ABSTRACT
Bacterial resistance to antibiotics, first a major concern in the 1960s, has re-emerged worldwide over the last 20 years. The World Health Organization (WHO) and other health organizations have, therefore, declared ‘war’ against human microbial pathogens, particularly hospital-acquired infections, and have made drug discovery a top priority for these diseases. Because these bacteria are refractory to conventional chemotherapy, medicinal and herbal plants used in various countries should be assessed for their therapeutic potential; these valuable bio-resources are a reservoir of complex bioactive molecules. Earlier studies from our laboratory on Rhazya stricta, a native herbal shrub of Asia, have shown that this plant has a number of therapeutic properties. In this study, we evaluated the antimicrobial activities of various concentrations of five solvent extracts (aqueous alkaloid, aqueous non-alkaloid, organic alkaloid, organic non-alkaloid and whole aqueous extracts) derived from R. stricta leaves against several multidrug-resistant, human-pathogenic bacteria, including methicillin-resistant Staphylococcus aureus (MRSA) and extended-spectrum beta-lactamase-positive Escherichia coli. In vitro, molecular and electron microscopy analyses conclusively demonstrated the antimicrobial effects of these extracts against a panel of Gram-negative and Gram-positive bacteria. The organic alkaloid extract was the most effective against E. coli and MRSA, resulting in cell membrane disruption visible with transmission electron microscopy. In the near future, we intend to further focus and delineate the molecular mechanism-of-action for specific alkaloids of R. stricta, particularly against MRSA.
Introduction
All over the world, a vast diversity of flora needs to be explored to discover and develop novel antimicrobial drugs. According to the World Health Organization (WHO), medicinal herbs could be an important resource for developing new antimicrobial drugs.[Citation1] The medicinal plant diversity in the Saudi Arabian peninsula presents a genetically, biochemically and ecologically diverse pool that can be utilized to discover new treatments for an array of infectious diseases. The evolutionary selection pressures undergone by these desert herbs have given rise to ‘survival molecules’ and secondary metabolites that counter local pathogenic microbes.[Citation2]
Traditional and complementary medicine is used by the majority of the world's population.[Citation3] Several studies have shown that herbal extracts commonly used in folkloric medicine have high bactericidal or bacteriostatic potential.[Citation3] Indeed, conventional antibiotics are generally secondary metabolites of microbial or plant origin with low molecular weight (less than 1000 Da).[Citation4] A recent survey reveals that natural products or natural product-based drugs account for >75% of the 97 antibacterial new chemical entities (NCEs) approved and marketed over the last three decades [Citation5] emphasizing the ongoing importance of natural products as the basis for new treatments of bacterial infectious diseases.[Citation3]
The rising problem of antibiotic resistance is a major threat to public health worldwide.[Citation6] Antimicrobial resistance continues to increase among key pathogens, placing a significant burden on health care systems and humanity.[Citation6–9] Multi drug resistant (MDR) is a troubling cause of treatment failure in several infectious diseases [Citation10] and, thus, antibiotic resistance is one of the major challenges for health care in the twenty-first century.
Bacteria possess numerous adaptations that result in resistance to antibiotic therapies. Extended-spectrum beta-lactamases (ESBLs) are a highly heterogeneous group of enzymes that confer resistance to beta-lactam antibiotics.[Citation11] ESBL-producing MDR bacteria, such as Klebsiella spp. and Escherichia coli, are emerging due to selection pressure exerted by the misuse of antibiotics.[Citation12] Infections caused by ESBL-producing Klebsiella and E. coli species have been on the increase in frequency and severity.[Citation13]
Collectively, Staphylococcus aureus and E. coli represent exemplary model organisms for Gram-positive and Gram-negative bacteria that can cause numerous diseases.[Citation14] Broad-spectrum antimicrobial agents can eliminate infections by these pathogens, but they can also result in the development of further drug resistance and carry possible side effects.[Citation15] Due to the emergence of micro-organisms’ resistance to several commonly available antibiotics, there is a dire need to develop new antibiotics.[Citation16]
The plant genus Rhazya belongs to the order Gentianales, family Apocynaceae [Citation17] and subfamily Rauwolfioideae.[Citation2] Rhazya stricta (known in Saudi Arabia as harmal) is a toxic, evergreen, small, erect shrub with glabrous leaves. It is used in local medicinal herbal drugs to cure various ailments in Afghanistan, India, Iran, Iraq, Pakistan, Qatar, United Arab Emirates (UAE) and Saudi Arabia.[Citation18,Citation19] Tetrahydrosecamine isolated from R. stricta demonstrates broad-spectrum antimicrobial activity, and another active component, strictanol, was found to be most active against E. coli and Pseudomonas aeruginosa.[Citation2] The crude ethanolic extract of R. stricta fruit has antibacterial, lipoxygenase and acetylcholinesterase activities.[Citation20] The chloroform and methanol extracts of R. stricta roots show antimicrobial and antifungal activities against Bacillus subtilis, P. aeruginosa, E. coli, S. aureus, Aspergillus terreus, Aspergillus flavus and Candida albicans. Although the aqueous extract of R. stricta leaves has genotoxic effects indicated by cytogenetic and molecular evaluation against fungal cells, including Saccharomyces cerevisiae [Citation21] and A. terreus,[Citation19] only a few studies have been conducted to assess the antibacterial activities of the aqueous extract of R. stricta leaves.[Citation22,Citation23]
In this study, the antimicrobial activities of different solvent extracts derived from R. stricta leaves (aqueous alkaloid (AA), aqueous non-alkaloid (AN), organic alkaloid (OA), organic non-alkaloid (ON) and whole aqueous extract (WAE)) against MDR human pathogens have been determined, and their effects at the microbiological, molecular and ultrastructural levels have been evaluated.
