ABSTRACT
The production of cellulases in plants is an economical method for the conversion of lignocellulosic biomass into fuels. Herein we report the expressions of two thermostable Acidothermus cellulolyticus cellulases, endo-1,4-β-D-glucanase (E1) and exoglucanase (Gux1), in tobacco and rice. To evaluate the expression of these recombinant cellulases, we expressed the full-length E1, the catalytic domains of E1 (E1cd) and Gux1 (Gux1cd), as well as an E1–Gux1cd fusion enzyme in various subcellular compartments. In the case of tobacco, transgenic plants that expressed apoplast-localized E1 showed the highest level of activity, about three times higher than those that expressed the cytosolic E1. In the case of rice, the level of cellulase-specific activity in the transgenic plants ranged from 11 to 20 nmol 4-methylumbelliferone min−1 mg−1 total soluble protein. The recombinant cellulases exhibited good thermostability below 70 °C. Furthermore, transgenic rice leaves that were stored at room temperature for a month lost about 20% of the initial cellulase activity. Taken together, the results suggested that heterologous expression of thermostable cellulases in plants may be a viable option for biomass conversion.
Introduction
Lignocellulosic biomass is an abundant and renewable source of carbon available for the bioenergy industry. The utilization of plant biomass via its conversion to glucose would not contribute to global CO2 increase [Citation1]. Cellulosic materials from plant cell walls can be converted into glucose, which can then be fermented into ethanol, one of the important biofuels that is currently being produced as an alternative energy source. The conversion of cellulosic materials to glucose can be achieved by degradation techniques that usually involve expensive enzymatic hydrolysis processes, which may result in environmental pollution [Citation2]. However, by using plants to produce the enzymes necessary for breaking down cellulosic materials, the conversion can be directly carried out without the need to add exogenous enzymes. Thus, converting the plants into green bioreactors for large-scale production of cellulases would be highly desirable, both in terms of economic benefits and environmental protection [Citation3].
The efficient conversion of cellulose to glucose requires the synergistic reaction of endo-1,4-β-glucanase (EC 3.2.1.4), exo-1,4-β-glucosidase (EC 3.2.1.91) and β-D-glucosidase (EC 3.2.1.21) [Citation4]. The cost of enzymes comprises a large percentage of the cost of cellulose-to-ethanol conversion. Traditional approaches have mainly focused on engineering recombinant microbial strains to perform both cellulose hydrolysis and fermentation to high-value products [Citation5–7]. A novel way to reduce the cost is to produce various cellulases within the plants that will be used as a source of biomass [Citation8]. Another way to further lower the cost is to increase the catalytic efficiency of individual enzymes and use a mixture of enzymes [Citation9]. Expression of mesophilic cellulases in crops would damage the host plants due to cell-wall degradation at normal growth temperatures, leading to reduced growth rates compared to their non-transgenic counterparts [Citation8]. However, the expression of thermophilic cellulases would cause little damage to the host plants, since these enzymes normally do not exhibit full activity unless the temperature is raised to the point that coincides with the optimum temperatures of the enzymes, which are usually above the normal growth temperatures of most plants [Citation10].
Acidothermus cellulolyticus belongs to the Gram-positive actinobacterial group and its DNA has a high G + C content. It is an acid-tolerant (optimal pH of 5.5) and a thermophilic bacterium (optimal growth temperature of 55 °C). A. cellulolyticus endoglucanase E1 and exoglucanase Gux1 both belong to the same family of glucoside hydrolases and are considered to have great potential in the biofuels industry because of their thermostability and activity at low pH [Citation11].
The present study investigated the expression of E1, E1cd and Gux1cd cellulases and an E1–Gux1cd fusion form in tobacco and rice. The recombinant genes were driven by the cauliflower mosaic virus (CaMV) 35S promoter or the double CaMV 35S promoter and the 5′ untranslated region (UTR) from tobacco etch potyvirus (TEV), both of which have been shown to enhance the transcription of transgenes in plants [Citation12]. The effect of subcellular expression on enzyme accumulation, activity and storage stability were also analysed.
Materials and methods
Strains, plant material and growth conditions
A. cellulolyticus was purchased from the American Type Culture Collection (ATCC 43068) and was cultured at 55 °C in low-phosphate basal salts medium. Agrobacterium tumefacines EHA105 was used in the transformation of tobacco and rice plants. The growth and maintenance procedures for transgenic tobacco and rice were performed as previously described [Citation4].
