ABSTRACT
A novel strain, Acinetobacter bereziniae strain GWF(KM062029), was isolated from anaerobic activated sludge. The complete 16S rDNA sequence showed that strain GWF has closest homology (100%) with A. bereziniae strain BENAB7 (HE651923.1) and A. bereziniae strain ATCC 17924 (HE651922.1). Strain GWF is a Gram-negative, non-fermentative facultative anaerobe. The optimum growth conditions were shown to be 30 °C, pH 7.5 and acetate-to-perchlorate ratio of 10, respectively. In this study, anthraquinone-1-sulfonate (α-AQS) was the best redox mediator in the perchlorate reduction, and the optimum concentration range was 1.067 mmol/L. The perchlorate reduction rate was enhanced 1.33-fold using 1.067 mmol/L α-AQS. Meanwhile, strain GWF could not withstand the effect of concomitant anions (nitrate, chlorate, chlorite). The concomitant anions constants were 30 mg/L and 1.0 mmol/L for nitrate and chlorate, respectively. Chlorite completely inhibited the perchlorate reduction, and the inhibition did not depend on the concentration of chlorite.
Introduction
Perchlorate is an oxidant or ignitable source, which is widely used in pyrotechnics, roadside flares, air bag inflation systems, solid rocket propellants and missiles.[Citation1,Citation2] Where it occurs, perchlorate ground water contamination varies in the range of a few micrograms per liter to hundreds of milligrams per liter, leading to the termination of water production from highly contaminated wells.[Citation3] Perchlorate is well known to cause adverse health effects on the thyroid gland by interfering with the uptake of iodide, which plays an important role in regulating the metabolic processes of the human body.[Citation4]
The United States Environmental Protection Agency (EPA) estimates that more than 10 million people live in the United States with detectable levels of perchlorate in their drinking water today.[Citation5] In China, perchlorate exists in milk, crops and vegetables at concentrations up to 9.1 ng/mL, 4.88 ng/g dry weight and 30.5 ng/g wet weight, respectively.[Citation6–8] Therefore, perchlorate has been regarded as a new threat to human beings. In 2009, United States EPA released an interim health advisory level of perchlorate at 15 μg/L.[Citation9]
At present, the available technologies for perchlorate remediation generally include physico-chemical and biological technologies. Physico-chemical technologies include membrane reduction processes,[Citation10] activated carbon [Citation11] and ion exchange.[Citation12] Although physical/chemical methods have been improved rapidly, the high costs and the second treatment remain irreparable defects. The regeneration of the resins produces a very high-strength waste brine stream and poses additional disposal problems.[Citation13] Additionally, in this case, environmentally friendly biological technologies can reduce perchlorate to the non-toxic chlorine ion, which is environmentally available, but low reduction rate and long lag period are the bottleneck problem for development of perchlorate biological reduction.
One of the promising technologies for the treatment of wastewater containing a low concentration of perchlorate is ion exchange systems integrated with biological brine treatment. The ion exchange systems can selectively adsorb anions such as perchlorate, nitrate and sulfate. On the other hand, high nitrate and sulfate concentration has also been observed in perchlorate wastewaters. In order to better use microbial agents to reduce perchlorate, it is necessary to (1) isolate and purify an efficient perchlorate reduction bacteria (PRB), (2) investigate the effect of the concomitant anions on the perchlorate reduction.
Recently, there is evidence that biological Cr(VI)-reduction [Citation14] and denitrification [Citation15] processes are dramatically affected by redox mediators; however, few relevant studies report the effect of redox mediators on perchlorate reduction. More investigations should be performed to further elucidate the effect of the redox mediators on the perchlorate reduction process.
In this study, a PRB strain GWF was isolated from mature sewage sludge. Biochemical analysis, 16S rRNA gene sequencing and phylogenetic analysis of strain GWF were also performed. In addition, the effects of temperature, pH, initial acetate-to-perchlorate ratio, five redox mediators of a similar structure and a range of concomitant anions were investigated in batch experiments for perchlorate reduction.