Material and methods
Chemicals for antimicrobial assays
Ammonium hydroxide, chloroform, ethanol, glacial acetic acid, dimethyl sulphoxide (DMSO), phosphotungstic acid (PTA) and several other reference chemicals and reagents used in this study were obtained from Sigma-Aldrich, USA; Invitrogen, USA and Biomatik, USA. Bacterial culture media and standard antibiotic discs were purchased from HiMedia, Mumbai, India. Analytical grade reagents/chemicals were used in this experiment.
Bacterial strains
All clinical bacterial isolates used in this study () were obtained from the clinical microbiology laboratory of King Abdulaziz University Hospital, Jeddah, KSA. Klebsiella pneumonia, ESBL−VE (isolate no. 14), isolated from urine sample, is resistant to ampicillin. E. coli, ESBL−VE (isolate no. 17), isolated from urine sample, is sensitive to various antibiotics. K. pneumonia, ESBL−VE, (isolate no. 18), isolated from urine sample, is resistant to ampicillin and nitrofurantoin. Vancomycin-resistant Enterococcus faecium VAN B (isolate no. 20), obtained from patient's urine sample, is resistant to oxacillin, vancomycin, cefazolin and amoxicillin/clavulanic acid. Streptococcus group B (isolate no. 21), isolated from urine, is sensitive to various antibiotics. K. pneumonia, ESBL+VE (isolate no. 36), isolated from urine, is resistant to colistin, gentamycin, amoxicillin/clavulanic acid and nitrofurantoin. K. pneumonia, ESBL+VE (isolate no. 38), isolated from urine, is resistant to gentamycin, amoxicillin/clavulanic acid. E. coli, ESBL+VE (isolate no. 26), isolated from superficial wound sample, is resistant to amikacin, ciprofloxacin, gentamycin and trimethoprim/sulphamethoxazole. E. coli, ESBL+VE (isolate no. 30), isolated from superficial wound sample, is resistant to ciprofloxacin and trimethoprim/sulphamethoxazole. E. coli, ESBL+VE (isolate no. 33), isolated from deep wound sample, is resistant to trimethoprim/sulphamethoxazole. E. coli, ESBL+VE (isolate no. 34), isolated from deep wound sample, is resistant to trimethoprim/sulphamethoxazole and ciprofloxacin. Methicillin-resistant Staphylococcus aureus (MRSA) (isolate no. 27), isolated from superficial wound sample, is resistant to oxacillin and vancomycin, while another MRSA (isolate no. 39), isolated from superficial wound sample, is resistant to clindamycin, erythromycin, oxacillin and cefazolin.
Table 1. Antibacterial activity of the different crude extracts of Rhazya stricta against the various human-pathogenic MDR bacteria.
Plant material
Leaves of R. stricta were collected from an area near the village Bahara along the Jeddah--Makkah road in the KSA. The species was identified and authenticated to the genus and species level by Professor Nabih A. Baeshen according to Baeshin et al.[Citation21] The sample of leaf collection was deposited in King Abdulaziz University harbarium, Jeddah, KSA).