Construction of expression constructs harbouring E1, E1cd and Gux1cd
The schematic representation of different cellulase expression cassettes is shown in . E1, E1cd and Gux1cd sequences were amplified from the genomic DNA of A. cellulolyticus. Restriction sites for XbaI and SacI were introduced, respectively, at the 5′ and 3′ termini of the amplified DNA fragments. The amplified E1 fragment was inserted into the pBIDST vector at a site downstream of the double 35S promoter and the 5′-UTR from TEV, yielding the construct pBIDST-E1. The amplified E1 fragment was also ligated to the 3′-end of the KOZAK-CALSP (tobacco calreticulin signal peptide) fragment, which was previously prepared in our laboratory [Citation1]. The combined KOZAK-CALSP-E1 fragment was then excised and ligated to XbaI-SacI cut pBIDST to generate the construct pBIDST-KCE1.
Figure 1. Schematic representation of the E1, E1cd and Gux1cd transgene expression cassettes used for transformation of tobacco and rice.
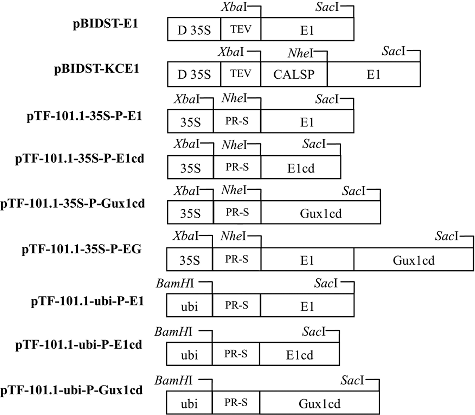
The apoplast transit peptide gene (PR-S, tobacco pathogenic resistance gene S, GeneBank accession number: AF284216.1) was synthesized by TaKaRa (Dalian, China). It was then inserted into pMD18T. The amplified E1, E1cd and Gux1cd DNA fragments were inserted downstream of the PR-S signal peptide sequence already cloned in pMD18T. The combined PR-S-E1, PR-S-E1cd or PR-S-Gux1cd fragment was cut and ligated into XbaI-SacI digested pTF-101.1-35S to generate either pTF-101.1-35S-P-E1, pTF-101.1-35S-P-E1cd or pTF-101.1-35S-P-Gux1cd. To construct the E1–Gux1cd fusion gene, Gux1cd was ligated to the 3′ end of the E1 gene containing the PR-S signal peptide sequence, and then the combined PR-E1–Gux1cd fragment was inserted into pTF-101.1-35S to yield pTF-101.1-35S-P-EG. In this construct, E1 and Gux1cd were linked by a hexa-glycine peptide.
To prepare the constructs for rice transformation, the PR-S-E1, PR-S-E1cd and PR-S-Gux1cd fragments were individually ligated into pTF-101.1-ubi to generate pTF-101.1-ubi-P-E1, pTF-101.1-ubi-P-E1cd and pTF-101.1-ubi-P-Gux1cd, using In-Fusion HD Cloning Kit (TaKaRa) for monocotyledon transformation.
Plant transformation
The plasmids pBIDST-E1, pBIDST-KCE1, pTF-101.1-35S-P-E1, pTF-101.1-35S-P-E1cd, pTF-101.1-35S-P-Gux1cd and pTF-101.1-35S-P-EG were used for tobacco transformation, whereas the plasmids pTF-101.1-ubi-P-E1, pTF-101.1-ubi-P-E1cd and pTF-101.1-ubi-P-Gux1cd were used for rice transformation. The plasmids were introduced into the tobacco and rice plants via Agrobacterium-mediated transformation. Positive tobacco transformants were selected on the basis of resistance to kanamycin, whereas positive rice transformants were selected by growth on medium containing hygromycin.