Materials and methods
Chemicals and reagents
The elution solvent for ion chromatography used chromatographic grade chemicals purchased from Guangfu Ltd. (Tianjin, China). Other chemicals and reagents of analytical grade were purchased from Yongda Ltd. (Tianjin, China).
Strain isolation, purification and identification
The seed sludge, which was from the municipal wastewater treatment plant in Shijiazhuang, China, was cultured in a continuous bioreactor. The hydraulic retention time (HRT) was set at 2.2 h and the influent concentration of substrate (perchlorate) was 20 mg/L. After two months of cultivation, the influent concentration of perchlorate was stepwise increased to 200 mg/L. PRB were isolated from the domesticated mature sludge of perchlorate reduction. The basal medium used for cultivation and isolation of PRB contained mineral salt medium supplemented with perchlorate as the electron acceptor and acetate as the electron donor. The composition of the mineral salt medium was as follows: 2.4 g/L of CH3COONa, 0.0585 g/L of MgSO4, 0.1 g/L of K2HPO4, 0.06 g/L of KH2PO4, 0.67 g/L of NH4Cl and trace-metal solution (1 ml/L). The trace-metal solution contained the following compounds: 63.68 mg/L of ethylenediaminetetraacetic acid (EDTA), 2.2 mg/L of ZnSO4, 5.5 mg/L of CaCl2, 4.32 mg/L of MnSO4, 5.0 mg/L of FeSO4·7H2O, 2.11 mg/L of Na2MoO4·2H2O, 1.57 mg/L of CuSO4·5H2O and 1.61 mg/L of CoCl2·6H2O. For solid mineral salt medium, 15 g/L of agar powder was added to the basal medium, which contained 100 mg/L of perchlorate. The initial pH required was approximately 7.5 and the medium was sterilized by autoclaving at 121 °C for 30 min.
The bacterial colonies were plated on solid plates, and colonies were re-grown at 30 °C for 12 h. For the second enrichment method, each bacterial colony sample was inoculated in a 250-mL Erlenmeyer flask containing 200 mL of sterile basal medium. The Erlenmeyer flasks were purged with N2/CO2 (80/20, v/v) for 3 min.[Citation16] The bacterial colony samples were cultured in a shaking incubator at 30 °C and 140 r/min. After 12 h of growth, the initial optical density of cells (OD600nm) was 0.3 ± 0.01. The purification method was repeated until purity was achieved and the isolates were tested for the ability to reduce perchlorate. At the end, four pure cultures of perchlorate-reducing strains (GWF, GWF1, GWF2, GWF3) were isolated. Strain GWF had the highest perchlorate-reduction ability and was selected for further investigation.
Gram-staining and standard biochemical analyses (catalase test, glucose acid production from glucose, gelatin hydrolysis, nitrate reduction, citric acid utilization, starch hydrolysis, oxidase test, mannitol fermentation, arabinose fermentation, Voges–Proskauer (VP) test, methyl red test, urease test, indole test) were performed to identify strain GWF. The pure culture was observed under an optical microscope and a scanning electron microscope after cultivation for 3 d on solid medium.
The 16S rDNA was sequenced by Guangdong Detection Center of Microbiology (Guangzhou, China). The sequences were compared against the GenBank database by using the Blastn and Blastx search tools of the Basic local alignment search tool (BLAST; www.ncbi.nlm.nih.gov/BLAST) for the similarity comparison analysis. A phylogenetic tree based on the 16S rRNA gene sequence analysis was constructed using the neighbour-joining method.
Conditions for perchlorate reduction by strain GWF
Strain GWF was grown at 30 °C in a shaking incubator at 140 r/min by 250-mL Erlenmeyer flasks, which contained 200 mL of sterile basal medium without perchlorate. After 10 h of pre-growth, the initial optical density of cells (OD600nm) was 0.3 ± 0.01.
