ABSTRACT
Domain-of-unknown function (DUF) proteins represent a number of gene families with no functional annotation in the Pfam database. So far, the DUF866 family has not been characterized, and no member has been functionally studied. In this study, we uncovered and analysed four OsDUF866 (OsDUF866.1–OsDUF866.4) family members in rice Nipponbare, in which three distinct motifs were identified. The expression of OsDUF866 family in nine tissues was examined by real-time polymerase chain reaction (PCR), and the highest expression of four genes members was found in embryos at 14 days after flowering. We performed real-time PCR to examine the expression of OsDUF866 family under abiotic stress and abscisic acid (ABA) treatment conditions. The expression level of OsDUF866.1 displayed significant decrease under drought and salt conditions, while significant increase under heat conditions. The expression level of OsDUF866.2 displayed significant decrease under drought conditions. The expression level of OsDUF866.3 was significantly elevated under drought and cold, while lowered under heat conditions. The expression level of OsDUF866.4 was increased under cold and heat conditions, while decreased under drought conditions. Interestingly, the expression level of OsDUF866 members was approximately constant under ABA treatment conditions. Overexpression of OsDUF866.1 in Escherichia coli could enhance cell viability and significantly improve the resistance to heat stress conditions. The results may provide new insights into the function of OsDUF866 family in rice.
KEYWORDS:
Introduction
Many genes have not yet been characterized at the biochemical and biological function level, despite the fact that sequencing and annotation of the genome of some species of animals, plants and microorganisms have been completed. The domain of unknown function (DUF) is a protein domain of proteins with unknown function. The names of DUFs were given when protein families with no functional annotation were added to the Pfam database (http://pfam.xfam.org/family) [Citation1,Citation2]. There are about 3000 DUF families within the Pfam database, representing over 20% of known families [Citation1,Citation2]. About 2700 DUFs are found in bacteria compared with just over 1500 ones in eukaryotes [Citation3]. Due to the high structural conservation of many DUFs, they are considered to play very important roles in biology. However, many DUFs are considered to be not essential. For example, when DUF143, which is present in most bacteria and eukaryotic genomes, was deleted in Escherichia coli, there could not be detected any obvious change in the phenotype [Citation4]. It has been suggested that many DUFs are only required under certain specific conditions [Citation4].
Abiotic stresses, including heat, cold, salt and drought, have a significant impact on plant productivity and crop quality. As a sessile organism, rice is often subjected to various abiotic stresses. In order to preclude the detrimental impacts from specific stress factors and ensure survival, rice has evolved a complex cellular and molecular stress response system [Citation5–9]. At present, some genes have been used successfully to improve the tolerance of stresses in rice [Citation10–15].
In recent years, it has been reported that some DUF-containing proteins play important roles in plant stress responses. TaSRG, a wheat DUF662 domain-containing transcription factor, significantly affects salt tolerance in transgenic rice and Arabidopsis [Citation16]. Arabidopsis RING-DUF1117 E3 ubiquitin ligase genes AtRDUF1 and AtRDUF2 are induced by abscisic acid (ABA) and drought stress, and suppression of Arabidopsis AtRDUF1 and AtRDUF2 reduced tolerance to ABA-mediated drought stress [Citation17]. OsDSR2, encoding a protein with a DUF966 domain, negatively regulates the rice response to salt and simulated drought stresses as well as ABA signalling [Citation18]. Since the transcriptional levels of some DUF1618 gene family members vary under stress and hormone treatment conditions, it has been suggested that some DUF1618 members are probably involved in stress responses [Citation19]. OsSIDP366, a DUF1644 gene, positively regulates salt and drought resistance in rice [Citation20].
The DUF866 family consists of a number of hypothetical eukaryotic proteins with an average length of about 165 residues [Citation1]. However, so far the gene family has not been characterized, and no member has been functionally studied. In this study, we analysed the protein sequence of the DUF866-encoding genes (the OsDUF866 family) in rice, their spatio-temporal expression profile and their expression pattern under various stresses and ABA treatment. Furthermore, we overexpressed gene OsDUF866.1 in E. coli. The results may provide new insights into the function of the OsDUF866 family in rice.
Materials and methods
Database searches
The sequences of putative OsDUF866 family proteins in the rice genome were obtained by searching the rice genome annotation project (RGAP version 7) database (http://rice.plantbiology.msu.edu/) with domain number PF05907. All the corresponding protein sequences of the putative OsDUF866 family members were then downloaded from RGAP and confirmed with the SMART database (http://smart.embl-heidelberg.de/smart/batch.pl) [Citation21,Citation22]. If there were several gene models at one locus, only the complete gene model was selected for further sequence analysis.