Molecular analysis
Genomic DNAs were isolated from tobacco and rice plants using a genomic DNA extraction kit (DP305, Tiangen Co., Dalian, China). The presence of E1, E1cd and Gux1cd inserts were confirmed by polymerase chain reaction (PCR) screening of the genomic DNA using a PCR kit (KT207, Tiangen, Dalian, China) with primer pairs 5′-CGTCTAGAATGGCGGGCGGCGGCTA-3′ and 5′-CTGAGCTCTTAACTTGCTGCGCAGG-3′, 5′-CGGCTAGCGCGGGCGGCGGCTA-3′ and 5′-CTGAGCTCTTAGACAGGATCGAAAATCG-3′, 5′-CGGCTAGCAACGACCCGTACATCCA-3′ and 5′-CTGAGCTCTTAGGGCGACCCGGATGGGA-3′, respectively. The PCR reactions were carried out using a Verity® thermocycler (HEROS Technology Co., Beijing, China).
Preparation of leaf extract
About 100 mg of leaf tissue was placed in a 1.5-mL microcentrifuge tube containing 200 μL of grinding buffer (50 mmol/L sodium acetate (pH 6.0), 100 mmol/L NaCl, 0.5 mmol/L ethylenediaminetetraacetic acid , 1 mmol/L phenylmethylsulphonyl fluoride, 10% (v/v) glycerol and 5 mmol/L 2-mercaptoethanol). The tube was then placed in liquid nitrogen, and the leaf tissue was homogenized with a small glass pestle. After homogenization, the insoluble materials were removed by centrifugation at 10,000 × g for 10 min at 4 °C. The supernatant was collected and subjected to cellulase activity and protein assays.
Cellulase activity and protein assays
Cellulase activity was measured by incubating the supernatant obtained above with the fluorogenic substrate, 4-methylumbelliferyl-β-D-cellobioside (MUC, Sigma, Spruce, St. Louis, MO, USA), at 65 °C for 30 min as described previously [Citation1]. The cleavage of 4-methylumbelliferyl-β-D-cellobioside by the enzymes release the fluorophore, 4-methylumbelliferone (MU), which was detected by excitation at 365 nm and emission at 445 nm. The specific cellulase activity was defined as the amount of MU released per min per one milligram of total soluble protein. The protein assay was carried out using the bicinchoninic acid method [Citation4].
Effects of temperature and pH
To test the thermostability of the recombinant cellulases, the supernatants obtained from the leaf extracts were incubated at 60, 65, 70, 75 and 80 °C for 30 min, and the residual cellulase activity of each sample was measured at 65 °C for 30 min. The effect of pH on cellulase activity was determined by carrying out the assay at 65 °C for 30 min, but at pH 4.0, 5.0, 6.0, 7.0, 8.0 and 9.0.
Storage stability
To examine the stability of recombinant cellulase, mature leaves from transgenic rice plants that expressed E1 were collected and stored at room temperature for 30 days, and the level of cellulase activity in the leaf extract was then measured and compared to that measured in the extract prepared from freshly harvested leaves.
Results and discussion
Molecular analysis
The presence of the recombinant cellulase genes in the genome of the positive transformants was confirmed by PCR using E1-, E1cd-, and Gux1cd-specific primers. PCR product was only obtained for the transformant that harboured one of these genes, and the sizes of the PCR products were 1563, 1074 and 1920 bp, respectively, for E1, E1cd and Gux1cd. No PCR product was obtained for the non-transformed plants. Positive transformants originally transformed with pBIDST-E1, pBIDST-KCE1, pTF-101.1-35S-P-E1, pTF-101.1-35S-P-E1cd, pTF-101.1-35S-P-Gux1cd, pTF-101.1-35S-P-EG, pTF-101.1-ubi-P-E1, pTF-101.1-ubi-P-E1cd and pTF-101.1-ubi-P-Gux1cd will henceforth be referred to as DST-E1, DST-KCE1, 35S-E1, 35S-E1cd, 35S-Gux1cd, 35S-EG, ubi-E1, ubi-E1cd and ubi-Gux1cd, respectively ().
Figure 2. PCR analysis of genomic DNA from tobacco and rice plants transformed with different cellulase-gene constructs. M, D2000 DNA markers (Dalian TaKaRa Bio Co., Dalian, China); lane 1: DST-E1; lane 2: DST-KCE1; lane 3: 35S-E1; lane 4: 35S-EG; lane 5: ubi-E1; lane 6: 35S-E1cd; lane 7: ubi-E1cd; lane 8: 35S-Gux1cd; lane 9: ubi-Gux1cd; lane 10: non-transgenic tobacco; lane 11: non-transgenic rice; lane 12: ddH2O.
