ABSTRACT
Durum wheat (Triticum turgidum var. durum) is one of the most important cereal crops widely cultivated all over the world with high economic value. In the present study, genetic variation in a mini-core collection of durum wheat germplasm, including 25 breeding lines and 18 landraces, was evaluated using 15 inter-simple sequence repeat (ISSR) and six start codon targeted (SCoT) markers. High levels of polymorphism were observed; 98.70% (ISSR) and 100% (SCoT), which indicated that these markers are useful tools for detection of genetic variation in the collection. Analysis of molecular variance revealed that the major part of genetic variations (90% and 93% for ISSR and SCoT, respectively) occurred within genotypes set. Comparing the genetic variation of breeding lines and landraces based on genetic parameters showed that effective number of alleles (Ne), Nei's gene diversity (He) and Shannon's Information index (I) in landraces were higher than in breeding lines. Although cluster analysis, based on both markers, separated the genotypes in five groups, the dendrogram obtained from SCoT provided the best clustering pattern. Inter-population differentiation (Gst) estimated on the basis of two marker systems representing that a vast portion of the total genetic diversity refers to variation within two sets of genotypes. In conclusion, the results verified a high level of genetic variation among the durum wheat mini-core collection, particularly among landraces, which can be interesting for future breeding programmes.
Introduction
Wheat (common and durum) is an important cereal crop used for human consumption worldwide. Durum wheat (Triticum turgidum L. subsp. durum), as one of the major cereal crops, is the only tetraploid (AABB, 2n = 4x = 28) species of commercial wheat that is widely cultivated in Mediterranean climates [Citation1,Citation2]. By 2020, the world need for wheat will be 40% greater than in the present day. In response to this challenge, breeders must increase the yield and simultaneously reduce the impact of agriculture on the environment.
The evaluation of genetic diversity in the present collection of germplasm is one of the main tasks of breeding programmes, because it may help in the selection of cultivars and lines with higher diversity and better performance under specific conditions. Molecular markers provide valuable information in crop breeding, especially in studies on genetic diversity and genetic relationships between different crop species. In particular, the polymerase chain reaction (PCR) is used to analyse the amplification fragment length polymorphism (AFLP), random amplified polymorphic DNA (), simple sequence repeats (SSR), and inter-simple sequence repeats (ISSR). ISSR marker is detected by means of repeaters’ anchored primers that are amplified between SSRs, and has also been used to analyse the genetic diversity among different species of plants. Furthermore, this technique, due to high repeatability and polymorphism, as well as highly informative, is suitable for assessing genetic diversity in different crops [Citation3,Citation4]. In recent years, many new alternative and capable marker techniques have been developed. Start codon targeted (SCoT) polymorphisms are reproducible markers that are based on the short conserved region in plant genes surrounding the ATG translation start (or initiation) codon [Citation5]. SCoT markers have been successfully used to evaluate genetic diversity and structure, identify cultivars, and for quantitative trait loci (QTL) mapping and DNA fingerprinting in different species, including wheat, rice, check pea, sugarcane and grape [Citation5–9].
Genetic erosion in cultivated wheat provides a good incentive for assessing genetic diversity among its relatives [Citation4], as well as determines the potential for improving efficiency of plant materials and hence their use for breeding, which eventually may result in improved food production [Citation10]. In this study, we used a mini-core collection of durum wheat germplasm. Thus, the main goal of the present study was to determine the genetic variability using ISSR and SCoT markers.
Materials and methods
Plant material and DNA isolation
A total of 43 durum wheat germplasm including 25 breeding lines (originated from ICARDA, Syria) and 18 landraces (collected from different regions of Iran) were considered in the current work (). From each genotype, after the seed germination and growth, the total genomic DNA was isolated from young leaves of greenhouse-grown plants according to the CTAB protocol [Citation11].
Table 1. Name, pedigree and origin of 43 durum wheat landraces and breeding lines.
