ABSTRACT
The genetic diversity and pathogenicity/virulence among 60 eggplant Sclerotinia sclerotiorum isolates collected from six different geographic regions of Turkey were analysed using mycelial compatibility groupings (MCGs), random amplified polymorphic DNA (RAPD) and simple sequence repeat (SSR) polymorphism. By MCG tests, the isolates were classified into 22 groups. Out of 22 MCGs, 36% were represented each by a single isolate. The isolates showed great variability for virulence regardless of MCG and geographic origin. Based on the results of RAPD and SSR analyses, 60 S. sclerotiorum isolates representing 22 MCGs were grouped in 2 and 3 distinct clusters, respectively. Analyses using RAPD and SSR markers illustrated that cluster groupings or genetic distance of S. sclerotiorum populations from eggplant were not distinctly relative to the MCG, geographical origin and virulence diversity. The patterns obtained revealed a high heterogeneity of genetic composition and suggested the occurrence of clonal and sexual reproduction of S. sclerotiorum on eggplant in the areas surveyed.
Introduction
Eggplant (Solanum melongena L.) is an important solanaceous crop for Turkey, which is the largest producer of eggplant in Europe, averaging a production of 826.941 tons and it ranks as one of the most important vegetable crops in the world with an annual worldwide production of 49.418.212 tons. [Citation1]
Sclerotinia sclerotiorum (Lib.) de Barry, cosmopolitan soilborne nectrotrophic plant pathogens, infects more than 400 different plant species worldwide and causes white mold, stem rot and fruit rot diseases.[Citation2] Most of the economically important dicotyledonous plant species are the main hosts of S. sclerotiorum, although several economically important monocotyledonous plant species are also reported as host of S. sclerotiorum.[Citation2] Recent estimates of annual yield losses caused by S. sclerotiorum exceed over several hundred million dollars due to lack of high levels of host resistance and difficulty of management practices. Management of diseases caused by S. sclerotiorum was highly difficult due to widespread distribution, sporadic occurrence and the long-term persistence of sclerotia in soil. Chemical and cultural control measures have been the major strategies for combating the diseases caused by S. sclerotiorum,[Citation3] although none of these management measures effectively prevent the infection process alone.
The amount and distribution of genetic variability of plant pathogen populations affect the efficacy of control strategies of plant diseases. Therefore, the studies on the genetic structure of pathogen populations should be an important step when planning management programmes aimed to prevent or control the disease. Differentiation of S. sclerotiorum strains based on morphological differences in sclerotia, mycelial growth and ascospores has been reported in previous studies.[Citation4,Citation5]
In Turkey, during the last few years, many Sclerotinia isolates have been recovered from host plants such as sunflower, sweet basil, rape seed, tomato, potato and melon,[Citation6,Citation7] and identified based on cultural and morphological characteristics. However, as the Sclerotinia collection grew, great variability in these characteristics was noted among isolates belonging to the same Sclerotinia sp. The presence of different physiological, ecological, biochemical, pathogenic and molecular variations within Sclerotinia isolates has also been reported worldwide.[Citation8–10]
Two different methods, mycelial compatibility groups (MCGs) and molecular markers, were used to determine genetic variation among populations of S. sclerotiorum.[Citation4] Mycelial compatibility (MC) is the ability of two fungal strains hyphae to anastomose and form one integrated colony in filamentous fungi.[Citation4] MCGs tend to be genetically isolated from each other.[Citation4] A number of molecular techniques have been used to investigate genetic diversity of S. sclerotiorum including random amplified polymorphic DNA (RAPD),[Citation11] inter simple sequence repeat (ISSR) [Citation12] and simple sequence repeat (SSR).[Citation13] Previous studies indicated that populations of S. sclerotiorum were genetically diverse and had predominantly several clonal components with occasional recombination within populations, as well as contemporary population diversification at a local scale.[Citation6,Citation8,Citation13,Citation14]
MCG diversity, genetic variability and pathogenicity relationships of S. sclerotiorum populations infesting eggplant production areas have not been extensively reported yet. It is important to understand the epidemiology and genetic diversity of S. sclerotiorum to control plant diseases by chemical and cultural methods. Additional insight into Sclerotinia problems might be achieved via molecular characterization of local isolates. The purpose of the current study was to investigate the genetic structure within the eggplant host populations of S. sclerotiorum in southern provinces of Turkey based on molecular and morphological markers.
