- Yoshida T , Fujita Y , Sayama H , et al. AREB1, AREB2, and ABF3 are master transcription factors that cooperatively regulate ABRE-dependent ABA signaling involved in drought stress tolerance and require ABA for full activation. Plant J. 2010;61:672–685.
- Li XY , Lu JB , Liu S , et al. Identification of rapidly induced genes in the response of peanut (Arachis hypogaea) to water deficit and abscisic acid. BMC Biotechnol [Internet]. 2014 [cited 2015 Jun 10];14:58. Available from: http://dx.doi.org/10.1186/1472-6750-14-58.
- Su LC , Liu X , Chen YP , et al. Isolation of AhDHNs from Arachis hypogaea L. and evaluation of AhDHNs expression under exogenous abscisic acid (ABA) and water stress. Afr J Biotechnol. 2012;11(51):11221–11229.
- Murashige T , Skoog F . A revised medium for rapid growth and bioassays with tobacco tissue culture. Physiol Plantarum. 1962;15:473–497.
- Sambrook J , Fritsch EF , Maniatis T . Molecular cloning: a laboratory manual, 2nd ed. Cold Spring Harbor ( NY ): Cold Spring Harbor Laboratory; 1989.
- Edward K , Johnstone C , Thompson C . A simple and rapid method for the preparation of plant genomic DNA for PCR analysis. Nucleic Acids Res. 1991;19:1349.
- Livak KJ , Schmittgen TD . Analysis of relative gene expression data using real-time quantitative PCR and the 2-ΔΔCT method. Methods. 2001;25:402–408.
- Ilina EL , Logachov AA , Laplaze L , et al. Composite Cucurbita pepo plants with transgenic roots as a tool to study root development. Ann Bot. 2012;110:479–489.
- Crane C , Wright E , Dixon RA , et al. Transgenic Medicago truncatula plants obtained from Agrobacterium tumefaciens transformed roots and Agrobacterium rhizogenes-transformed hairy roots. Planta. 2006;223(6):1344–1354.
- Orlikowska TK , Cranston HJ , Dyer WE . Factors inflencing Agrobacterium tumefaciens-mediated transformation and regeneration of the safflower cultivar ‘Centennial’. Plant Cell. 1995;40(1):85–91.
- Sreeramanan S , Vinod B , Sashi S , et al. Optimization of the transient Gus a gene transfer of Phalaenopsis Violacea orchid via Agrobacterium tumefaciens: an assessment of factors inflencing the effiency of gene transfer mechanisms. Adv Nat Appl Sci. 2008;2(2):77–88.
- Kumar V , Jones B , Davey MR . Transformation by Agrobacterium rhizogenes and regeneration of transgenic shoots of the wild soybean Glycine argyrea . Plant Cell Rep. 1991;10:135–138.
- Karthikeyan AS , Sarma KS , Veluthambi K . Agrobacterium tumefaciens mediated transformation of Vigna mungo (L.) Hepper . Plant Cell Rep. 1996;15:328–331.
- Price AH , Atherton NM , Hendry GAF . Plants under drought-stress generate activated oxygen . Free Rad Res Comms. 1989;8:61–66.
- Gill SS , Tuteja N . Reactive oxygen species and antioxidant machinery in abiotic stress tolerance in crop plants. Plant Physiol Biochem. 2010;48:909–930.
- Kim JS , Lee SY , Park SU . Resveratrol production in hairy root culture of peanut, Arachis hypogaea L. transformed with different Agrobacterium rhizogenes strains. Afr J Biotechnol. 2008;7:3788–3790.
- Geng LL , Niu LH , Gresshoff PM , et al. Efficient production of Agrobacterium rhizogenes-transformed roots and composite plants in peanut (Arachis hypogaea L.). Plant Cell Tiss Organ Cult. 2012;9:491–500.