ISSR–PCR amplification
After optimizing PCR, 15 ISSR primers were selected from the set of Biotechnology Laboratory, University of British Columbia, Canada (). The PCRs were performed in the reaction mixture 20 µL volume, with 2 µL of isolated DNA from each sample, 1.2 µL of each primer, 0.4 µL dNTPs mix, 1.5 µL MgCl2, 5 units of Taq polymerase (0.3 µL), 2 µL PCR buffer and 12.6 µL ddH2O (double-distilled water).Amplification was run at 94 ºC for 4 min, followed by 30 cycles of denaturation at 94 ºC for 30 sec, primer annealing at 34–45 ºC (varied for each primer) for 45 sec and primer elongation at 72 ºC for 2 min. The final extension was 7 min at 72 ºC. The amplification reaction products were detected by 1.5% denaturing agarose gels stained with safe view ΙΙ.
Table 2. ISSR and SCoT primers and their amplification results generated in the 43 durum wheat germplasm.
SCoT–PCR amplification
A total of six SCoT primers were randomly selected (). The PCRs were performed in a reaction mixture with a volume of 20 µL, with 10 µL master mix 2XPCR (ready-to-use PCR master mix 2X), 6 µL ddH2O, 2 µL of isolated DNA from each sample and 2 µL of each primer. Amplification was run at 94 ºC for 5 min, followed by 45 cycles of denaturation at 94 ºC for 45 sec, primer annealing at 45 ºC for 45 sec and primer elongation at 72 ºC for 90 sec. The final extension was 10 min at 72 ºC. The amplification reaction products were detected by 1.5% denaturing agarose gels stained with safe view ΙΙ.
Data analysis
In order to measure the informativeness of the markers to differentiate between genotypes, polymorphism information content (PIC), marker index (MI) and resolving power (Rp) were calculated. PIC was calculated according to the formula of Anderson et al. [Citation12], as PIC = 1 − ∑pi 2, where pi is the frequency of the ith allele of the locus in 43 genotypes. MI was determined according to Varshney et al. [Citation13] as the product of PIC and effective multiplex ratio. The Rp of each primer was calculated using the formula Rp = ∑Ib , where Ib is band informativeness (the Ib can be represented on a scale of 0–1 by the following formula: Ib = 1 − [2 × (0.5 − p)], where p is the proportion of individual containing the band) [Citation14].
The distribution of genetic variation within and among genotypes (analysis of molecular variance [AMOVA]) was estimated using GenAlEx software [Citation15]. To characterize genetic variation, some parameters, namely Shannon's information index (I), Nei's gene diversity (H) and the observed number of alleles (Na), the total gene diversity (Ht), effective number of alleles (Ne), gene flow (Nm) and inter-population differentiation (Gst) were calculated using PopGen 1.31 software [Citation16]. Cluster analysis based on Jaccard's similarity coefficient matrix was constructed using NTSYS-pc software [Citation17].
Results and discussion
Germplasm evaluation helps in the identification of diverse genotypes that can be utilized by breeding for creating the desired variation. Morphological characters may be affected by environmental conditions. Therefore, the use of morphological characters for classification may result in discrepancies [Citation18].
ISSR and SCoT analysis
Fifteen ISSR primers were screened to investigate the genetic variation between and within mini collection of durum wheat germplasm. A total of 155 bands were produced (), out of which 149 (96.13%) were polymorphic bands. The number of polymorphic bands ranged from 7 (ISSR-9) to 15 (ISSR-25) with a mean of 10.2. The PIC values varied from 0.14 (ISSR-11) to 0.49 (ISSR-3, ISSR-5, ISSR-23 and ISSR-25) with an average of 0.41. Resolving power parameter is described by Prevost and Wilkinson [Citation14] as a degree of the discriminatory power of ISSR molecular markers. The Rp value of the 15 primers varied from 10.04 (ISSR-23) to 21.34 (ISSR-25) distinguishing the different genotypes. The highest value of MI was observed for ISSR-25 (6.15), while the lowest value related to ISSR-11 (1.28).