Materials and methods
Sclerotia of S. sclerotiorum were obtained from stem rot infested plants from commercial eggplant fields and greenhouses in Turkey during 2012, surface-sterilized using 70% ethanol for 1 min, washed three times in sterile distilled water, plated on potato dextrose agar (PDA) (Difco Laboratories, Detroit) containing 75 μL L−1 tetracycline and incubated for 72 h at room temperature for three days. A total of 60 isolates were collected on single infected eggplant plants grown in Adana, Antalya, Hatay, Kahramanmaraş and Osmaniye provinces (). The isolates were stored in a collection maintained at Mustafa Kemal University, Plant Protection Department.
Table 1. Sclerotinia sclerotiorum isolates used in this study with indication of geographic origin (province and location), mycelial compatibility grouping (MCG), virulence (lesion length) and pattern of random amplified polymorphic DNA (RAPD) and haplotype of single strand polymorphism.
MC grouping was determined as described by Schafer and Kohn.[Citation15] A 6 mm plug was taken from each isolate, 1 cm inside the growing edge of the colony, and placed in PDA (9 cm Petri plate) amended with 75 μL L−1 of McCormick's red food colouring. Each isolate was purified by transferring the single hyphal tip onto the fresh medium, and generated sclerotia were stored at −20 °C until use.[Citation8]
Each isolate was paired with itself as a control for compatibility. Pairing plates were incubated in 12/12 h light/dark cycle at room temperature. The two sets of isolates were paired in all possible combinations. MC and incompatibility reactions were recorded 7 and 14 days after inoculation. Each experiment was repeated at least twice. Pairings were scored as mycelially compatible when no reaction line was observed within the interaction zone between paired isolates. Pairings were scored as mycelially incompatible when a thin to wide band of uniform, aerial mycelium or thin red line on the colony surface, reverse or both was observed between adjacent paired isolates.[Citation4]
All 60 S. sclerotiorum isolates were tested for pathogenicity assays on stems of eggplant cultivar Halep Karası. The plants were grown in potting mix in containers for three weeks in a greenhouse under natural light. A cut-stem method of inoculation [Citation14] was employed. The wide ends of 1000 μL sterile pipette tips were pressed into the leading edge of mycelium of four- to five-days-old cultures grown on PDA until having 12 mm length of agar inside the pipette tip. Then, the pipette tips were placed over the ends of freshly cut stems until the cut ends of stems were inserted into the agar. The inoculated eggplants were kept under humid conditions (≥90% relative humidity (RH)) for 36 h, and then transferred in a growth room at 26 ± 2 °C under fluorescent lights for a 12 h day−1. Lesion length was recorded four days after inoculation. Pipette tips containing PDA having no inoculum were placed on the control plants.
DNA manipulation
Single sclerotium isolates were incubated at 28 °C for three days on Petri dishes containing solid PDA medium. Single 3 mm mycelial plugs excised from the margin of the colonies grown on PDA were introduced into conical flasks containing 50 mL potato dextrose broth and were maintained at 28 °C in a shaker at 120 rpm for 10 days. Mycelium was collected by filtration, washed with saline and kept in liquid nitrogen until use. DNA extraction was performed according to the protocol described by Zolan and Pukkila.[Citation16] DNA quantification was determined by spectrophotometry and visualization under ultraviolet light after electrophoresis on 0.8% agarose gels prepared with 1× TBE (Tris-borate-ethylenediaminetetraacetic acid (EDTA)) and staining with ethidium bromide (0.5 μg/mL in water).[Citation17]
RAPD analysis
Purified DNA was amplified with 10 different 10-mer oligonucleotide RAPD primers; OPA-01, OPA-05, OPA-16, OPB-01, OPB-06, OPB-114, OPB-119, OPC-02, OPC-08 and OPC-15 (). The RAPD reactions were performed in a final volume of 25 μL reaction mixture containing 16.0 μL milliq-water (MQ-water), 2 μL (50 ng) template DNA, 1.5 μL deoxyribonucleotide triphosphates (dNTPs), 1.5 μL MgCl2, 2.5 μL 10× buffer, 0.5 μL (0.5 U) Taq DNA polymerase and 1 μL primer. All of the reagents were obtained from Fermentas Inc., USA. Amplification was performed in a programmable thermo cycler (Eppendorf Mastercycler) with the following profile: one cycle at 95 °C for 5 min; 35 cycles at 94 °C for 1 min, 45 °C for 1 min and 72 °C for 2 min; one cycle at 72 °C for 5 min. Amplification was performed in programmable thermo cycler (Eppendorf Mastercycler) with amplification profile. The amplified fragments were electrophoresed on 1.8% (w/v) agarose gel with ethidium bromide (EtBr) stain (0.1 μg μL−1), for 3 h at 80 V in 1× TBE buffer (89 mmol/L Tris, 89 mmol/L boric acid and 2 mmol/L EDTA). Gel was visualized under UV light with Gel Documentation System (Vilbert, Lourmat, Marne La Vallee, France).