Sequence analysis
Information about the chromosomal localization, amino acid (aa) length, full-length cDNA accessions was obtained for each OsDUF866 gene from RGAP and the Knowledge-Based Oryza Molecular Biological Encyclopedia (KOME) (http://cdna01.dna.affrc.go.jp/cDNA). PSORT (http://psort.hgc.jp/form.html) was used to predict the protein localization of OsDUF866 members. MEME version 4.11.2 (http://meme.nbcr.net/meme/) was used to predict motifs [Citation23]. Alignment of the protein sequences was performed using Clustal Omega [Citation24].
Plant growth and various stress and ABA treatment
Rice (Oryza sativa L. subsp. japonica cv. Nipponbare) seeds were germinated for three days, then seedlings were grown hydroponically in a 30-L vessel containing nutrient solution [Citation25]. At the emergence of the fourth leaf, the seedlings of rice Nipponbare were subjected to various stresses and ABA treatment. For high salinity treatment, NaCl solution was added to achieve a final concentration of about 200 mmol/L. Drought stress was realized by putting intact plants in the air without water supply. For cold stress, the vigorous seedlings were transferred to a growth chamber at 4 °C (12-h-light/12-h-dark cycle). For heat stress, the seedlings of rice Nipponbare were subjected to 42 °C heat shock treatment. For ABA treatment, 100 μmol/L solution was sprayed onto the leaves of seedlings.
To acquire the expression patterns of OsDUF866 family members at different stages, nine representative tissues were sampled: one-week-old seedlings, shoot at the four-leaf stage, root at the four-leaf stage, leaf from plants with four tillers, leaf sheath from plants with four tillers, stem from plants with four tillers, embryo 14 days after flowering, leaf at 14 days after flowering and stem at 14 days after flowering.
RNA extraction and real-time PCR
Samples were collected and ground to fine powder in liquid nitrogen. Total RNA was extracted with Trizol reagent (GIBCO, Burlington, ON, USA), according to the manufacturer's instructions. Then, the total RNA was treated with RNase-free DNase I (Invitrogen, Carlsbad, CA, USA) for 15 min to degrade any potentially contaminated DNA. Real-time PCR was performed on an optical 96-well plate with a Bio-Rad (Cambridge, MA, USA) CFX96 real-time PCR system. Each reaction contained 5 µL of 2× SYBR® Green Master Mix Reagent (Roche, Indianapolis, IN, USA), 1 µL of cDNA samples and 200 nmol/L gene-specific primer in a final volume of 10 µL. The thermal cycle used was as follows: 95 °C for 10 min, followed by 39 cycles of 95 °C for 10 s, 60 °C for 30 s and 72 °C for 1 min. The rice OsUbiquitin gene (LOC102716385) was used as an internal control with primers 5'-AACCAGCTGAGGCCCAAGA-3' and 5'-ACGATTGATTTAACCAGTCCATGA-3'. The primers used in the OsDUF866 gene family members’ expression analysis are listed in Table S1 in the Supplementary Appendix. Data analyses of the relative expression levels with the 2−ΔΔCt method were performed as described previously [Citation26].
Assay for heat stress tolerance of E. coli transformants
In order to construct the expression vector pET32a-OsDUF866.1, specific primers were designed as follows: sense, 5'-GGATCCGTGACGCACTACCGCCGCGA-3' (BamHI site underlined), and antisense, 5'-AAGCTTACGAAATGATCCAGGTTAAT-3' (HindIII site underlined). The amplified products were cloned into pET32a vector at the BamHI–HindIII site to express the pET32a-OsDUF866.1 fusion protein, which has a Trx•Tag™ thioredoxin at the N-terminus [Citation27]. The transformed E. coli Rosetta cells (Sanborn, MN, USA) were grown in Luria–Bertani (LB) liquid medium containing 100 µg/mL of ampicillin at 37 °C overnight. Then they were inoculated into fresh LB medium (1:100 dilution) supplemented with ampicillin (100 µg/mL) to incubate until exponential growth phase (optical density at 600 nm (OD600) of 0.5–0.6). Isopropylthio-β-D-galactoside (IPTG) was added into the cultures to induce the expression of the transformed gene OsDUF866.1. For the assay of the heat tolerance, 2 mL samples were placed into 50 °C water bath. At 0, 0.5, 1, 1.5, 2, 2.5 h after heat shock, respectively, 10 μL of different dilutions (1:100) were spotted onto LB agar plates with 1 mmol/L IPTG.
Data analysis
The experiments were repeated three times. Data are mean values from three independent experiments, with standard error of the means (±SEM). Statistical analysis was performed using the Student's t-test by Microsoft EXCEL version 2003.