Preparation of OA and ON extracts from R. stricta leaves
Leaves were washed and dried under shade and then ground to prepare a fine powder. Eight hundred millilitres of absolute ethanol (95%) was added to 500 g powder to produce an extract; this mixture was allowed to stand for two days. The extract was collected by filtration through Whatman filter paper (125 mm) and then evaporated by rotary evaporator (Buchi, Switzerland). Twenty-five grams of solid extract was removed, and 200 mL each of 1 mol/L HCl and chloroform were added. The chloroform layer, which contained non-alkaloids (ON), was separated and collected. Ammonium hydroxide was added to the acidic water layer to make it alkaline. An equal volume of chloroform was then added to the alkaline water layer; the chloroform layer, which contained alkaloids (OA), was separated, collected and stored at 4 °C.
Preparation of AA and AN extracts from R. stricta leaves
AA and AN were extracted according to Elkady.[Citation24] Briefly, fresh R. stricta leaves were washed and dried under shade. Dried leaves (350 g) were soaked in 80% ethanol for three days and then filtered and evaporated using a rotary evaporator (Buchi, Switzerland). Eighty millilitre distilled water and 20 mL glacial acetic acid were added; the solution was shaken vigorously and the extract was collected by filtration through Whatman filter paper (Grade 1) filtered. An equal volume of chloroform was added, and the solution was shaken for 2 h. Two layers were formed; the upper layer was discarded, and the pH of the lower layer was neutralized with HCl. An equal volume of chloroform was added, and the solution was shaken for 3 h. Finally, two layers were formed, one the chloroform layer, from which alkaloids were extracted, and the other the aqueous layer, from which non-alkaloids were extracted; the separate contents of both layers were immediately freeze-dried.[Citation24]
Preparation of whole aqueous extract from R. stricta leaves
WAE was prepared according to our laboratory protocol as described by Kabli et al.[Citation23] In brief, washed fresh leaves of R. stricta were cut into small pieces and 96 g were taken along with 1 L of distilled water in a blender The mixture was left for 24 h in a shaker at room temperature. The mixture was later filtered with filter paper (125 mm), and then with membrane filters (0.22 μm). The stock solution with a concentration of 96 g/L was stored at 4 °C.
Antibacterial activities of R. stricta extract
Antimicrobial activities of R. stricta crude extracts on various human-pathogenic bacteria: E. faecium (vancomycin-resistant E. faecium (VRE)), E. coli (ESBL-negative and ESBL-positive), K. pneumoniae (ESBL-negative and ESBL-positive), Group B Streptococcus, S. aureus (MRSA and methicillin-susceptible) were assessed. Antibacterial activity of different fractions of R. stricta extracts (i.e. OA, AA, OA + AA, ON, AN, ON + AN, WAE), along with different solvent controls (10% DMSO, sterile distilled water [SDW] and Mueller Hinton broth) was tested in 96-well micro-plates. Varying concentrations of R. stricta extracts [Citation25] and WAE fractions [Citation23] were prepared. Separate OA and AA extracts, as well as mixtures of both (OA + AA), were prepared at varying concentrations (4.4, 5.5, 6.6 and 7.7 mg/mL). Separate ON and AN extracts, as well as mixtures of both (ON + AN), were also prepared at varying concentrations (4.9, 6.1, 7.3 and 8.5 mg/mL). Fifty microlitres of fresh bacterial cultures with 50 µL WAE of different concentrations (3.0, 6.0, 12.0 and 18.0 mg/mL) were also included in the study design. Several wells in each plate served as negative controls (no inoculum added, no extract added and only solvent added [i.e. 10% DMSO, 0.04% methanol and SDW]). The plates were shaken gently, incubated for 24 h at 35 °C in a humidified incubator, and assayed for bacterial growth visually and by absorbance measurements using an enzyme-linked immunosorbent assay (ELISA) reader device (ELx Absorbance Reader ELISA system; BioTek Instruments, USA). The optical density of each well was measured at 0 h and after 24 h incubation periods using an ELISA reader device at 630 nm.[Citation26]
Transmission electron microscopy
Samples of clinical isolates of E. coli and MRSA were investigated by negative contrast transmission electron microscopy (TEM), as described by Haschemyer and Meyers.[Citation27] Bacteria were transferred to a 300 mesh carbon-coated copper grid by keeping the grid coated-side-down on a drop of MRSA or E. coli culture for about 2 min. Excess liquid was removed by touching the grid with a piece of Whatman filter paper, and bacteria were then negatively stained by floating the grid on a drop of 2% (w/v) PTA solution (pH 6.5) for 1 min. Excess liquid was again removed by touching the grid with a piece of Whatman filter paper, and the bacterial preparation was examined by TEM using a JEM-1011 microscope (Jeol, Tokyo, Japan) at 80 KV accelerated voltage.