Table 2. List of RAPD primers, observed band numbers and polymorphic band numbers.
SSR analysis
For the SSR reactions, six primer pairs were used. Polymerase chain reaction (PCR) amplifications were performed in 25 μL reaction mixtures solution containing 2.5 μL of 10× standard buffer (15 mmol/L of MgCl2), 0.25 μ mol/L each SSR primer (), 250 μ mol/L each dNTP (Promega), 1.25 U of Taq DNA polymerase, 0.20 μL of stabilizer (1% W-1 (v/v), Life Technologies), ddH2O and 25 ng template DNA. Amplification was performed in a programmable thermo cycler (Eppendorf Mastercycler) with the following profile: initial denaturation at 95 °C for 8 min, followed by 35 cycles primer annealing at 59 °C (for all microsatellite primers) and extension at 72 °C for 60 s, with a 5 min extension at 72 °C on the final cycle. The SSR amplification products were separated in a vertical denaturing 10% polyacrylamide gel by electrophoresis at 200 V in TBE buffer (500 mmol/L EDTA pH 8.0, 1 mol/L Tris pH 8.0, 89 mmol/L boric acid) for 2 h. After that, the gel was silver nitrate-stained (0.2%) and visualized under UV light with Gel Documentation System (Vilbert, Lourmat, Marne La Vallee, France).
Table 3. List of SSR primers, observed band numbers and polymorphic band numbers.
Statistical analysis
Banding patterns were scored as 1 when present or 0 when absent. DNA fragments of the same size, produced by a single primer, were considered to be identical. Each isolate was identified regarding the presence (1) or absence (0) of the fragment amplified in each isolate for each primer. Similarity matrices were obtained by the un-weighted pair group method with arithmetic mean (UPGMA) using the NTSYSpc2 software, Version 2.1.[Citation18] The pathogenicity data were analysed by one-way analysis of variance using PROC GLM in SAS. Isolate was the treatment and lesion length was the observational units.
Results and discussion
For plant-pathogenic fungi, MCG, a phenotypic marker system controlled by multiple loci, is a useful method to study the distribution of isolates in geographic areas for a fungal species.[Citation19] During the surveys, 60 isolates each from a different commercial field and unique sclerotium were recovered from Adana (3 locations, 13 isolates), Antalya (3 locations, 17 isolates), Hatay (4 locations, 12 isolates), Kahramanmaraş (2 locations, 5 isolates), Mersin (4 locations, 8 isolates) and Osmaniye (1 location, 5 isolates).