SCoT analysis
A total of 54 bands were generated from six SCoT primers (), and all of them were polymorphic bands. The total bands per primer ranged from 6 (SCoT-21) to 12 (SCoT-4). The PIC values varied from 0.21 (SCoT-21) to 0.50 (SCoT-4) with an average of 0.39. The Rp value of the six primers varied between 4.37 (SCoT-12) to 13.76 (SCoT-11) discriminating the different genotypes. The highest and lowest values of MI were observed for SCoT-14 (43.49) and SCoT-14 (3.01), respectively.
ISSR and SCoT markers have been proved to be useful in genetic diversity studies because of their high reproducibility and great power for the detection of polymorphism [Citation7,Citation8,Citation19–Citation21]. The results showed that there is a high genetic variation among tested genotypes, that is in agreement with the results from previous studies carried out on durum wheat germplasm [Citation22,Citation23]. In the present study, the efficiency of both ISSR and SCoT markers is estimated through parameters such as PIC, MI and Rp. Parameters such as PIC have been used usually for evaluating the informative potential of ISSR markers in different germplasm and cultivated genotypes [Citation24–27]. Based on the results of data analysis, the moderate values of PIC for both marker primers could be attributed to evaluation of genetic diversity. Also, in this work, the average PIC value per primer is comparable to that found by Zamanifard et al. [Citation23] using ISSR, and by Hamidi et al. [Citation8] using SCoT markers in wheat germplasm. The MI, which can be considered to be a common measure of efficiency in discovering polymorphism [Citation28], was different in two marker systems (). On the other hand, an important property of a suitable marker system is the capacity to distinguish among different accessions. Our study revealed that the resolving power of ISSR primers is higher than SCoT primers. Consequently, these results are in agreement with previous studies [Citation14,Citation28], in which MI and PR can be proposed as most marker parameters for selecting informative primers. Thus primer ISSR-25 with (CA)8RG motif and SCoT-4 was identified as the best primer for distinguishing the 43 durum wheat germplasm tested.
Genetic variation revealed by ISSR and SCoT markers
The results of AMOVA analysis demonstrated that the major part of genetic variations occurred within genotypes set: 90% based on the ISSR markers and 93% on the basis of SCoT markers (). Thus, this result indicated that the estimated variance within genotypes was greater among them. A summary of genetic diversity indices in two different sets of genotypes is shown in . For ISSR markers, the Na value varied from 1.78 ± 0.41 (landraces) to 1.87 ± 0.32 (breeding lines). The Ne value was invariably less than the Na value for each set, presenting a distinction in the range of 1.52 ± 0.33 (in breeding lines set) to 1.55 ± 0.38 (in landraces set).The highest and the lowest Nei's gene diversity (H) related to landraces (0.33 ± 0.19) and breeding lines (0.30±0.16) set, respectively. The maximum (0.44 ± 0.27) and the minimum (0.41 ± 0.22) Shannon's information index (I) also observed for landraces and breeding lines set, respectively. In contrary, the results obtained by SCoT primers were different. So, the maximum and minimum Na values were for breeding lines (1.92 ± 0.232) and landraces (1.92 ± 0.26), respectively. Ne ranged from 1.55 ± 0.30 (in breeding lines set) to 1.57 ± 0.32 (in landraces set). The highest and lowest H belonged to landraces (0.33 ± 0.15) and breeding lines (0.32 ± 0.14) set, respectively. In both breeding lines and landraces genotypes sets, the same Shannon's information index (I) was observed. Previously, the high level of genetic variation in durum wheat had been reported by Mardi et al. [Citation22] and El-Assal and Gaber [Citation10]. In addition, Gst determined on the basis of the ISSR and SCoT markers was 0.07 and 0.05 respectively, indicating that a little amount of the total genetic variability (7% and 5% for ISSR and SCoT, respectively) was among two sets of materials, and 93% (ISSR) and 95% (SCoT) of the total genetic variability were within two sets of genotypes. Also, the Nm value between two sets of genotypes was 6.51 for ISSR and 9.53 for SCoT markers.