To investigate changes in ESBL gene expression after treatment with R. stricta extracts
To investigate changes in ESBL gene expression after treatment with R. stricta extracts, genomic DNA was extracted using Wizard Genomic DNA Purification Kit. Polymerase chain reaction (PCR) amplification of beta-lactamase genes was performed using DNA primers specific for the TEM-5 and SHV-1 genes. Primers used for TEM-5 genes were: 5’ CTT CCT GTT TTT GCT CAC CCA 3’ and 5’ TAC GAT ACG GGA GGG CTT AC 3’; primers used for SHV-1 genes were: 5’ TCA GCG AAA AAC ACC TTG 3’ and 5’ TCC CGC AGA TAA ATC ACC 3’. PCR analysis for TEM-5 and SHV-1 was carried out according to Sharma et al.[Citation28] PCR products were electrophoresed onto 1% agarose gels in tris-acetate-EDTA (TAE) buffer, and amplified products were visualized under UV light. The resolved bands were visualized using the gel documentation system. Fragment size was determined according to a standardized DNA ladder (Cleaver Scientific's 1 Kb DNA ladder). PCR products with correct band sizes were collected (TEM-1: 500 bp; SHV-1: 650 bp) by excision from the gel with a sterile scalpel. The SHV and TEM genes, conserved region and its primers were sent to a private company for sequencing (Macrogen, South Korea). The sequences of both control and treated bacteria were aligned with published sequences for the SHV and TEM genes using basic local alignment search tool (BLAST) (National Center for Biotechnology Information, USA).
Results and discussion
The antimicrobial activities of R. stricta leaf extracts were tested against MDR Gram-positive and Gram-negative bacteria. The alkaloid fractions (organic, aqueous and the mixture of both) were active against various MDR bacteria, including ESBL-positive E. coli (isolates 20, 30, 33, 34) and K. pneumoniae (isolate 36) and ESBL-negative E. coli (isolate 17), K. pneumoniae (isolate 38), VRE and group B Streptococcus. The non-alkaloid fractions (organic, aqueous and the mixture of both) also showed activity against various MDR bacteria, including ESBL-positive E. coli (isolates 26, 30, 33, 34,) and Gram-negative E. coli (isolates 17), ESBL-positive K. pneumonia (isolate 36), ESBL-negative K. pneumonia (isolates 14, 18, 38) and E. faecium (VRE). WAE of R. stricta was also found to be effective against all pathogens tested () except Streptococcus of group B (isolate 21), and K. pneumoniae showed ESBL+VE (isolate 36). Various solvent controls such as 10% DMSO, 0.04% methanol and SDW did not inhibit the bacterial growth.
Morphological alterations after the treatment of bacterial cells with different concentrations of R. stricta extracts were evident after TEM analysis. Ultrastructural examination of treated bacterial cells revealed that R. stricta extract-treated clinical pathogens had extra and intracellular changes not seen in non-treated cells, including separation of the cytoplasmic membrane from the cell envelope, coagulation of the cytosolic components and disruption of the outer membrane structure with membrane sloughing, breaching and blebbing ( and ; not all bacterial species investigated re-depicted). We amplified specific genes for ESBL in E. coli, TEM-5 and SHV-1, before and after treatment with R. stricta extracts, comparing treatment effects of those extracts on the sequences of TEM-5 and SHV-1 genes (; –Citation5).
Figure 1. Transmission electron micrograph of negatively stained preparations of ESBL-positive E. coli cells treated with R. stricta extracts. (A) ‘Negative control (0.04% methanol) cell (bar = 1 µm)’ of E. coli (bar = 1 µm). (B) Treatment with organic non-alkaloid extract (0.49 mg/mL), resulting in alterations due to bactericidal effects (bar = 1 µm). (C) Treatment with aqueous non-alkaloid extract (0.85 mg/mL), resulting in bulging and cell wall alteration (bar = 1 µm). (D) Treatment with organic non-alkaloid extract (0.85 mg/mL), resulting in rupture of the cell wall (bar = 1 µm). (E) Treatment with non-alkaloid extract (0.85 mg/mL), resulting in cytoplasmic leakage (bar = 1 µm). (F) Treatment with whole aqueous extract (96 g/L), resulting in cell wall bulging and shrunken cytoplasm (bar = 0.5 µm).