S. sclerotiorum isolates obtained from 6 provinces in 16 locations were compared with each other and with isolates in the other localities and 22 MCGs were obtained (). MCGs 1, 2, 3, 4 and 5 originated exclusively from Adana province. MCG 1 occurred only in Adana-Ceyhan location, and MCGs 4 and 5 were found in Adana-Kozan, while Adana-Center had mostly MCG 2 and rarely MCG 3. In Antalya province, 7 different MCGs, from 6 to 12 were identified. MCGs 6 and 7 were isolated merely from Demre, MCGs 11 and 12 were isolated from Manavgat, MCGs 8, 9 and 10 from Kumluca and MCG 11 from Antalya-Center. Three MCGs determined in Hatay province were MCGs 13, 14 and 15. The MCG 13 was unique in Kırıkhan. The MCG 14 was found from Hatay-Center and Reyhanlı, while MCG 15 was isolated from Samandağ. MCG 17 was the only MCG at all locations of Kahramanmaraş. MCGs 18, 19, 20 and 21 isolated from Mersin province at Erdemli, Mersin-Center and Silifke locations. MCGs 18 and 21 were unique to Erdemli-Mersin and Tarsus-Mersin, respectively. MCG 20 was found in Tarsus and Silifke. However, the most prevalent group was MCG 19 found in Mersin-Center and Mersin-Silifke province. No MCG diversity was observed among isolates from Osmaniye and Kahramanmaraş. MCGs 17 and 22 were unique to all locations of Kahramanmaraş and Osmaniye, respectively.
Isolates of S. sclerotiorum from eggplant fields of Adana and Antalya were heterogeneous mix of MCGs. Consequently, the population of S. sclerotiorum isolates affecting eggplants of Adana and Antalya is more diverse than the population affecting eggplants grown in Mersin, Hatay, Osmaniye and Kahramanmaraş. This verifies the previous reports of S. sclerotiorum MCG population structures on cucumber,[Citation20] sunflower,[Citation5] soybean [Citation21] and canola.[Citation22] In the present study, some of the isolates collected from the same or rather close location of the same provinces were grouped into two different MCGs, suggesting the existence of a heterogeneous mix of MCGs in that location. Variation in environmental conditions, selection pressure due to introduction of resistant eggplant cultivars can favour the increase of certain MCGs, while recombination and mutations can lead to new MCGs in S. sclerotiorum contaminated eggplant fields. In addition, new isolates can be introduced by human activities and contaminated plant materials. The distribution of S. sclerotiorum in Adana, Antalya, Mersin, Hatay, Osmaniye and Kahramanmaraş suggests that almost all of the MCGs are more specific for certain locations, showing very limited movement between locations.
To determine pathogenicity/virulence of 60 isolates, lesion lengths were measured. All tested isolates were pathogenic and initial lesion formation was visible 24 h after inoculation. Lesion length of isolates significantly varied between 20 and 102 mm. The lowest and the highest lesion lengths were obtained from Scl59 in Osmaniye-Center and Scl18 in Antalya-Demre locations, respectively (). Significantly different lesion lengths were obtained in the same MCGs and in the same locations. In terms of virulence, there were significant differences among isolates and groups in all localities. Highly virulent or weakly virulent isolates were found in the same group.
The results of the pathogenicity test showed that all of the tested 60 isolates were pathogenic to eggplant. However, significant differences in virulence were observed within the same location, similar to other reports.[Citation5,Citation10] Isolates Scl11, Scl18, Scl36, Scl58 and Scl44 from Adana, Antalya, Hatay, Osmaniye and Kahramanmaraş, respectively, were consistently the most virulent isolates tested on eggplant. While the isolates Scl59 (Osmaniye), Scl51 and Scl52 (Mersin), Scl40 (Hatay) and Scl22 (Antalya) were the least virulent isolates. In the current study, significant differences in virulence were observed among same MCGs, regardless of the isolates locations. Therefore, MCG did not seem to be a suitable marker for virulence since the variations in virulence of isolates associated with neither MCG, nor geographic origin belongings. Highly virulent or weakly virulent isolates of S. sclerotiorum were obtained in the same group and also among the groups. Our findings agree with the previous studies [Citation20,Citation23] in which virulence levels of the isolates were different within and among the MCGs. Results of this study are in line with other published studies on S. sclerotiorum, with examples of different levels of MCG diversity in a given geographical area.[Citation11] Significant differences in aggressiveness among MCGs were also reported by Li et al.[Citation5] However, no variation in aggressiveness among isolates from different geographical areas has been noted in a number of studies.[Citation8,Citation24]
S. sclerotiorum isolates evaluated in the current study were characterized using 12 random primers of the OPC and OPE series. The data set of DNA markers obtained from all the isolates with RAPD markers was analysed with UPGMA by the NTSYS 2.0 software, and a dendogram (Jaccard's coefficient) was constructed (). Based on the results of RAPD analysis, 60 S. sclerotiorum isolates representing 22 MCGs were grouped in two distinct clusters at 89.2% of distance (). Group I consisted of 35 isolates with 93%–95% genetic similarity, whereas, Group II comprised of 25 isolates in distinct locations, and exhibited genetic similarity of 89%–94%. At 93% of genetic similarity coefficient, Group I was divided into four subgroups with 28 isolates in subgroup IA, 4 isolates in subgroup IB, 3 isolates in subgroup IC, 3 isolates in subgroup ID, while Group II was divided into five subgroups, with 3 isolates in subgroup IIA, 13 isolates in subgroup IIB, 2 isolates in subgroup IIC, 4 isolates in subgroup IID and 3 isolates in subgroup IIE at 95% similarity coefficient. There were no strong associations between RAPD clustering and MCG profiles.