Table 3. Analysis of molecular variance (AMOVA) based on ISSR and SCoT markers for two sets of durum wheat genotypes.
Table 4. Summary of genetic variations among two sets of durum wheat genotypes as revealed through ISSR and SCoT analyses.
In order to investigate, genetic relationships among breeding lines and landrace genotypes cluster analysis based on Jaccard's similarity coefficients and UPGMA algorithm were calculated for the 43 durum wheat germplasm. According to the clustering pattern obtained by ISSR () and SCoT () data, the 43 durum wheat classified into five groups. Based on ISSR dendrogram, the first group consists of 14 landraces (LR-26, LR-27, LR-28, LR-30, LR-32, LR-33, LR-35, LR-36, LR-37, LR-38, L-39, LR-41, L-42 and LR-43) along with 8 breeding lines (BL-1, BL-2, BL-3, BL-4, BL-5, BL-6 and BL-8). Eleven breeding lines (BL-10, BL-12, BL-13, BL-14, BL-15, BL-16, BL-18, BL-19, BL-20, BL-21 and BL-24) were classified in a separate group (the second group). The third and fourth groups consist of one (LR-34) and three genotypes (BL-7, LR-31 and LR-40), respectively. Finally, five breeding lines (BL-9, BL-17, BL-22, BL-23 and BL-25) along with one landrace (LR-29) were distinguished as the fifth group.
Figure 1. Dendrogram of 43 durum wheat germplasm resulting from the UPGMA cluster analysis based on Jaccard's similarity coefficients obtained from ISSR marker.
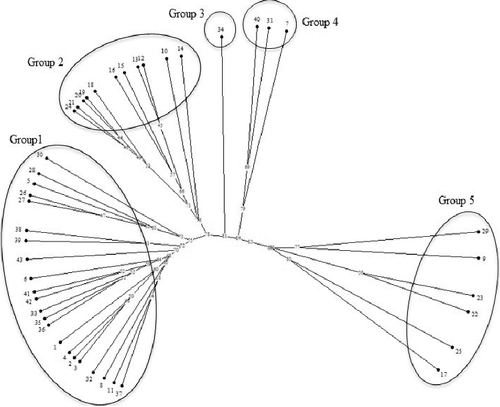
Figure 2. Dendrogram of 43 durum wheat germplasm resulting from the UPGMA cluster analysis based on Jaccard's similarity coefficients obtained from SCoT marker.
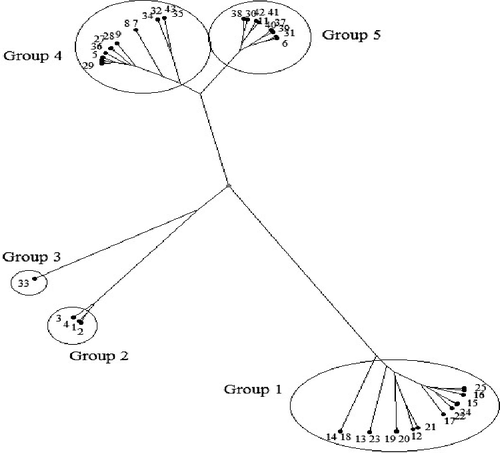
According to SCoT dendrogram, 14 breeding lines were placed in the first group (BL-13, BL-14, BL-15, BL-16, BL-17, BL-18, BL-19, BL-20, BL-21, BL-22, BL-23, BL-24 and BL-25). The second group consists of four breeding lines (BL-1, BL-2, BL-3, and BL-4). Landrace No. 33 was also separated as the third group. Eight landraces (LR-27-, LR-28, LR-29, LR-32, LR-34, LR-35, LR-36 and LR-43) as well as three breeding lines (BL-5, BL-7 and BL-8) were clustered in the fourth group. The rest of landraces along with breeding line No. 6 were placed into the fifth group.