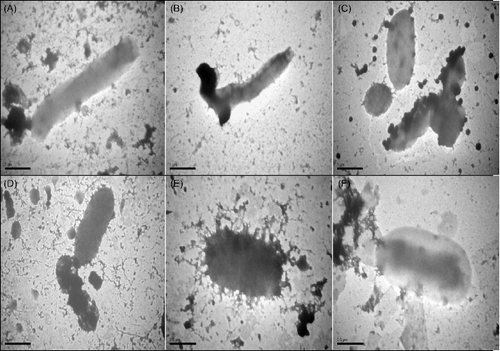
Figure 2. Transmission electron micrograph of negatively stained preparations of methicillin-resistant Staphylococcus aureus (MRSA) cells exposed to R. stricta leaf extracts. (A) Control cell. (B) Treatment with organic alkaloid extract (7.7 mg/mL), resulting in shrunken cytoplasm. (C) Treatment with aqueous alkaloid extract (7.7 mg/mL), resulting in burst cells. (D) Treatment with organic non-alkaloid extract (7.3 mg/mL), resulting in distorted cells. (E) Treatment with organic non-alkaloid extract (8.5 mg/mL), resulting in cell wall alterations. (F) Whole aqueous extract (96 g/L), resulting in shrunken cytoplasm. Bar = 0.5 µm in all panels.
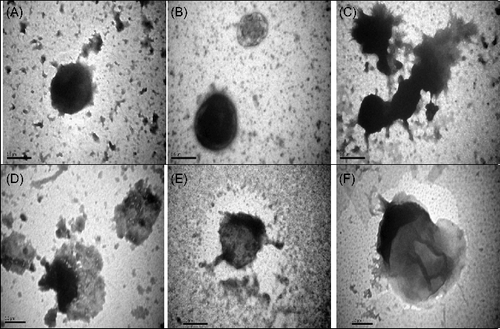
Table 2. Sequences producing significant alignments between S1 F (control) and all treated S2 F, S3 F, S4 F and S5 F.
Figure 3. Banding pattern of TEM-5 gene of three E. coli isolates (17, 30 and 33) revealed by gel electrophoresis pre- and post-treatment with different concentrations of Rhazya stricta leaf extracts DNA marker. T1: isolate 17 without treatment; T2: isolate 17 treated with organic alkaloid extract (4.4 mg/mL); T3: isolate 17 treated with organic alkaloid extract (7.7 mg/mL); T4: isolate 17 treated with aqueous alkaloid (4.4 mg/mL); T5: isolate 17 treated with aqueous alkaloid extract (7.7 mg/mL); T6: isolate 30 without treatment; T7: isolate 30 treated with organic non-alkaloid extract (6.1 mg/mL); T8: isolate 30 treated with organic non-alkaloid extract (8.5 mg/mL); T9: isolate 33 without treatment; T10: isolate 33 treated with organic alkaloid and aqueous alkaloid extract (4.4 mg/mL); T11: isolate 33 treated with organic alkaloid and aqueous alkaloid extract (7.7 mg/mL).
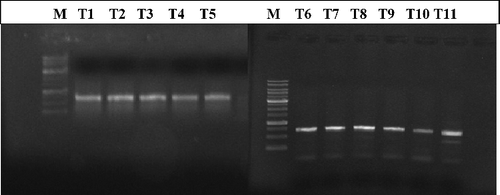
Figure 4. Banding pattern of SHV-1 gene of three E. coli isolates (17, 30 and 33) revealed by gel electrophoresis pre- and post-treatment with different concentrations of Rhazya stricta leaf extracts. M: DNA marker; S1: isolate 17 without treatment; S2: isolate 17 treated with organic alkaloid extract (4.4 mg/mL); S3: isolate 17 treated with organic alkaloid extract (7.7 mg/mL); S4: isolate 17 treated with aqueous alkaloid extract (4.4 mg/mL); S5: isolate 17 treated with aqueous alkaloid extract (7.7 mg/mL); S6: isolate 30 without treatment; S7: isolate 30 treated with organic non-alkaloid extract (6.1 mg/mL); S8: isolate 30 treated with organic non-alkaloid extract (8.5 mg/mL); S9: isolate 33 without treatment; S10: isolate 33 treated with organic alkaloid and aqueous alkaloid extract (4.4 mg/mL); S11: isolate 33 treated with organic alkaloid and aqueous alkaloid (7.7 mg/mL).
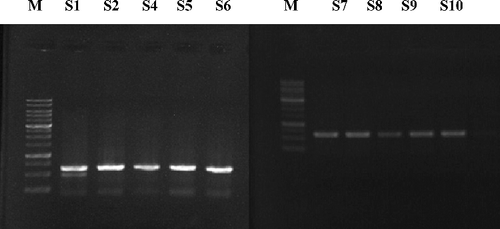
Figure 5. Sequence alignment for SHV-1 between S1F: (control; 10% DMSO) and treated with organic alkaloid at 4.4 mg/mL treated E. coli (isolate 17). Highlighted sequences indicated alterations.