Figure 1. Un-weighted pair group method with arithmetic mean (UPGMA) dendrograms showing the genetic relationships between the 60 isolates of Sclerotinia sclerotiorum based on RAPD markers.
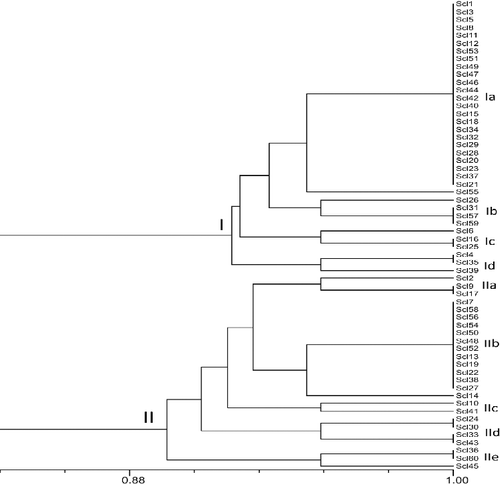
The data set of DNA markers obtained from all the isolates with SSR markers was analysed with UPGMA by the NTSYS 2.0 software, and a dendogram (Jaccard's coefficient) was constructed (). All the isolates were divided into three major groups (). Group I consisted of 15 isolates with 89% genetic similarity. Group II comprised of 25 isolates in distinct locations, exhibited genetic similarity of 92%, whereas, Group III comprised of 20 isolates, and had genetic similarity of 91%. At 95% of genetic similarity coefficient, Group I was divided into four subgroups with 3 isolates in subgroup IA, 2 isolates in subgroup IB, 4 isolates in subgroup IC and 4 isolates in subgroup ID. Group II was divided into four subgroups with 5 isolates in subgroup IIA, 12 isolates in subgroup IIB, 3 isolates in subgroup IIC and 5 isolates in subgroup IID, and Group III was divided into four subgroups with 4 isolates in subgroup IIIA, 11 isolates in subgroup IIIB, 3 isolates in subgroup IIIB and 2 isolates in subgroup IIID at 95% similarity coefficient. There were no strong associations between SSR clustering and MCG profiles.
Figure 2. Un-weighted pair group method with arithmetic mean (UPGMA) dendrograms showing the genetic relationships between the 60 isolates of Sclerotinia sclerotiorum based on SSR markers.
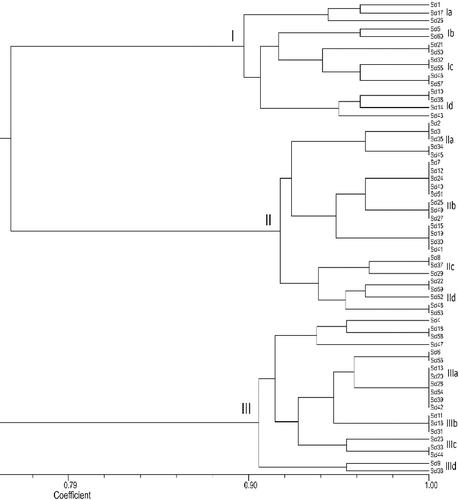
There was no obvious clustering according to geographic location for MCGs. However, MCGs containing isolates from more than one location clustered separately from groups containing isolates from only one location. Previous studies showed that MCG diversity can vary greatly on a specific host in a given geographical area.[Citation25] The present study confirmed this conclusion, for instance, 17 isolates collected from eggplant fields in Antalya were separated into seven MCGs. Most of the isolates collected from the same province could be grouped together, such as MCG 17 in which the tested isolates were collected from Kahramanmaraş province, MCG 22 mainly contains isolates collected from Osmaniye; also, majority of isolates from Adana province was grouped into MCG 11 and MCG 12 (). Conversely, some of S. sclerotiorum isolates, collected from the same provinces such as Antalya (17 isolates), were grouped into seven different MCGs. The presence of unique MCGs in some of the locations is the evidence of new MCGs emergence and evolution of new genotypes.