The perchlorate reduction experiments with strain GWF were conducted in a 300-mL serum bottles with 250 mL of mineral salt medium. The initial concentration of perchlorate in the batch experiments was fixed at 100 mg/L. The bottles were purged with N2/CO2 (80/20, v/v) and were then immediately capped with thick, butyl rubber stoppers and an aluminum crimp seal. Finally, the serum bottles were purged again for 3 min.
Effects of temperature, pH and acetate-to-perchlorate ratio on perchlorate reduction
To analyse the effects of temperature on the perchlorate reduction process, the incubation temperature was varied at 20, 25, 30, 35, 40 and 45 °C. The pH and acetate-to-perchlorate ratio were set at 7.0 and 10:1 (mol-acetate to mol-perchlorate), respectively. Batch experiments were adjusted to pH 6, 6.5, 7, 7.5, 8, 8.5 and 9.0 with 1.0 mol/L HCl and 1.0 mol/L NaOH. The temperature and acetate-to-perchlorate ratio were set at 30 °C and 10:1 (mol-acetate to mol-perchlorate), respectively. The acetate-to-perchlorate ratio was varied: 1:1, 2:1, 4:1, 6:1, 8:1 and 10:1 (mol-acetate to mol-perchlorate), to study the optimum ratio of acetate to perchlorate on the perchlorate reduction. The temperature and pH were set at 30 °C and pH 7, respectively.
Effects of redox mediators on the perchlorate reduction
Anthraquinone-1-sulfonate (α-AQS), anthraquinone-2-sulfonate (AQS), anthraquinone-1,5-disulfonate (1,5-AQDS), anthraquinone-2,6-disulfonate (AQDS) and anthraquinone-2,7-disulfonate (2,7-AQDS) are five typical water-soluble redox mediators [Citation17] that were selected to study the accelerating ability of different redox mediators on the perchlorate reduction. The initial concentrations of redox mediators were fixed at 0.356 mmol/L. Different concentrations of α-AQS were also tested: 0, 0.178, 0.356, 0.533, 0.711, 0.889, 1.067, 1.224, 1.422, 1.600 and 1.778 mmol/L.
Effects of concomitant anions on the perchlorate reduction
The batch tests were also used to study the perchlorate reduction in the presence of concomitant anions (nitrate, chlorate and chlorite). The basal medium was modified by adding solutions of NaNO3, NaClO3 and NaClO2 to obtain various initial concentrations of nitrate (10, 20, 30 mg/L), chlorate (0.2, 0.4, 0.6, 0.8 and 1.0 mmol/L) and chlorite (0.2, 0.4, 0.6, 0.8 and 1.0 mmol/L).
Analytical methods
The concentrations of perchlorate and other anions (nitrate, chlorate, chlorite etc.) were determined by ion chromatography (Metrohm 761, Metrohm AG, Herisau, Switzerland), which was equipped with a Metrosep A Supp 4 column (250 × 4.0 mm), Metrosep A Supp 4/5 S-guard column (50 × 4.0 mm) and a conductivity detector. The elution solvent used was of chromatographic grade chemicals, including sodium carbonate (1.8 mmol/L), sodium bicarbonate (1.7 mmol/L) and acetone (12.5%, v/v). Sulfuric acid (50.0 mmol/L) was used as the regenerant in the chromatographic analysis. Samples from batch experiments were centrifuged at 10,000 × g for 10 min at room temperature and were filtered through a Cleanert C18-SPE and 0.22 µm filter for analysis. The effluent pH was measured using a digital pH meter (Delta-320, China).
Data analysis
Data analysis was performed using the Orgin software. Error bars represent standard errors of the means (±SEM).