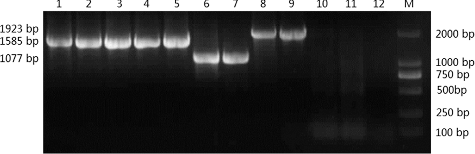
Cellulase activities
Most of the positive transgenic tobacco plants confirmed by PCR exhibited positive cellulase activity, with 35S-E1 showing the highest level, and 35S-Gux1cd the lowest level ((A)).
Figure 3. Enzyme activity measured with MUC as a substrate. (A) cellulase activity of extracts prepared from the leaves of tobacco plants that expressed E1, E1cd, Gux1cd or EG. (B) Cellulase activity of extracts prepared from the leaves of rice plants overexpressing E1, E1cd or Gux1cd.
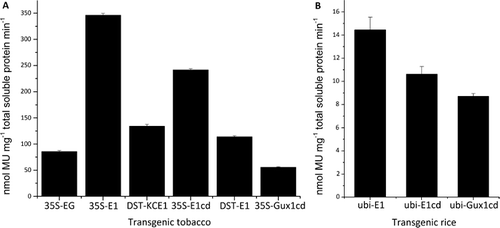
The location of the expressed cellulase within a specific sub-cellular compartment has been shown to profoundly affect its accumulation in plants [Citation4,Citation13]. The 5′ PR-S signal peptide has previously been shown to target, with reliability, heterologous proteins to plant cell apoplast after expression [Citation14]. Thus, for 35S-E1, the inclusion of the PR-S signal peptide in the construct allowed the expressed cellulase to be directed to the apoplast where it could accumulate in the intercellular space, therefore avoiding degradation by host proteases in the cytosol. As a result, 35S-E1 exhibited the highest level of cellulase activity, about twofold higher than that displayed by DST-E1, which did not carry the PR-S signal peptide sequence ((A)).
The attachment of the KOZAK-CALSP sequence to the 5′-end of the Thermobifida fusca E2 gene expressed in tobacco greatly facilitates the translocation of the expressed enzyme to the lumen for secretion and allows it to be targeted to the apoplast, resulting in marked increases in cellulase activity for the transgenic plant [Citation1]. However, the attachment of the KOZAK-CALSP sequence to E1 only resulted in slight enhancement of activity (compare DST-KCE1 with DST-E1 in (A)). We assumed this could possibly be due to the different cellulase genes used.
In the case of 35S-E1 and 35S-E1cd, the cellulase activity obtained for 35S-E1 was nearly 1.5-fold higher than that obtained for 35S-E1cd ((A)), and therefore the catalytic domain of E1 was as active as the holoenzyme, indicating that the catalytic domain could function without the carbohydrate-binding domain (CBD). However, the lower activity of E1cd showed that without the CBD, the conformation of the enzyme might be affected and its translocation across the cell envelope might be hindered. In addition, the MUC assay measures glucanase activity, which is based on the hydrolysis of soluble substrate. On the other hand, CBD has been demonstrated to enhance the ability of the cellulase to degrade insoluble substrates, even though it is not essential for the hydrolysis of crystalline cellulose [Citation15,Citation16].
Exoglucanase is more important for the degradation of amorphous or crystalline cellulose than for carboxymethyl cellulose, since such degradation would release soluble reducing sugars. To our knowledge, this study is the first report to describe the expression of an A. cellulolyticus exoglucanase Gux1 in plants. As for 35S-Gux1cd, the activity detected was relatively low, and this was not unexpected, since the activity of an exoglucanase is usually very low as assayed by the MUC method [Citation17].
The E1–Gux1cd fusion enzyme did not show any significant enhancement in cellulase activity over E1 alone (compare 35S-EG with 35S-E1 in (A)), although the hydrolysis of cellulose requires the concerted action of endo–exo cellulases [Citation18]. Since the two enzymes were fused via a six-glycine peptide chain, part of the structure of the individual enzyme components might be affected by the six-glycine peptide chain, resulting in an incorrectly folded structure. Alternatively, the six-glycine peptide chain might reduce the flexibility and synergistic action of the exo- and endo-glucanase domains in the E1–Gux1cd fusion enzyme [Citation19]. We concluded that the activity of E1–Gux1cd was mainly attributed to the E1 component, since expression of Guxcd alone (35S-Gux1cd) gave very low activity.