The analysis of population dynamics of S. sclerotiorum is an essential part of understanding how the underlying mechanisms are involved in pathogen distribution across different geographic regions. Based on molecular characterization of S. sclerotiorum isolates, it is evident that the isolates from eggplants in Adana, Antalya, Mersin, Hatay, Osmaniye and Kahramanmaraş provinces had a high level of diversity and were grouped in two and three clusters determined by the RAPD and SSR markers, respectively. When clusters of RAPD and SSR were compared with those of MC, there was no strong association between the genetic distance for isolates collected from the different locations and provinces ( and ). The distribution of the isolates indicated no sign of tendency toward the formation of clusters with respect to province, showing that occurrence of specific population distribution restricted to a specific location. Several studies have demonstrated that isolates from the same MCG are genetically more similar than isolates from different MCGs.[Citation4,Citation26,Citation27] Previous studies showed that MCGs or SSR markers were not associated with specific virulence characteristics or ecological adaptations of the pathogen.[Citation8,Citation9,Citation14,Citation25] However, in other studies, a close association between MCG and cluster grouping was reported [Citation5,Citation21]; SSR markers were found to be more efficient in detecting genetic diversity among the isolates than RAPD. SSR markers exhibited the best polymorphic DNA banding pattern.
Conclusions
The results from this study demonstrate that MCG variations in S. sclerotiorum occur within the populations of provinces, as well as populations collected from the same location of each province. The difference in the distribution of MCG among locations and provinces was notable. Pathogen virulence had neither relationship with clusters of RAPD and SSR nor with MCGs. The populations of S. sclerotiorum were genetically heterogeneous and that combination of MCG and molecular analysis did not offer a powerful tool for understanding this heterogeneity. Human activities, movement of contaminated plant materials and soil debris might tend to increase the diversity of MCGs while outbreeding would raise genetic diversity through meiotic recombination that causes to increase MCG numbers. The potential sexual recombination between MCGs indicates the possible rise of diverse genotypes in each province. Therefore, preventive control methods combined with agricultural practices are needed to restrict gene flow between populations and provinces.
Disclosure statement
No potential conflict of interest was reported by the authors.
Additional information
Funding
References
- FAOSTAT. Food and Agricultural Organization of the United Nations. 2015. [cited 2015 Dec 15]. Available from: http://faostat3.fao.org/browse/Q/QC/E
- Boland GJ, Hall R. Index of plant hosts of Sclerotinia sclerotiorum. Can J Plant Pathol. 1994;16:93–100.
- Rousseau G, Rioux S, Dostaler D. Effect of crop rotation and soil amendments on Sclerotinia stem rot on soybean in two soils. Can J Plant Sci. 2007;87:605–614.
- Kohn L, Stasovski E, Carbone I, et al. Mycelial incompatibility and molecular markers identify genetic variability in field populations of Sclerotinia sclerotiorum. Phytopathology. 1991;81:480–485.
- Li Z, Zhan M, Wang Y, et al. Mycelial compatibility group and pathogenicity variation of Sclerotinia sclerotiorum populations in sunflower from China, Canada and England. Plant Pathol J. 2008;2:131–139.
- Mert-Türk F, Ipek M, Mermer D, et al. Microsatellite and morphological markers reveal genetic variation within a population of Sclerotinia sclerotiorum from oilseed rape in the Çanakkale province of Turkey. J Phytopathol. 2007;155:182–187.
- Tok FM. First report of white mold caused by Sclerotinia sclerotiorum on sweet basil in Turkey. Plant Dis. 2008;92:1471.
