ABSTRACT
Ribulose-1,5-bisphosphate carboxylase/oxygenase (Rubisco) is a key enzyme involved in CO2 assimilation during photosynthesis. Rubisco activation depends on the activity of Rubisco activase (RCA). We performed 3′/5′ rapid amplification of cDNA ends (RACE) and reverse transcription polymerase chain reaction (RT-PCR) to amplify the 3′ and 5′ end sequences of RCA genes from hickory. We obtained two full-length gene sequences, designated CcRCAα and CcRCAβ. The two corresponding cDNAs are divergent in both the translated and 3′ untranslated regions. The analysis of the genomic DNA sequences suggested that the corresponding mRNAs are transcripts of two different genes and not the products of a single alternatively spliced pre-mRNA. We examined the expression of CcRCAα and CcRCAβ in hickory leaves at various stages of development by quantitative real-time PCR (qRT-PCR) analysis. The results suggest that RCA genes play an important role in development and environmental responses. These results provide a basis for modulating RCA gene expression to improve the photosynthetic rate and plant growth in hickory.
Introduction
Photosynthesis is one of the most important biochemical reactions in nature. CO2 fixation, a key step in this process, requires catalysis by the enzyme ribulose-1,5-bisphosphate carboxylase/oxygenase (Rubisco) in higher plants.[Citation1] Rubisco enzyme activity is regulated by complex mechanisms. Numerous studies have demonstrated that Rubisco activase (RCA) activates and maintains Rubisco catalytic activity.[Citation2] RCA is also considered to be a key regulator of photosynthesis under various environmental stress conditions.[Citation3] Plants expressing low levels of RCA activity exhibit reduced accumulation of nitrogen and total biomass.[Citation4] Plants with little or no RCA expression are unable to survive standard atmospheric CO2 concentrations.[Citation5] Therefore, it would be important to investigate how to increase CO2 assimilation by modulating RCA expression, which would ultimately affect crop yields.
RCA is an AAA+ (ATPases associated with a variety of cellular activities) family protein that catalyses the activation of Rubisco and participates in the formation of macromolecular complexes;[2] this activation is adenosine triphosphate (ATP)-dependent and is inhibited by adenosine diphosphate (ADP).[Citation6] Portis et al. [Citation7] proposed a model of the mode of action of RCA based on numerous mutagenesis studies of RCA and Rubisco: first, RCA is bound to Rubisco through electrostatic and other forces; second, ATP hydrolysis promotes the movement of the C-terminal domain of Rubisco, causing the N-terminal domain of this protein to also move, which breaks the interactions between the N-terminal domain of Rubisco and the bound sugar phosphate; finally, RCA induces activation of Rubisco by freeing the active sites of this enzyme. Activated Rubisco catalyses the carboxylation of ribulose-1,5-bisphosphate (RuBP) to form two molecules of 3-phosphoglycerate under ample concentrations of CO2. Most plants studied to date contain two forms of RCA, i.e. the α-isoform and β-isoform, which differ only at their C termini. Unlike the β-isoform, the α-isoform harbours a C-terminal extension that contains two redox-sensitive Cys residues.[Citation8] Both the α- and β-isoforms are capable of activating Rubisco independently, but with slightly different maximal activities.[Citation9] Amino-acid sequencing analysis has shown that α-isoforms in different plant species share an average of 74.8% similarity, while that of the β-isoform is 65.9% and antibodies of these isoforms cross-react.[Citation10] Genomic analyses have revealed the presence of only one RCA gene in spinach,[Citation11] Arabidopsis,[Citation11] rice [Citation12] and wheat,[Citation13] two RCA genes in barley [Citation14] and cotton [Citation8] and more than three RCA genes in tobacco [Citation15] and soybean.[Citation16]
Hickory (Carya cathayensis Sarg.) is an important tree used to produce nuts and wood products. Hickory is susceptible to natural stress factors during production, especially high-temperature stress.[Citation17] In this study, we isolated two full-length mRNA sequences of hickory RCA genes and performed sequence analysis and expression pattern analysis of these genes. The expression patterns in the leaves at different developmental stages were analysed by quantitative real-time polymerase chain reaction (qRT-PCR) so as to determine the different gene expression pattern of the two RCA genes. The results of this study lay the foundation for regulating RCA gene expression to increase both the rate of photosynthesis and stress resistance in hickory.