Rice straw has a high cellulose content (approximately 45%). Expression of cellulases in transgenic rice can facilitate the saccharification of cellulose in the rice plant, and this would significantly reduce the cost associated with the use of hydrolytic enzymes. Among the transgenic rice plants that expressed ubi-E1, ubi-E1cd and ubi-Gux1cd, only those that expressed ubi-E1 yielded the highest level of cellulase activity, with a specific activity of 20 nmol MU min−1 mg−1 total soluble protein ((B)). No severely impaired growth was observed for these plants. The significant difference in the level of cellulase activity between rice and tobacco expressing E1 could be related to the compositional differences between the primary structures of monocot (rice) and dicot (tobacco) cell walls [Citation20]. However, since an impressively high level of specific activity (850 nmol min−1mg−1) has been obtained for E1 expressed in maize [Citation2], we speculated that differences among the host plant species might also be a contributing factor.
Effects of temperature and pH
As rice is one of the world's main crops, we mainly focused on the properties of the cellulases expressed in rice. The effects of temperature and pH on enzyme stability were only investigated for ubi-E1, ubi-E1cd and ubi-Gux1cd. All three enzymes showed the same trend in thermostability, as determined by preincubation of leaf tissues at 60–80 °C prior to cellulase activity assay performed at 70 °C ((A)). They also had similar pH profiles, displaying optimum pH at around pH 5.0, with a rapid drop in activity upon further increases in pH ((B)).
Figure 4. Effects of temperature (A) and pH (B) on the activities of ubi-E1, ubi-E1cd and ubi-Gux1cd expressed in rice.
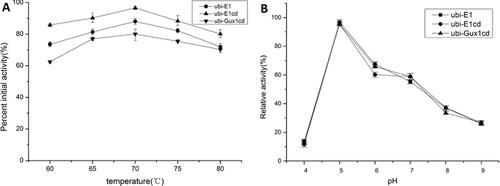
The recombinant A. cellulolyticus E1 was stable up to 70 °C ((A)), which was slightly less than the stability of the native enzyme [Citation4]. The reduced thermal stability of the enzyme may be attributed to the presence of additional amino acids from the insertion of the PR-S peptide that was introduced at the N-terminus of the mature enzyme. Other structural changes might be caused by the different physiological environments. Nevertheless, many studies have shown that most cellulases expressed in plants retain their native characteristics [Citation14]. However, if the recombinant enzyme has good thermostability, it will result in lower cost for downstream processing, since both transportation and enzyme purification can be carried out at room temperature, dispensing with the need for refrigeration equipment.
Storage stability
High storage stability for cellulases expressed in plants is important for long-term storage [Citation21]. The extract prepared from rice leaves that had been harvested and stored for one month at room temperature had only 75% of the activity of the extract prepared from fresh leaves (). This indicated that the enzyme was slightly affected by the drying process during storage. We have previously shown that T. fusca E2 expressed in tobacco lost about 80% activity after three days of storage at room temperature [Citation1]. Considering that one of the major factors that affects the stability of cellulase during storage is the optimal temperature of the enzyme, we concluded that the difference in optimal temperature between A. cellulolyticus E1 (81 °C) and T. fusca E2 (55 °C) might be the reason behind their different storage stability. The relatively high stability that we obtained for E1 expressed in rice would help to decrease the cost associated with post-harvest.
Conclusions
We expressed the thermostable bacterial cellulases, E1, E1cd and Gux1cd individually or as a fusion enzyme in tobacco and rice. All the recombinant cellulases were functional and showed good thermal stability. The apoplast-localized E1 showed the highest activity, threefold higher than that of the cytosolic E1. The transgenic plants showed no obvious morphological changes. The recombinant enzymes remained active in the rice plant after a 30-day storage at room temperature, with only 25% loss of activity. Taken together, the results of this study showed that transgenic plants could be used as potential bioreactors for the large-scale production of cellulases. We think that the future prospect of producing thermostable cellulases within the biomass is encouraging, and it would greatly reduce the cost of enzymes, since hydrolysis of the biomass can be carried out without addition of exogenous cellulases.
Disclosure statement
No potential conflict of interest was reported by the authors.
Additional information
Funding
References
- Jiang XR, Zhou XY, Jiang WY, et al. Expressions of thermostable bacterial cellulases in tobacco plant. Biotechnol Lett. 2011;33:1797–1803.