- Atallah ZK, Larget B, Chen X, et al. High genetic diversity, phenotypic uniformity, and evidence of outcrossing in Sclerotinia sclerotiorum in the Columbia basin of Washington state. Phytopathology. 2004;94:737–742.
- Sexton AC, Howlett BJ. Microsatellite markers reveal genetic differentiation among populations of Sclerotinia sclerotiorum from Australian fields. Curr Genet. 2004;46:357–365.
- Irani H, Heydari A, Javan-Nikkhah M, et al. Pathogenicity variation and mycelial compatibility groups in Sclerotinia sclerotiorum. J Plant Prot Res. 2011;51:329–336.
- Sun JM, Irzykowski W, Jedryczka M, et al. Analysis of the genetic structure of Sclerotinia sclerotiorum (Lib.) de Bary populations from different regions and host plants by random amplified polymorphic DNA markers. J Integr Plant Biol. 2005;47:385–395.
- Arbaoui M, Kraic J, Huszar J. Genetic variation of Sclerotinia sclerotiorum isolates from different conditions. Agriculture (Poľnohospodárstvo). 2008;54:36–39.
- Sexton AC, Whitten AR, Hawlett BJ. Population structure of Sclerotinia sclerotiorum in an Australian canola field at flowering and stem-infected stages of the disease cycle. Genome. 2006;49:1408–1415.
- Kull LS, Vuong TD, Powers KS, et al. Evaluation of resistance screening methods for Sclerotinia stem rot of soybean and dry bean. Plant Dis. 2003;87:1471–1476.
- Schafer MR, Kohn LM. An optimized method for mycelial compatibility testing in Sclerotinia sclerotiorum. Mycologia. 2006;98(4):593–597.
- Zolan ME, Pukkila PJ. Inheritance of DNA methylation in Coprinus cinereus. Mol Cell Biol. 1986;6:195–200.
- Sambrook J, Fritsch EF, Maniatis T. Molecular cloning: a laboratory manual. Vol. 3. Cold Spring Harbor (NY): Cold Spring Harbor Laboratory Press; 1989. p. 1626.
- Rohlf FJ. NTSYS-pc: numerical taxonomy and multivariate analysis system. Version 2.1. Setauket (NY): Exeter Biological Software; 2000.
- Anderson JB, Kohn LM. Clonality in soilborne, plant-pathogenic fungi. Annu Rev Phytopathol. 1995;33:369–391.
- Onaran A, Yanar Y. Distribution, pathogenicity and mycelial compatibility groups of Sclerotinia sclerotiorum (Lib.) De Bary, causal agent of white mold disease of cucumber, in greenhouses in the vicinity of Tokat and Amasya. (TABAD) Res J Agric Sci. 2009;1:63–68.
- Hambleton S, Walker C, Kohn LM. Clonal lineages of Sclerotinia sclerotiorum previously known from other crops predominate in 1999–2000 samples from Ontario and Quebec soybean. Can J Plant Pathol. 2002;24:309–315.
- Kohli Y, Morrall RAA, Anderson JB, et al. Local and trans-Canadian clonal distribution of Sclerotinia sclerotiorum on canola. Phytopathology. 1992;82:875–880.
- Auclair J, Boland GJ, Kohn LM, et al. Genetic interactions between Glycine max and Sclerotinia sclerotiorum using a straw inoculation method. Plant Dis. 2004;88:891–895.
- Xie C, Huang CH, Vallad GE. Mycelial compatibility and pathogenic diversity among Sclerotium rolfsii isolates in the southern United States. Plant Dis. 2014;98:1685–1694.
- Yanar Y, Onaran A. Mycelial compatibility groups and pathogenicity of Sclerotinia sclerotiorum (Lib.) De Bary causal agent of white mold disease of greenhouse grown cucumber in Antalya-Turkey. Afr J Biotechnol. 2011;10:3739–3746.
- Leslie JF. Fungal vegetative compatibility. Annu Rev Phytopathol. 1993;31:127–151.
- Meinhardt LW, Wulff NA, Bellato CM, et al. Telomere and microsatellite primers reveal diversity among Sclerotinia sclerotiorum isolates from Brazil. Fitopatol Bras. 2002;27:211–215.