In general, although there are no significant differences between these markers in terms of evaluating and distinguishing of genotypes, the dendrogram obtained from SCoT showed the best clustering pattern. More importantly, SCoT marker is generated from the functional region of the genome; the genetic analyses using this marker would be more useful for crop improvement programmes, such as genotype identification, considering genetic diversity, construction of linkage maps and QTL mapping.
Conclusion
Information about the degree and distribution of genetic variation and relationships among breeding materials has a significant effect on crop improvement. In the present study, SCoT marker, similar to ISSR marker, was an efficient technique to estimate the genetic variation. Additionally, the large polymorphic fragment percentage and number of polymorphic bands obtained in our study indicate the power of SCoT marker in fingerprinting and diversity analyses. Also, our results revealed a high genetic variation among tested genotypes, which can be used in rain-fed durum wheat breeding programmes. Thus should focus on collecting more landrace collections, as well as obtaining more genetic information for the purpose of improvement and development of new cultivars.
Acknowledgments
The authors are grateful to Kermanshah Branch, Islamic Azad University for hosting the lab facilities. Also, the authors acknowledge and appreciate the comments of anonymous reviewers and technical editors of the Journal of Biotechnology & Biotechnological Equipment.
Disclosure statement
No potential conflict of interest was reported by the authors.
References
- Poursiahbidi M , Pour-Aboughadareh A , Tahmasebi G , et al. Evaluation of genetic diversity and interrelationships of agro-morphological characters in durum wheat (Triticum durum desf.) lines using multivariate analysis. IJARR. 2013;3:184–194.
- Tian Y , Xing C , Cao Y , et al. Evaluation of genetic diversity on Prunus mira Koehne by using ISSR and RAPD markers. Biotechnol Biotechnol Equip. 2015;29:1053–1061.
- Bornet B , Branchard M . Nonanchored. inter simple sequence repeat (ISSR) markers: reproducible and specific tools for genome fingerprinting. Plant Mol Biol Rep. 2001;19:209–215.
- Moradkhani H , Pour-Aboughadareh AR , Mehrabi AA , et al. Evaluation of genetic relationships of Triticum-Aegilops species possessing D genome in different ploidy levels using microsatellites. Int J Agri Crop Sci. 2012;23:1746–1751.
- Collard BC , Mackill DJ . Start codon targeted (SCoT) polymorphism: a simple, novel DNA marker technique for generating gene-targeted markers in plants. Plan Mol Biol Rep. 2009;27:86–93.
- Amirmoradi B , Talebi R , Karami E .Comparison of genetic variation and differentiation among annual Cicer species using start codon targeted (SCoT) polymorphism, DAMD-PCR, and ISSR markers. Plant Syst Evol. 2012;298:1679–1688.
- Guo DL , Zhang JY , Liu CH . Genetic diversity in some grape varieties revealed by SCoT analyses. Mol Biol Rep. 2012;39:5307–5313.
- Hamidi H , Talebi R , Keshavarzi F . Comparative efficiency of functional gene-based markers, start codon targeted polymorphism (SCoT) and conserved DNA-derived polymorphism (CDDP) with ISSR markers for diagnostic fingerprinting in wheat (Triticum aestivum L.). Cereal Res Commun. 2014;42(4):558–567.
- Que YX , Pan YB , Lu YH , et al. Genetic analysis of diversity within a Chinese local sugarcane germplasm based on start codon targeted polymorphism. Bio Med Res Int. 2014;2014:1–10.
- El-Assal S , Gaber, A . Discrimination capacity of RAPD, ISSR AND SSR markers and their effectiveness in establishing genetic relationship and diversity among Egyptian and Saudi wheat cultivars. Am J Appl Sci. 2012;9:724–735.