Results and discussion
Strain isolation and identification
Four purified bacteria (GWF, GWF1, GWF2 and GWF3) were successfully isolated from domesticated mature sludge in this study. shows the perchlorate reduction capacity of the four bacteria. Nearly 100% of the perchlorate was removed by strain GWF after five days of growth, but only 72.1%, 41.7% and 80.1% were removed by strains GWF1, GWF2 and GWF3, respectively. Thus, strain GWF was chosen as the best strain in the following experiments. Strain GWF was identified to be a Gram-negative bacterium and the results from the biochemical identification analysis are shown in Table S1 in the Online Supplementary Appendix. The colony morphology of strain GWF was translucent oval shape, smooth, convex, moist texture, entire edge, 0.5 mm in diameter. The strain formed round and smooth colonies. The cell size was between 0.4 µm × 0.8 µm and 0.5 µm × 1.4 µm (Online Supplementary Figures S1 and S2).
The 16S rDNA sequence analysis showed sequence homology of the 16S rRNA gene of strain GWF with that of Acinetobacter bereziniae strain BENAB7 (HE651923.1) and Acinetobacter bereziniae strain ATCC 17924 (HE651922.1) reaching 100%. Together with the results from the biochemical identification tests, strain GWF was identified as Acinetobacter bereziniae and was deposited in GenBank under the accession number KM062029. A phylogenetic tree of strain GWF was constructed and is shown in
Effects of temperature, pH and acetate-to-perchlorate ratio on perchlorate reduction
The effect of temperature on the perchlorate reduction process was studied in controlled temperatures in the range of 20–45 °C ((a)). Strain GWF could reduce the perchlorate most rapidly at 30 °C, and perchlorate could be reduced completely after 108 h. The reduction efficiency was 36.72% and 65.06% for 20 °C and 25 °C after 108 h. The metabolic activity of strain GWF was slower at low temperatures. When the temperature increased above 35 °C, the higher the temperature, the lower the rate of perchlorate reduction was. The activity of the perchlorate reductase is likely to be inhibited at high temperatures. The optimum temperature of the stains is 30 °C.[Citation18]
Figure 3. Effect of temperature (a), pH (b) and acetate-to-perchlorate ratio (c) on perchlorate reduction by strain GWF.
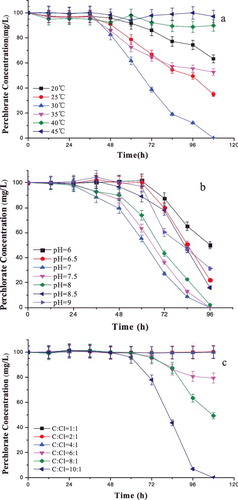
Variations of pH in the environment change the ionic form of acid and base groups in the active sites of an enzyme. To determine the optimal pH for perchlorate reduction by strain GWF, the pH of the basal medium was varied in the range of 6.0–9.0 ((b)). The obtained results showed that strain GWF could substantially reduce perchlorate in the studied pH range. The highest perchlorate reduction rate was obtained at pH 7.0–8.0, followed by those at pH 8.5, 6.5, 9.0 and 6.0. These results are in accordance with previous reports that PRB can grow and reduce perchlorate in the pH range of 5.0–9.0, but with a pH optimum in the narrow range of 6.8–7.5.[Citation19,Citation20] Wu et al. [Citation21] noted the highest rate of perchlorate reduction at pH 7.0–8.0.
The optimal acetate-to-perchlorate ratio is defined as the minimum ratios that will result in complete consumption of both perchlorate and acetate.[Citation22] In our experiments, the tested ratio values ranged from 1:1 to 10:1 (mol-acetate to mol-perchlorate) with initial values of pH 7.5 and perchlorate 100 mg/L ((c)). There was no obvious perchlorate reduction when the initial acetate-to-perchlorate ratio was 1:1, 2:1 and 4:1 after 108 h. When the acetate-to-perchlorate ratio ranged between 6:1 and 10:1, the higher the initial acetate-to-perchlorate ratio was, the higher the perchlorate reduction rate was. A possible explanation may be that the nutrient was sufficient for the microbial growth and perchlorate reduction. This reduction was completed after 108 h. Therefore, the optimal acetate-to-perchlorate ratio obtained was 10:1.