Materials and methods
Plant materials
Hickory plants were grown naturally with normal irrigation and fertilization at the hickory breeding base of Zhejiang A & F University, Zhejiang, China. Hickory leaves were harvested (every seven days from 1 April 2015 to 20 May 2015). They were immediately frozen in liquid nitrogen and stored at −80 °C. Each seven-day period was considered to represent a single stage.
Sequence analysis and primer design
Homologous cloning and transcriptome analysis were conducted to obtain hickory RCA genes. Open-reading frame (ORF) sequences of the β-isoform RCA were used as a query to find similar sequences in the hickory transcriptome. Based on sequence information, gene-specific primers were designed to amplify cDNA by reverse transcription PCR (RT-PCR) and genome DNA fragments and for quantitative analysis using Primer Premier 5.0 software and Primer 3.0 online (http://bioinfo.ut.ee/primer3-0.4.0/), after which two RCA full-length cDNA sequences were cloned from leaves of hichory.
Rapid amplification of cDNA ends (RACE)
Total RNA extraction from leaf samples and first-strand cDNA synthesis were performed as recommended by the manufacturer (TaKaRa Bio, Dalian, China). Specific primer pairs were used to amplify partial gene segments, and the β-actin gene was used as a reference gene (). Information about RCA homologous genes was obtained by searching the National Center for Biotechnology Information (NCBI) database (http://www.ncbi.nlm.nih.gov/). Primers were designed for CcRCA amplification based on the conserved sequences and transcriptome sequences.
Table 1. Primers used to amplify hickory RCA genes and the actin gene.
Both 5′ and 3′ RACE were performed using a SMART™ RACE cDNA Amplification Kit (CLONTECH, Mountain View, CA, USA). Gene-specific primers (GSPs) were designed based on partial hickory RCA gene sequences (). RACE PCR were carried out in T100 Thermal Cycler (BIO-RAD, Hercules, CA, USA) by heating the reaction mixture as follows: 5 cycles of 94 °C for 30 s, 72 °C for 3 min; 5 cycles of 94 °C for 30 s, 70 °C for 30 s and 72 °C for 3 min, then 25 cycles of 94 °C for 30 s, 68 °C for 30 s and 72 °C for 3 min. The RACE PCR products were then cloned into the pMD18-T vector (TaKaRa Bio, Dalian, China) [Citation18] and sent to Sangon Biotech Company (Shanghai) for sequencing.
RCA gene sequence analysis
The cetyltrimethylammonium bromide (CTAB) method was used for the extraction of genomic DNA from leaves. The extracted DNA was then digested with RNase (TaKaRa Bio, Dalian, China) and EcoRI (TaKaRa Bio, Dalian, China) and used as a template to amplify the RCA gene sequences. PCR amplification of the RCA gene sequences was carried out by heating the reaction mixture as follows: 94 °C for 3 min, followed by 32 cycles of 94 °C for 30 s, 61 °C for 30 s and 72 °C for 1.5 min, and finally 94 °C for 5 min to terminate the reaction. Multiple sequence analysis of all sequences was confirmed using DNAMAN V6 software.[Citation18]
Bioinformatics analysis
The ProtParam tool of ExPASy (http://web.expasy.org/protparam/) was applied to calculate physical chemistry characteristics of the encoded RCA gene products, including relative molecular weight, amino acid sequence and hydrophilicity. The PsiPred v3.3 program (http://bioinf.cs.ucl.ac.uk/psipred/) was used for prediction of the secondary structures of the proteins. The ChloroP 1.1 Server (http://www.cbs.dtu.dk/services/ChloroP/) was used for prediction of the chloroplast transit peptides (cTP) of proteins. The subcellular localization of proteins was predicted using the SLP-Local program (http://sunflower.kuicr.kyoto-u.ac.jp/∼smatsuda/slplocal.html). Homologous protein sequence alignment was carried out with ClustalX2.1 software. Multiple amino acid sequence alignments were performed with the nucleotide basic local alignment search tool (BLASTn) in GenBank (http//:www.ncbi.cn) and phylogenetic analysis were performed with MEGA 5.05 software based on the neighbour-joining (NJ) method with 1000 bootstraps.[Citation19]
qRT-PCR for RCA expression profile analysis
qRT-PCR was performed to evaluate the mRNA levels of RCA genes using the BIO-RAD CFX96 System (Bio-Rad, Hercules, CA, USA). A 10-µL reaction mixture was used for PCR containing 5 µL SYBR® Green real-time PCR Master Mix (TaKaRa Bio, Dalian, China), 40 ng cDNA template and 500 nmol/L of each primer (). The expression levels of genes were quantified relative to the expression of the reference gene, β-actin, using the 2−ΔΔCt method.