Results and discussion
Identification and sequence analysis of OsDUF866 family members in rice
Four OsDUF866 gene family members were identified () by searching the RGAP with domain number PF05907. Then, all the members of OsDUF866 were confirmed with the SMART database. These genes were named OsDUF866.1–OsDUF866.4 according to their positions on pseudomolecules (). The four members of the rice OsDUF866 gene were found to be distributed on chromosome 1 (OsDUF866.1) and 6 (OsDUF866.2, OsDUF866.3 and OsDUF866.4), respectively. Only the full-length cDNAs of OsDUF866.1 was found in the KOME database (). The length of the OsDUF866 proteins was predicted to vary from 68 (OsDUF866.4) to 232 (OsDUF866.2) amino acids. OsDUF866.2 was predicted to be located in the nucleus, while OsDUF866.1, OsDUF866.3 and OsDUF866.4 were predicted to be located in the cytoplasm. Details about the four OsDUF866 family members, including ID numbers, number of introns, isoelectric point (pI) and molecular weight (MW) are provided in
Table 1. Structure information of OsDUF866 gene and protein.
The multiple sequence alignment analysis of OsDUF866 members (Figure S1 in the Supplementary Appendix) showed that the per cent similarity was 30.37 (OsDUF866.1 and OsDUF866.2), 28.30 (OsDUF866.1 and OsDUF866.3), 22.03 (OsDUF866.1 and OsDUF866.4), 42.34 (OsDUF866.2 and OsDUF866.3), 50.00 (OsDUF866.2 and OsDUF866.4) and 39.66 (OsDUF866.3 and OsDUF866.4), respectively. The MEME motif search tool used for examination of the four putative OsDUF866 sequences identified three distinct motifs (Figure S2A in the Supplementary Appendix). Notably, motif 2 and motif 3 were found in all the OsDUF866 members, whereas motif 1 was found in all OsDUF866 members except for OsDUF866.4 (Figure S2B).
There are 10 duplicated blocks accounting for 45% of the total size of the rice genome [Citation28]. Gene duplication events including segmental duplication, tandem duplication and transposition, are all important to the evolution of gene families [Citation29]. As OsDUF866.3 and OsDUF866.4 are very adjacent to each other in terms to their genomic location (1.7 kb), it is likely that these two genes were the results of a segmental duplication event in the evolution of the genome. Motif 1 was not found in OsDUF866.4, suggesting that the DNA fragment of OsDUF866.4 corresponding to motif 1 may have been lost in the process of gene duplication from OsDUF866.3 to OsDUF866.4.
Expression patterns of OsDUF866 family members in various tissues
So far little is known about the expression patterns of OsDUF866 family members in various tissues of rice at different stages. To acquire more information in this regard, we examined the expression of the OsDUF866 family members in nine tissues. The results indicated that OsDUF866.1, OsDUF866.2, OsDUF866.3 and OsDUF866.4 were all widely expressed in almost all the samples and the highest expression of the four genes was found in the embryo at 14 days after flowering (). It was suggested that OsDUF866 family members play vital roles in all tissues at the stage of growth, development and maturation in rice.
Figure 1. Real-time PCR analysis of OsDUF866 gene family members in rice Nipponbare in different tissues: Sd, one-week-old seedlings; St, shoot at the four-leaf stage; Rt, root at the four-leaf stage; Lf1, leaf from plants with four tillers; Ls, leaf sheath from plants with four tillers; St1, stem from plants with four tillers; Em, embryo 14 days after flowering; Lf2, leaf at 14 days after flowering; St2, stem at 14 days after flowering.
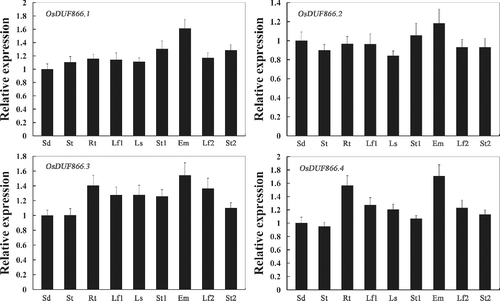
Expression analysis of OsDUF866 genes under various stress conditions
At present, there is little information on the expression of OsDUF866 genes under abiotic stress conditions. To study the expression of this gene family under various types of abiotic stress, seedlings of rice Nipponbare were subjected to drought, salt, cold, heat stress and ABA treatment at the emergence of the fourth leaf. The real-time PCR results showed that there were distinct changes in the expression of OsDUF866 genes when rice was subjected to various stresses (). The expression level of OsDUF866.1 displayed a significant decrease under drought and salt conditions, whereas a significant increase under heat conditions. The expression level of OsDUF866.2 decreased significantly under drought conditions. The expression level of OsDUF866.3 was significantly elevated under drought and cold stress but was lowered under heat stress conditions. The expression level of OsDUF866.4 was increased under cold and heat conditions, whereas it decreased in conditions of drought stress. Interestingly, the expression level of OsDUF866 members was approximately constant under ABA treatment conditions.