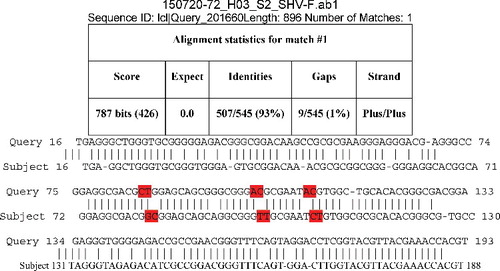
The increased prevalence of bacterial resistance to commonly used antibiotics has made susceptibility testing necessary when treating life-threatening bacterial infections. Antibiotic policies must be revolutionized in health care, and these changes depend on the adoption of new antibiotics with novel activity spectra, pharmacokinetics and pharmacodynamics.
Extensive use of antibiotics exerts selection pressure that leads to antibiotic resistance. Indeed, the association of antibiotic use and the development of antibiotic resistance have been recognized as a cause of nosocomial and community-acquired infections. ESBLs, including TEM and SHV enzymes,[Citation29] have been identified in Gram-negative bacteria such as K. pneumonia and E. coli; this adaptation has contributed enormously to the increased resistance to a broad range of antibiotics currently in use.
In the last 50–60 years, products from medicinal plants have driven pharmaceutical invention and are proving to be the most important resource for NCEs.[Citation30] Plants are a rich source of secondary metabolites, such as alkaloids, terpenoids, tannins and flavonoids, which have antimicrobial properties.[Citation31] Plant extracts have been the main source of crude therapeutic formulations for traditional and complementary medicine in Asian countries. With technological advancements, drug formulations have been purified, standardized isolated, screened and clinically developed into new drug molecules.[Citation30] Thus, natural plant products are a key source of therapeutic agents. Indeed, several studies have established the antimicrobial properties of plant products.[Citation12,Citation15,Citation31–33]
In our study, the activity of R. stricta extracts against Gram-positive and Gram-negative bacteria revealed the plant's potential as an antimicrobial agent. Our findings of antimicrobial activity corroborate the results of previous studies.[Citation12,Citation15,Citation34] Certain R. stricta extracts (WAE, AN, AA and AN + ON) showed low activities against a limited number of strains (), a finding that Lacmata et al.[Citation35] also reported. These low activities could be due to mutations present in these multidrug-resistant bacteria that block or circumvent shared mechanisms of action of R. stricta extracts and other antibiotics. However, other R. stricta extracts (ON, OA and AA + OA) had clear antimicrobial activity on MDR Gram-negative and Gram- positive bacteria.
Table 3. Susceptibility/resistance of clinical pathogens toward different extracts of Rhazya stricta leaves at various concentrations.
Electron micrographs of clinical isolates (MRSA and ESBL-positive E. coli) showed dramatic alterations in pathogen morphology after exposure to R. stricta leaf extracts. In all pathogens and across fraction types, cytoplasmic granularity indicated the presence of DNA fibrils. This intracytoplasmic coagulation is probably due to the micro-precipitation of abnormal proteins or denatured membranes [Citation36,Citation37] that resulted from treatment with R. stricta leaf extracts. Furthermore, in MRSA cells, cell wall disruptions that resulted in the release of intracellular material and, ultimately, empty and flaccid cells were observed ((D,F)), as were ghost cells, empty cellular envelopes structure devoid of cytoplasmic content (including genetic material; (E)). Collectively, the cellular damage seen on TEM examination indicated the lethal effects of R. stricta leaf extracts on the pathogens.
These observations suggest that treatment with R. stricta extracts either quickly killed the cells or inhibited growth by directly disrupting their membranes. Others have reported similar disruptions to cellular function and morphological parameters. Treatment of E. coli O157:H7 with oregano and thyme essential oil results in severe alterations visible with scanning electron microscope (SEM),[Citation38] and the action of Piper betle L. leaf extract on Streptococcus mutans results in nucleoid and plasma membrane disruption visible with TEM that results in cytoplasmic loss [Citation39] Furthermore, the exposure of P. aeruginosa to tea polyphenols resulted in alteration and disruption of the cell membrane.[Citation40]
Our TEM results are in accordance with a rapid decrease in microbial viability after treatment (). We also explored the antimicrobial effects of R. stricta extracts at the molecular level. Genomic DNA indicated polymorphisms in treated ESBL-positive and negative E. coli not present in the untreated control strains. This may also indicate the susceptibility of specific strains of bacteria to key alkaloids and other therapeutic constituents present in the extracts of R. stricta.