Statistical methods
Statistical analysis was performed with SPSS 10.0 software (SPSS, Chicago, IL, USA), using Student's t-test to compare means with the significance level at p < 0.05.
Results and discussion
Cloning and sequence analysis of the CcRCA gene
Two full-length cDNAs of RCA-like genes were cloned from the leaves of hickory by RT-PCR and RACE; these genes were designated as CcRCAα and CcRCAβ. The full-length CcRCAα and CcRCAβ are similar in size, but both the 3′ translated regions and 3′ untranslated regions of these genes differ. The cDNAs of CcRCAα and CcRCAβ contained a complete ORF and partial 3′-untranslated sequences. The predicted proteins encoded by CcRCAα and CcRCAβ contain 472 and 435 amino acids, with calculated molecular weights of 51.52 and 47.52 kD, respectively. Multiple sequence analysis revealed 85.44% similarity between the amino acid sequences of these proteins. As shown in , the first 55 and 57 amino acids at the N-termini of CcRCAα and CcRCAβ were predicted to be cTPs. Thus, the predicted mature proteins encoded by CcRCAα and CcRCAβ would contain 417 and 378 amino acids, with calculated molecular weights of 46.11 and 42.08 kD, respectively. The deduced protein sequence of CcRCAα contains a 37-amino acid extension at the C-terminus, including two Cys residues that are known to be involved in redox regulation.[Citation7,Citation8] Two conserved ATP-binding domains, GGKGQGKS and LFIND, were identified at amino acid positions 162 to 170 and 222 to 227, respectively, which indicated that both of these genes belong to the ABC-ATPase superfamily.[Citation20] In addition to the 37-amino acid extension at the C-terminus of CcRCAα, the two RCAs were shown to differ in 26 positions, including nine residues in the putative transit peptides. Obvious differences in CcRCAα and CcRCAβ cDNAs were observed, although all untranslated sequences of these genes have not yet been obtained.
The amino-acid sequence alignment of CcRCAα and CcRCAβ showed that the RCA amino acid sequences in hickory share high homology with those of many plants. We constructed phylogenetic trees including RCA sequences from hickory, apple and 22 other plant species using the NJ method. As shown in and , hickory CcRCAα was shown to have a close genetic relationship with the α-isoform RCA genes of Carya illinoensis, Acer rubrum and Malus domestica. And CcRCAβ was shown to be highly homologous to the β-isoform RCA genes of Vitis vinifera, Glycine max and Gossypium hirsutum. However, the phylogenetic relationship to Picea sitchensis and Chlamydomonas reinhardtii was identified to be distant.
Figure 2. Phylogenetic tree of α-isoform RCA amino acid sequences in different plants based on the NJ method with 1000 bootstrap replicates.
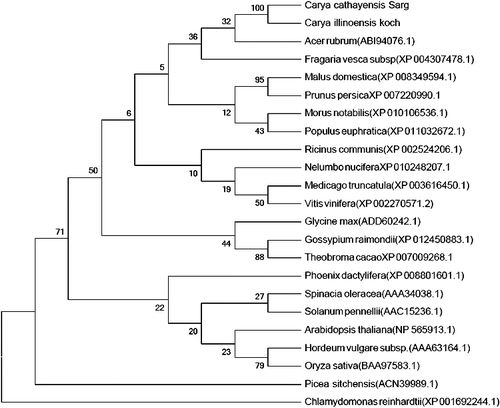
Figure 3. Phylogenetic tree of β-isoform RCA amino acid sequences in different plants based on the NJ method with 1000 bootstrap replicates.