Effects of various redox mediators on perchlorate reduction
Redox mediators could accelerate the electron transfer between an electron donor and an electron acceptor to benefit biological reduction.[Citation14,Citation15,Citation23] The effects of five similarly structured redox mediators on the perchlorate reduction processes are shown in (a) (0.356 mmol/L of α-AQS, AQS, 1,5-AQDS, AQDS and 2,7-AQDS). The perchlorate reduction rate changed from 19.00% (control (blank) sample), to 100% (α-AQS), 54.88% (AQS), 74.10% (1,5-AQDS), 77.46% (AQDS) and 54.78% (2,7-AQDS), after 72 h of culture. This result indicated that α-AQS showed the best catalytic effect on the perchlorate reduction.
Figure 4. Effect of various redox mediators (a) and of different concentrations of α-AQS (b) on perchlorate reduction by strain GWF.
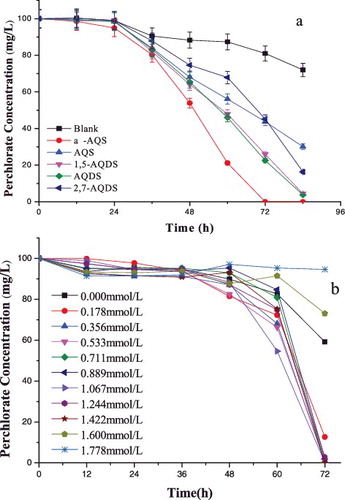
The biocatalytic perchlorate reduction process was also conducted at different α-AQS concentrations ranging from 0 to 1.778 mmol/L. The results ((b)) showed that α-AQS could substantially accelerate the perchlorate reduction process in its concentration range of 0.178–1.422 mmol/L. There was almost no catalytic acceleration at 1.600 mmol/L α-AQS and 1.778 mmol/L α-AQS. This phenomenon may be due to the high concentration of quinone, which may cause toxic effects to the microorganisms themselves [Citation24] and the perchlorate reduction.
Cervantes [Citation25] reported that quinone compounds could accelerate the electron transfer from the primary electron donor to the final electron acceptor, which could lead to an increase in the reduction rate from one to several orders of magnitude. The mechanism could be that (1) biological anaerobic reduction processes need co-enzymes; quinone compounds and some co-enzymes have similar structural characteristics; thus quinone compounds could play a similar role to that of a co-enzyme in the reduction process; (2) under the action of enzymes, quinone compounds could change into a highly active and stable intermediate; this intermediate could transfer a hydrogen proton or electron from the substrate to the final electron acceptor. The acceleration mechanism by α-AQS may be a combination of both. For example, Xi et al. [Citation17] reported that quinones not only have similar structural characteristics to methyl naphthoquinone, which is an important coenzyme, but also function as electron carriers that transfer the electron from the substrate to the nitrate in the denitrification process.
Effects of nitrate, chlorate and chlorite on perchlorate reduction
Nitrate, chlorate and chlorite are often found as concomitant pollutants in perchlorate wastewater from fireworks, electroplating, electro-polishing, army ammunitions and other industries.[Citation26–28] That is why, as a next step in our experiments, we studied the effects of these anions on the perchlorate reduction by strain GWF.
The effect of nitrate on the perchlorate reduction was operated under concentrations of nitrate of 0.16, 0.32 and 0.48 mmol/L ((a)). The perchlorate reduction rate was not affected by a lower concentration of nitrate (0.16 mmol/L) but was completely inhibited when the nitrate concentration was 30 mg/L. This result is consistent with previous reports that perchlorate reduction is inhibited by nitrate, which could be attributed mainly to the suppression of perchlorate reductase by nitrate.[Citation29] However, the result also suggests that perchlorate and nitrate probably had a synergistic effect on the perchlorate reduction when the nitrate concentration was less than that of perchlorate and that a small amount of nitrate may be beneficial to promote the activity of perchlorate reductase.
Figure 5. Effect of different concomitant anions: nitrate (a), chlorate (b) and chlorite (c); on perchlorate reduction by strain GWF.