- Gadab C, Biswas G, Ransom C, et al. Expression of biologically active Acidothermus cellulolyticus endoglucanase in transgenic maize plants. Plant Sci. 2006;171:617–623.
- Chung D, Young J, Cha M, et al. Expression of the Acidothermus cellulolyticus E1 endoglucanase in Caldicellulosiruptor bescii enhances its ability to deconstruct crystalline cellulose. Biotechnol Biofuels. [ Internet]. 2015 [cited 2016 Mar 21];8:113. Available from: https://biotechnologyforbiofuels.biomedcentral.com/articles/10.1186/s13068-015-0296-x
- Dai Z, Hooker BS, Quesenberry R, et al. Optimization of Acidothermus cellulolyticus endoglucanase (E1) production in transgenic tobacco plants by transcriptional, post-transcription and post-translational modification. Transgenic Res. 2005;14:627–643.
- Olson DG, McBride JE, Shaw AJ, et al. Recent progress in consolidated bioprocessing. Curr Opin Biotechnol. 2012;23:396–405.
- Mazzoli R, Lamberti C, Pessione E. Engineering new metabolic capabilities in bacteria: lessons from recombinant cellulolytic strategies. Trends Biotechnol. 2012;30:111–119.
- Lambertz C, Garvey M, Klinger J, et al. Challenges and advances in the heterologous expression of cellulolytic enzymes: a review. Biotechnol Biofuels. [ Internet]. 2014 [cited 2016 Jan 18];7:135. Available from: https://biotechnologyforbiofuels.biomedcentral.com/articles/10.1186/s13068-014-0135-5
- Ziegelhoffer T, Raasch JA, Phillips SA. Expression of Acidothermus cellulolyticus E1 endo-β-1,4-glucanase catalytic domain in transplastomic tobacco. Plant Biotechnol J. 2009;7:527–536.
- Berlin A, Maximenko V, Gilkes N, et al. Optimization of enzyme complexes for lignocellulose hydrolysis. Biotechnol Bioeng. 2007;97:287–296.
- Jiang XR, Li WL. Research progress in genetic engineering for cellulosic ethanol. China Biotechnol. 2009;29:127–133.
- Edward MR. Genomics of cellulosic biofuels. Nature. 2008;454:841–845.
- Youm JW, Kim H, Han JH, et al. Expression of bacterial cellulose genes in transgenic alfalfa (Medicago sativa L.), potato (Solanum tuberosum L.) and tobacco (Nicotiana tabacum L.). Mol Breed. 1999;5:309–318.
- Klose H, Günl M, Usadel B, et al. Cell wall modification in tobacco by differential targeting of recombinant endoglucanase from Trichoderma reesei. BMC Plant Biol. [ Internet]. 2015 [ cited 2016 Mar 21];15:54. Available from: http://bmcplantbiol.biomedcentral.com/articles/10.1186/s12870-015-0443-3
- Ziegler MT, Thomas SR, Danna KJ. Accumulation of a thermostable endo-1,4-β-D-glucanase in the apoplast of Arabidopsis thaliana leaves. Mol Breed. 2001;6:37–46.
- Tomme P, Warren RA, Gilkes NR. Cellulose hydrolysis by bacteria and fungi. Adv Microb Physiol. 1995;37:1–81.
- Wang J, Gao G, Li Y, et al. Cloning, expression, and characterization of a thermophilic endoglucanase, AcCel12B from Acidothermus cellulolyticus 11B. Int J Mol Sci. 2015;16:25080–25095.
- Pang Z, Dong Z, Liang J, et al. Study on cellulase properties of thermophilic actinomycete GPL1. Mod Food Sci Technol. 2005;22:20–23.
- Wilson DB. Studies of Thermobifida fusca plant cell wall degrading enzymes. Chem Rec. 2004;4:72–82.
- Mingardon F, Chanal A, Tardif C, et al. Exploration of new geometries in cellulosome-like chimeras. Appl Environ Microbiol. 2007;73:7138–7149.
- Vogel J. Unique aspects of the grass cell wall. Curr Opin Plant Biol. 2008;11:301–307.
- Mir BA, Mewalal R, Mizrachi E, et al. Recombinant hyperthermophilic enzyme expression in plants: a novel approach for lignocellulose digestion. 2014;32:281–289.