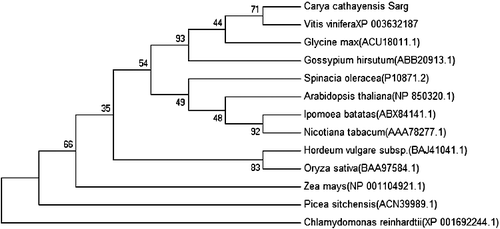
No signal peptides were found in the two putative proteins, using SignalP 4.1, indicating that these proteins are not secretory proteins. The cTP prediction revealed that the two proteins contain cTPs 55 and 57 amino acids in length, respectively. We predicted the transmembrane domains of the proteins using the TMpred program, finding two transmembrane helices (TMHs) located at domains 88 to 107 and 143 to 162, respectively. Protein subcellular localization prediction performed by the PSORT program suggested that both proteins are localized to the chloroplast. Based on the above results, the CcRCAs could be considered nucleus-encoded proteins that function in the chloroplast membrane. Bayramov and Guliyev showed that the RCA was located in leaf, green stem and ear elements in Brachypodium distachyon, where photosynthesis takes place.[Citation21]
Comparison of the sequences of CcRCAα and CcRCAβ using DNAMAN software showed that these two sequences are highly similar, sharing 73.23% similarity, which is consistent with previous results [Citation8,Citation14] of RCA mRNAs comparisons. Therefore, we analysed the genomic DNA from hickory to determine whether CcRCAα and CcRCAβ mRNAs arise from a single alternatively spliced gene. Amplification of genomic DNAs of CcRCAα and CcRCAβ using gene-specific primers produced PCR fragments of 3009 bp for CcRCAα and 2125 bp for CcRCAβ. As shown in , CcRCAα was predicted to contain six introns and seven exons, totalling 1590 and 1419 bp in length, respectively, whereas CcRCAβ contains five introns and six exons totalling 817 and 1308 bp in length, respectively. These results demonstrated the presence of two separate genes encoding RCAα− and β-isoforms in hickory.
RCA genes have been found in all species of higher plants examined to date, including spinach, rice and wheat. In most plants, such as rice,[Citation12] Arabidopsis,[Citation22] spinach,[Citation10] meadow fescue and perennial ryegrass,[Citation23] two isoforms of RCA arise from alternative spicing of a single RCA transcript. However, the number of RCA-encoding genes varies depending on the plant species. In cotton [Citation8] and sweet potato,[Citation24] the two RCA isoforms are encoded by two different genes. In barley, there are two genes, one that is alternatively spliced and a second one that encodes only the β-isoform of RCA.[Citation14] In the aquatic plant Sagittaria graminea, the alternative splicing of RCA mRNA resulted in two protein forms which all belong to the β isoform.[Citation25] To our knowledge, this is the first study identifying two separate genes encoding α− and β-isoforms of RCA in woody plants. However, whether hickory contains other RCA-like genes and what the mechanism regulating alternative splicing of the cloned genes is require further study.