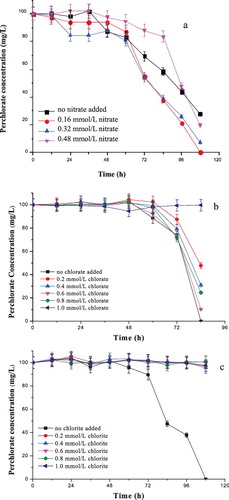
The effect of chlorate in the concentration range from 0.2 mmol/L to 1.0 mmol/L on reduction of perchlorate by strain GWF is shown in (b). Strain GWF was able to reduce perchlorate completely in the absence of chlorate after 84 h, while the other conditions led to reduction of up to 52.3%, 69.0%, 89.8%, 75.7% and 0.3% (after 84 h of culture). These results indicated that the reduction of perchlorate to chlorate was the rate-determining step in the biological perchlorate reduction by strain GWF. This could also indicate that a certain concentration of chlorate may affect the activity of perchlorate reductase for the reduction of perchlorate and chlorate.[Citation30]
(c) shows that chlorite had a lesser effect on the perchlorate reduction by strain GWF. The perchlorate in the control (blank) sample (without chlorite) was completely reduced after 108 h. However, chlorite severely inhibited the perchlorate reduction and, and the inhibition did not depend on the concentration of chlorite. This could suggest that chlorite had a toxic effect on strain GWF and inhibited the activity of perchlorate reductase.[Citation19]
Thus, the results from our study indicate that strain CWF could be considered promising for reduction of perchlorate in wastewaters that do not contain chlorite. Further research needs to focus on a larger-scale process in bioreactors.
Conclusions
A. bereziniae strain GWF, a novel perchlorate-reducing strain, was isolated. At optimum conditions (30 °C, 7.5 and a 10:1 acetate-to-perchlorate ratio), the strain could reduce 100 mg/L in 120 hours. The perchlorate reduction rate was enhanced 1.33-fold by 1.067 mmol/L α-AQS. The process of perchlorate reduction by strain GWF was significantly inhibited by nitrate, chlorate and chlorite. These results provide evidence that the A. bereziniae strain GWF is a promising bacterium for perchlorate reduction. The optimal parameters of the perchlorate reduction process can be used in further research.
Sup_mat_Isolation_and_characterization_of_a_Jianbo.doc
Download MS Word (5.8 MB)Acknowledgments
The authors are very grateful to the editors and reviewers for their constructive comments on this paper.
Disclosure statement
No potential conflict of interest was reported by the authors.
Additional information
Funding
References
- Wang Z, Gao M, Zhang Y, et al. Perchlorate reduction by hydrogen autotrophic bacteria in a bioelectrochemical reactor. J Environ Manage. 2014;142:10–16.
- Ye L, You H, Yao J, et al. Water treatment technologies for perchlorate: a review. Desalination. 2012;298:1–12.
- Fox S, Oren Y, Ronen Z, et al. Ion exchange membrane bioreactor for treating groundwater contaminated with high perchlorate concentrations. J Hazard Mater. 2014;264:552–559.
- Brent GA. The impact of perchlorate exposure in early pregnancy: is it safe to drink the water? J Clin Endocrinol Metab. 2013;95:3154–3157.
- Sharbatmaleki M, Unz RF, Batista JR. Potential mechanisms for bioregeneration of perchlorate-containing ion-exchange resin. Water Res. 2015;75:1–10.
- Ye L, You H, Yao J, et al. Seasonal variation and factors influencing perchlorate in water, snow, soil and corns in Northeastern China. Chemosphere. 2013;90:2493–2498.
- Shi Y, Zhang P, Wang Y, et al. Perchlorate in sewage sludge, rice, bottled water and milk collected from different areas in China. Environ Int. 2007;33:955–962.
- Gan Z, Pi L, Li Y, et al. Occurrence and exposure evaluation of perchlorate in indoor dust and diverse food from Chengdu, China. Sci Total Environ. 2015;536:288–294.