Conclusions
Our data clearly demonstrate the antibacterial effects of R. stricta leaf extracts on various pathogenic strains. These results indicate the possibility of using R. stricta products as a source of antibacterial compounds for the treatment of bacterial infections and clearly have global ramifications. Further, in vitro and in vivo studies are required to extract and examine the antimicrobial properties of specific alkaloids derived from R. stricta.
Acknowledgment
We would like to thank the laboratory of King Fahd Hospital, and Microbial Analysis Unit, King Fahd Medical Research Center, King Abdulaziz University, Jeddah, Saudi Arabia for the help during study. The authors gratefully acknowledge technical and financial support of Deanship of Scientific Research and Deanship of Graduate Studies, Abdulaziz University, Jeddah, Saudi Arabia.
Disclosure statement
No potential conflict of interest was reported by the authors.
Additional information
Funding
References
- Khan R, Islam B, Akram M, et al. Antimicrobial activity of five herbal extracts against multi drug resistant (MDR) strains of bacteria and fungus of clinical origin. Molecules. 2009;14(2):586–597.
- Akhgari A. Alkaloids of in vitro cultures of Rhazya stricta [dissertation]. Helsinki: university of Helsinki; 2015.
- Taylor PW. Alternative natural sources for a new generation of antibacterial agents. Int J Antimicrob Agents. 2013;42(3):195–201.
- Fabbretti A, Gualerzi CO, Brandi L. How to cope with the quest for new antibiotics. FEBS Lett. 2011;585(11):1673–1681.
- Newman DJ, Cragg GM. Natural products as sources of new drugs over the last 25 years. J Nat Prod. 2007;70(3):461–477.
- Högberg LD, Heddini A, Cars O. The global need for effective antibiotics: challenges and recent advances. Trends Pharmacol Sci. 2010;31(11):509–515.
- Tanaka J, Da Silva C, De Oliveira A, et al. Antibacterial activity of indole alkaloids from Aspidosperma ramiflorum. Braz J Med Biol Res. 2006;39:387–391.
- Kumarasamy KK, Toleman MA, Walsh TR, et al. Emergence of a new antibiotic resistance mechanism in India, Pakistan, and the UK: a molecular, biological, and epidemiological study. Lancet Infect Dis. 2010;10(9):597–602.
- Davies J. How to discover new antibiotics: harvesting the parvome. Curr Opin Chem Biol. 2011;15(1):5–10.
- Kuete V, Alibert-Franco S, Eyong K, et al. Antibacterial activity of some natural products against bacteria expressing a multidrug-resistant phenotype. Int J Antimicrob Agents. 2011;37(2):156–161.
- Perez F, Endimiani A, Hujer KM, et al. The continuing challenge of ESBLs. Curr Opin Pharmacol. 2007;7(5):459–469.
- Zuo GY, Meng FY, Han J, et al. In vitro activity of plant extracts and alkaloids against clinical isolates of extended-spectrum b-Lactamase (ESBL)-producing strains. Molecules. 2011;16(7):5453–5459.
- Rattan A, Raj VS, Saini KS. Recent advances in the discovery and development of new drugs against gram-negative pathogens. In: Atta-ur-Rahman, editor. Frontiers in clinical drug research – anti-infectives. Vol. 1. Sharjah: Bentham Science Publishers; 2014.
- Nyatoti VN, Mtero SS, Rukure G. Pathogenic Escherichia coli in traditional African weaning foods. Food Control. 1997;8(1):51–54.
- Li N, Tan SN, Cui J, et al. PA-1, a novel synthesized pyrrolizidine alkaloid, inhibits the growth of Escherichia coli and Staphylococcus aureus by damaging the cell membrane. J Antibiot. 2014;67:689–696.10
- Wright GD. Antibiotics: a new hope. Chem Biol. 2012;19(1):3–10.
- Baeshen NA, Elkady AI, Abuzinadah OA, et al. Potential anticancer activity of the medicinal herb, Rhazya stricta, against human breast cancer. Afr J Biotechnol. 2012;11:8960–8972.37
- Gilani SA, Kikuchi A, Shinwari ZK, et al. Phytochemical, pharmacological and ethnobotanical studies of Rhazya stricta Decne. Phytother Res. 2007;21(4):301–307.
- Baeshin NA, Qari SH, Sabir JS, et al. Biochemical and molecular evaluation of genetic effects of Rhazya stricta (Decne) leafs extract on Aspergillus terreus. Saudi J Biol Sci. 2008;15(1):25–33.
- Sultana N, Khalid A. Phytochemical and enzyme inhibitory studies on indigenous medicinal plant Rhazya stricta. Nat Prod Res. 2010;24(4):305–314.