CcRCA gene expression during leaf expansion
We investigated the CcRCA gene expression in hickory leaves at different developmental stages, from the activation of leaf buds to the full expansion of leaves (). As shown in , the expression level of CcRCAα was lowest on 1 April, when leaf buds began to become active after the dormant state. The expression level increased significantly with leaf growth. The level reached a maximum on 22 April, when the leaves had stopped expanding and the leaf area was largest. Plants expressing increased levels of RCA exhibit an increase in the net photosynthesis rate and/or growth.[Citation4,Citation26] Consequently, higher RCA expression is needed to activate Rubisco, resulting in enhanced photosynthesis. After leaf expansion was complete, the CcRCAα expression dropped slightly and then remained at a relatively steady level during the subsequent stages (). The expression pattern of CcRCAβ was similar, with expression increasing significantly and reaching a peak on 22 April. However, the relative expression levels of CcRCAβ were lower than those of CcRCAα at these stages. Notably, in subsequent stages, the expression patterns of these genes differed, with CcRCAβ expression peaking on 6 May and even becoming significantly higher than that of CcRCAα on 20 May (). Some plant species, such as Arabidopsis, camelina and spinach, express equal amounts of α-isoform and β-isoform, while others like rice and wheat accumulate much more β-isoform than α-isoform.[Citation27] Recently, Jurczyk et al. [Citation23] showed that, under normal growth conditions, meadow fescue and perennial ryegrass leaves had a higher content of small RCA isoforms than of large ones. Higher RCAα/RCAβ ratio was recently observed in rice leaves with higher photosynthetic capacity and the decrease in photosynthetic rate and Rubisco activation state highly correlated with the decline of the RCAα/RCAβ ratio during leaf ageing.[Citation28] In the present study, these expression patterns are consistent with the expression patterns of RCA genes in tobacco, which vary and are strongly influenced by environmental factors.[Citation29] Indeed, sudden changes in transcript levels of a β-isoform RCA gene occur in Arabidopsis thaliana, which may result from temperature stress.[Citation30] Both RCA isoforms are active in ATP hydrolysis and Rubisco activation,[Citation7,Citation8] but the specific roles of each isoforms remain unclear. Therefore, further studies are necessary to elucidate the specific functions of both isoforms.
Conclusions
In this study, we isolated two full-length mRNA sequences of hickory RCA genes, CcRCAα and CcRCAβ, and performed sequence alignment and phylogenetic analysis of their deduced encoded protein. The results suggested that these mRNAs are not the products of a single alternatively spliced pre-mRNA transcript, but represent two different genes. We also analysed the expression patterns of these genes in leaves at different developmental stages by qRT-PCR. The results suggested that CcRCAα probably plays an important role both in initial photosynthesis and in the development of the photosynthetic apparatus. CcRCAβ may function synergistically with CcRCAα during early leaf development.
Acknowledgment
We are very grateful to the editors and reviewers for their constructive comments on this paper.
Disclosure statement
No potential conflict of interest was reported by the authors.
Additional information
Funding
References
- Spreitzer RJ, Salvucci ME. Rubisco: structure, regulatory interactions, and possibilities for a better enzyme. Annu Rev Plant Biol. 2002;53:449–475.
- Portis AR Jr. Rubisco activase: Rubisco's catalytic chaperone. Photosynth Res. 2003;75:11–27.
- Pollock SV, Colombo SL, Prout DL Jr, et al. Rubisco activase is required for optimal photosynthesis in the green alga Chlamydomonas reinhardtiiin a low-CO2 atmosphere. Plant Physiol. 2003;133:1854–1861.
- Mate CJ, von Caemmerer S, Evans JR, et al. The relationship between CO2-assimilation rate, Rubisco carbamylation and Rubisco activase content in activase-deficient transgenic tobacco suggests a simple model of activase action. Planta. 1996;198:604–613.
- von Caemmerer S, Hendrickson L, Quinn V, et al. Reductions of Rubisco activase by antisense RNA in the C4 plant Flaveria bidentis reduces Rubisco carbamylation and leaf photosynthesis. Plant Physiol. 2005;137:747–755.
- Kallis RP, Ewy RG, Portis AR Jr. Alteration of the adenine nucleotide response and increased Rubisco activation activity of Arabidopsis Rubisco activase by site-directed mutagenesis. Plant Physiol. 2000;123:1077–1086.
- Portis AR Jr, Li C, Wang D, et al. Regulation of Rubisco activase and its interaction with Rubisco. J Exp Bot. 2008;59:1597–1604.
- Salvucci ME, van de Loo FJ, Stecher D. Two isoforms of Rubisco activase in cotton, the products of separate genes not alternative splicing. Planta. 2003;216:736–744.
- Shen JB, Orozco EM Jr, Ogren WL. Expression of the two isoforms of spinach ribulose 1,5-bisphosphate carboxylase activase and essentiality of the conserved lysine in the consensus nucleotide-binding domain. J Biol Chem. 1991;266:8963–8968.
- Werneke JM, Zielinski RE, Ogren WL. Structure and expression of spinach leaf cDNA encoding ribulose bisphosphate carboxylase/oxygenase activase. Proc Natl Acad Sci USA. 1988;85:787–791.