- Xu X, Gao B, Jin B, et al. Study of microbial perchlorate reduction: considering of multiple pH, electron acceptors and donors. J Hazard Mater. 2015;285:228–235.
- Park SH, Batchelor B, Lee C, et al. Perchlorate degradation using a titanium and membrane hybrid (TMH) system: transport, adsorption, chemical reduction. J Membrane Sci. 2012;390–391:84–92.
- Parette R, Cannon FS. Perchlorate removal by modified activated carbon. New York: Springer; 2006.
- Lehman SG, Badruzzaman M, Adham S, et al. Perchlorate and nitrate treatment by ion exchange integrated with biological brine treatment. Water Res. 2008;42:969–976.
- Lin Y, Fang Q, Chen B. Perchlorate uptake and molecular mechanisms by magnesium/aluminum carbonate layered double hydroxides and the calcined layered double hydroxides. Chem Eng J. 2014;237:38–46.
- Guo J, Lian J, Xu Z, et al. Reduction of Cr(VI) by Escherichia coli BL21 in the presence of redox mediators. Bioresour Technol. 2012;123:713–716.
- Guo J, Kang L, Yang J, et al. Study on a novel non-dissolved redox mediator catalyzing biological denitrification (RMBDN) technology. Bioresour Technol. 2010;101:1867–1878.
- Cang Y, Roberts DJ, Clifford DA. Development of cultures capable of reducing perchlorate and nitrate in high salt solutions. Water Res. 2004;38:3322–3330.
- Xi Z, Guo J, Lian J, et al. Study the catalyzing mechanism of dissolved redox mediators on bio-denitrification by metabolic inhibitors. Bioresour Technol. 2013;140:22–27.
- Dahl R. Drinking water: NAS Reports on perchlorate safety. Environ Health Perspect. 2005;113:A449.
- Coates J, Achenbach LA. Microbial perchlorate reduction: rocket-fueled metabolism. Nat Rev Microbiol. 2004;2:569–580.
- Wang C, Lippincott L, Meng X. Kinetics of biological perchlorate reduction and pH effect. J Hazard Mater. 2008;153:663–669.
- Wu D, He P, Xu X, et al. The effect of various reaction parameters on bioremediation of perchlorate-contaminated water. J Hazard Mater. 2008;150:419–423.
- Shrout JD, Parkin GF. Influence of electron donor, oxygen, and redox potential on bacterial perchlorate degradation. Water Res. 2006;40:1191–1199.
- Thrash JC, Van Trump JI, Weber KA, et al. Electrochemical stimulation of microbial perchlorate reduction. Environ Sci Technol. 2007;41:1740–1746.
- Jiao L, Lu H, Zhou J. Quinone-mediated decolorization of sulfonated azo dyes by cells and cell extracts from Sphingomonas xenophaga. J Environ Sci En. 2009;21:503–508.
- Cervantes FJ. Quinones as electron acceptors and redox mediators for the anaerobic biotransformation of priority pollutants [dissertation]. Wageningen: Wageningen University; 2002.
- Hackenthal E, Mannheim W, Hackenthal R, et al. The reduction of bacteria by perchlorate, studies on intact cells. Biochem Pharmacol. 1964;14:195–206.
- Dugan NR, Williams DJ, Meyer M, et al. The impact of temperature on the performance of anaerobic biological treatment of perchlorate in drinking water. Water Res. 2009;43:1867–1878.
- Ghosh A, Pakshirajan K, Ghosh PK, et al. Perchlorate degradation using an indigenous microbial consortium predominantly Burkholderia sp. J Hazard Mater. 2011;187:133–139.
- Nerenberg R, Kawagoshi Y, Rittmann BE. Microbial ecology of a perchlorate-reducing, hydrogen-based membrane biofilm reactor. Water Res. 2008;42:1151–1159.
- Logan BE. A review of chlorate and perchlorate respiring microorganisms. Bioremed J. 1998;2:69–79.