- Doyle JJ , Doyle JL . A rapid DNA isolation procedure for small quantities of fresh leaf tissue. Phytochem Bull. 1987;19:11–15.
- Anderson JA , Churchill GA , Autrique JE , et al. Optimizing parental selection for genetic linkage maps. Genome. 1993;36:181–186.
- Varshney RK , Chabane K , Hendre PS , et al. Comparative assessment of EST-SSR, EST-SNP and AFLP markers for evaluation of genetic diversity and conservation of genetic resources using wild, cultivated and elite barleys. Plant Sci. 2007;173:638–649.
- Prevost A , Wilkinson MJ . A new system of comparing PCR primers applied to ISSR fingerprinting of potato cultivars. Theor Appl Genetic. 1999;98:107–112.
- Peakall R , Smouse PE . GENALEX 6: genetic analysis in Excel. Population genetic software for teaching and research. Mol Ecol. 2006;6:288–295.
- Yeh FC , Yang RC , Boyle T . Microsoft Window-based freeware for population genetic analysis (POPGENE). Version 1.31. 1999. Available from: http://ftp.microsoft.com/softlib/MSLFILES/HPGL.EXE.
- Rholf F . Numerical taxonomy and multivariate analysis system: version 2.10. Setauket ( NY ): Exeter Software; 2007.
- Abdel Kalil K , Abd El-Twan M , Galal R . Genetic diversity and relationships among Egyptian Galium (Rubiaceae) and related species using ISSR and RAPD markers. Biologia. 2014;69:300–310.
- Cao PJ , Yao QF , Ding BY , et al. Genetic diversity of Sinojackia dolichocarpa (Styracaceae), a species endangered and endemic to China, detected by inter-simple sequence repeat (ISSR). Biochem Syst Ecol. 2006;34:231–239.
- Galvan MZ , Bornet B , Balatti PA , et al. Inter simple sequence repeat (ISSR) marker as a tool for the assessment of both genetic diversity and gene pool origin in common bean (Phaseolus vulgaris L.). Euphytica. 2003;132:297–301.
- Sofalian O , Chaparzadeh N , Dolati M . Genetic diversity in spring wheat landraces from northwest of Iran assessed by ISSR markers. Not Bot Horti Agrobot Cluj Napoca. 2009;37:252–256.
- Mardi M , Naghavi MR , Pirseyedi SM , et al. Comparative assessment of SSAP, AFLP and SSR markers for evaluation of genetic diversity of durum wheat (Triticum turgidum L. var. durum). J Agr Sci Tech. 2011;13:905–920.
- Zamanianfard Z , Alireza Etminan A , Mohammadi R , et al. Evaluation of molecular diversity of durum wheat genotypes using ISSR markers. Biol Forum. 2015;7:214–218.
- Gomes S , Martins-Lopes P , Lopes J , et al. Assessing genetic diversity in Oleaeuropaea L. using ISSR and SSR markers. Plant Mol Biol Rep. 2009;27:365–373.
- Grativol C , da Fonseca C , Medeiros L , et al. High efficiency and reliability of inter-simple sequence repeats (ISSR) markers for evaluation of genetic diversity in Brazilian cultivated Jatropha curcas L. accessions. Mol Biol Rep 2011;38:4245–4256.
- Patra B , Acharya L , Mukherjee AK , et al. Molecular characterization of ten cultivars of Canna lilies (Cannalinn.) using PCR based molecular markers (RAPDs and ISSRs). Int J Integr Biol. 2008;2:129–137.
- Tatikonda L , Wani SP , Kannan S , et al. AFLP-based molecular characterization of an elite germplasm collection of Jatrophacurcas L. a biofuel plant. Plant Sci. 2009;176:505–513.
- Khodadadi M , Fotokian MH , Miransari M . Genetic diversity of wheat (Triticum aestivum L.) genotypes based on cluster and principal component analyses for breeding strategies. Aust J Crop Sci. 2011;5:17–24.