- Werneke JM, Chatfield JM, Ogren WL. Alternative mRNA splicing generates the two ribulosebisphosphate carboxylase/oxygenase activase polypeptides in spinach and Arabidopsis. Plant Cell. 1989;1:815–825.
- To KY, Suen DF, Chen SCG. Molecular characterization of ribulose-1,5-bisphosphate carboxylase/oxygenase activase in rice leaves. Planta. 1999;209:66–76.
- Law RD, Crafts-Brandner SJ. High temperature stress increases the expression of wheat leaf ribulose-1,5-bisphosphate carboxylase/oxygenase activase protein. Arch Biochem Biophys. 2001;386:261–267.
- Rundle SJ, Zielinski RE. Organization and expression of two tandemly oriented genes encoding ribulosebisphosphate carboxylase/oxygenase activase in barley. J Biol Chem. 1991;266:4677–4685.
- Qian J, Rodermel SR. Ribulose-1,5-bisphosphate carboxylase/oxygenase activase cDNAs from Nicotiana tabacum. Plant Physiol. 1993;102:683–684.
- Yin Z, Meng F, Song H, et al. Expression quantitative trait loci analysis of two genes encoding rubisco activase in soybean. Plant Physiol. 2010;152:1625–1637.
- Li B, Chi D, Chen H, et al. The relationship between physiological characteristics and yield of hickory. Forest Sci Technol. 2007;21:34–37.
- Wang Z, Huang Y, Xia G, et al. Cloning and analysis of the APETALA1(AP1) homolog gene from Carya cathayensis. J Zhejiang Forest Coll. 2008;25:427–430.
- Saitou N, Nei M. The neighbor-joining method: a new method for reconstructing phylogenetic trees. Mol Biol Evol. 1987;4:406–425.
- Ogura T, Wilkinson AJ. AAA+ superfamily ATPases: common structure—diverse function. Genes Cells. 2001;6:575–597.
- Bayramov S, Guliyev N. Changes in Rubisco activase gene expression and polypeptide content in Brachypodium distachyon. Plant Physiol Biochem. 2014;81:61–66.
- Werneke JM, Ogren WL. Structure of an Arabidopsis thaliana cDNA encoding Rubisco activase. Nucleic Acids Res. 1989;17:2871.
- Jurczyk B, Hura K, Trzemecka A, et al. Evidence for alternative splicing mechanisms in meadow fescue (Festuca pratensis) and perennial ryegrass (Lolium perenne) Rubisco activase gene. J Plant Physiol. 2015;176:61–64.
- Jiang Y, Yong B, Chen J, et al. Two Rubisco activase genes from Ipomoea Batatas have different roles in photosynthesis of Arabidopsis. Pak J Bot. 2014;46:1381–1388.
- Wand D, Xie SE, Yang J, et al. Molecular characteristics and expression patterns of Rubisco activase, novel alternative splicing variants in a heterophyllous aquatic plant, Sagittaria graminea. Photosynthetica. 2014;52:83–95.
- Eckardt NA, Snyder GW, Portis AR Jr, et al. Growth and photosynthesis under high and low irradiance of Arabidopsis thaliana antisense mutants with reduced ribulose-1,5-bisphosphate carboxylase/oxygenase activase content. Plant Physiol. 1997;113:575–586.
- Fukayama H, Ueguchi C, Nishikawa K, et al. Overexpression of Rubisco activase decreases the photosynthetic CO2 assimilation rate by reducing Rubisco content in rice leaves. Plant Cell Physiol. 2012;53:976–986.
- Wang D, Lu Q, Li XF, et al. Relationship between Rubisco activase isoform levels and photosynthetic rate in different leaf positions of rice plant. Photosynthetica. 2009;47:621–629.
- Zhang G, Wang W, Zou Q. Molecular biology of Rubisco activase. Plant physiol commun. 2004;40:633–637.
- Kumar A, Portis AR Jr, Li C. Arabidopsis thaliana expressing a thermo stable chimeric Rubisco activase exhibits enhanced growth and higher rates of photosynthesis at moderately high temperatures. Photosynth Res. 2009;100:143–153.