- Baeshin NA, El-Twaty NH, Al-Hebshi AM. Evaluating the genotoxicity of Rhazya stricta leaves extract by the Saccharomyces cerevisiae auxotrophic mutants test. Egypt J Nat Tox. 2005;2:87–100.
- Abadi F, Abdulaziz A, Hadhoud A, et al. An epidemiological survey and evaluation of the antimicrobial growth effect of Rhazya stricta (Decne) leaves extract on different genotypes of Neisseria meningitides. Egypt J Med Microbiol. 2011;20(2):77–86.
- Kabli SAA, Hadhoud AEDA, Baeshen MN. An epidemiological survey of extended-spectrum β-lactamases producing bacteria genotypes and the evaluation of the antimicrobial effect of Rhazya stricta leaf extract. Microbiol Res. 2012;3(2):66–73.
- Elkady AI. Crude alkaloid extract of Rhazya stricta inhibits cell growth and sensitizes human lung cancer cells to cisplatin through induction of apoptosis. Genet Mol Biol. 2013;36(1):12–21.
- Baeshen NA, Elkady AI, Yaghmoor SS, et al. Evaluation of the cytotoxicity and genotoxicity of alkaloid-rich and alkaloid-free aqueous extracts of Rhazya stricta leaves. Bothalia J. 2014;44(5):358–371.
- Shukla R, Singh P, Prakash B, et al. Antifungal, aflatoxin inhibition and antioxidant activity of Callistemon lanceolatus (Sm.) Sweet essential oil and its major component 1, 8-cineole against fungal isolates from chickpea seeds. Food Control. 2012;25(1):27–33.
- Haschemyer RH, Meyers RJ. Negative staining. In: Hayat MA, editor. Principles and techniques of electron microscopy. Vol. 2. New York, NY: Van Nostrand Reinhold; 1972. p. 101–143.
- Sharma J, Sharma M, Ray P. Detection of TEM & SHV genes in Escherichia coli & Klebsiella pneumoniae isolates in a tertiary care hospital from India. Indian J Med Res. 2010;132:332–336.
- Khan R, Zakir M, Afaq SH, et al. Activity of solvent extracts of Prosopis spicigera, Zingiber officinale and Trachyspermum ammi against multidrug resistant bacterial and fungal strains. J Infect Dev Count. 2010;4(05):292–300.
- Mishra BB, Tiwari VK. Natural products: an evolving role in future drug discovery. Eur J Med Chem. 2011;46(10):4769–4807.
- Karaman I, Şahin F, Güllüce M, et al. Antimicrobial activity of aqueous and methanol extracts of Juniperus oxycedrus L. J Ethnopharmacol. 2003;85(2):231–235.
- Prabuseenivasan S, Jayakumar M, Ignacimuthu S. In vitro antibacterial activity of some plant essential oils. BMC Complement Altern Med. 2006;6(1):39.
- Mohtar M, Johari SA, Li AR, et al. Inhibitory and resistance-modifying potential of plant-based alkaloids against methicillin-resistant Staphylococcus aureus (MRSA). Curr Microbiol. 2009;59(2):181–186.
- Rasooli I, Rezaei MB, Allameh A. Ultrastructural studies on antimicrobial efficacy of thyme essential oils on Listeria monocytogenes. Int J Infect Dis. 2006;10(3):236–241.
- Lacmata ST, Kuete V, Dzoyem JP, et al. Antibacterial activities of selected Cameroonian plants and their synergistic effects with antibiotics against bacteria expressing MDR phenotypes. Evid-Based Complement Altern Med. 2012;12:1–11
- Gustafson JE, Liew YC, Markham J, et al. Effects of tea tree oil on Escherichia coli. Lett Appl Microbiol. 1998;26:194–198.3
- Becerril R, Gómez-Lus R, Goñi P, et al. Combination of analytical and microbiological techniques to study the antimicrobial activity of a new active food packaging containing cinnamon or oregano against E. coli and S. aureus. Anal Bioanal Chem. 2007;388:1003–1010.
- Burt SA, Reinders RD. Antibacterial activity of selected plant essential oils against Escherichia coli O157: H7. Lett Appl Microbiol. 2003;36(3):162–167.
- Nalina T, Rahim Z. The crude aqueous extract of Piper betle L. and its antibacterial effect towards Streptococcus mutans. Am J Biotechnol Biochem. 2007;3(1):10–15.
- Yi SM, Zhu J, Fu L, et al. Tea polyphenols inhibit Pseudomonas aeruginosa through damage to the cell membrane. Int J Food Microbiol. 2010;144(1):111–117.