Figure 2. Relative expression levels of OsDUF866 gene family members in rice Nipponbare under various stresses and ABA conditions: D, drought; S, salt; C, cold; H, heat; A, ABA.
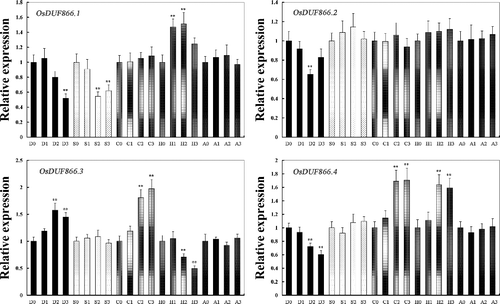
The expression level of some gene family members usually shows a common significant increase or decrease under various stresses. For example, OsDREB1A, OsDREB1B and OsDREB1C, three members of the OsDREB1 family, were all induced during the early stage of the cold response, and the expression of these genes could activate diverse sets of cold-responsive genes so as to better deal with the low temperature [Citation30–32]. In our study, four members of the OsDUF866 family in rice displayed very different expression patterns in RNA level under various abiotic stress conditions. This observation suggests that OsDUF866 family members probably play different roles in response to stresses.
OsDUF866.1 improved heat stress resistance in transgenic E. coli
Based on the fact that the expression level of OsDUF866.1 displayed significant increase under heat conditions, we speculated that this protein might be involved in response to heat resistance. To test this hypothesis, we overexpressed this gene in E. coli and tested the resistance of this bacterium to heat. We determined the growth effect on E. coli recombinants overexpressing OsDUF866.1 under heat stress (). Under normal conditions, the growth rate in E. coli transformed with pET32a-OsDUF866.1 was the same as that of E. coli transformed with pET-32a, whereas under heat conditions, the number of transgenic colonies (Rosetta/OsDUF866.1) was much higher than that in the control (Rosetta/ pET-32a) at 0.5, 1, 1.5, 2, 2.5 h after heat treatment, respectively.
The overexpression of some heat shock protein genes can enhance heat tolerance in E. coli [Citation33–36]. For example, the expression of OsHSP90-2 was up-regulated under heat conditions and the overexpression of OsHSP90-2 in E. coli enhanced the cell viability and significantly improved the resistance to heat [Citation36]. In our study, OsDUF866.1 also improved heat stress resistance in transgenic E. coli. However, the mechanism of enhancing the heat tolerance in E. coli is not very clear. It could be speculated that OsDUF866.1 may possibly interact with some heat shock protein, and the protein–protein interaction might lead to improvement in the stress resistance in transgenic E. coli.
Conclusions
In this study, we identified four OsDUF866 family members and provided important information regarding these members. We also explored the expression patterns of the four OsDUF866 family members in different tissues, and under various abnormal conditions including stress and ABA. Furthermore, we overexpressed gene OsDUF866.1 in E. coli, and found that OsDUF866.1 could improve the resistance to heat stress in transgenic E. coli. These data can provide important reference for further studies of the functions of the OsDUF866 family.
tbeq_a_1268932_sm9159.pdf
Download PDF (720.8 KB)Disclosure statement
No potential conflict of interest was reported by the authors.
Additional information
Funding
References
- Finn RD, Coggill P, Eberhardt RY, et al. The Pfam protein families database: towards a more sustainable future. Nucleic Acids Res. 2016;44:D279–D285.
- Bateman A, Coggill P, Finn RD. DUFs: families in search of function. Acta Crystallogr Sect F Struct Biol Cryst Commun. 2010;66:1148–1152.
- Goodacre NF, Gerloff DL, Uetz P. Protein domains of unknown function are essential in bacteria. MBio [Internet]. 2014 [ cited 2016 Jul 2];5:e00744–00713. Available from: http://mbio.asm.org/content/5/1/e00744-13.long.
- Häuser R, Pech M, Kijek J, et al. RsfA (YbeB) proteins are conserved ribosomal silencing factors. PLoS Genet [Internet]. 2012 [ cited 2016 Jul 2];8:e1002815. Available from: http://journals.plos.org/plosgenetics/article?id=10.1371/journal.pgen.1002815.
- Wang W, Vinocur B, Altman A. Plant responses to drought, salinity and extreme temperatures: towards genetic engineering for stress tolerance. Planta. 2003;218:1–14.
- Herms DA, Mattson WJ. The dilemma of plants: to grow or defend. Q Rev Biol. 1992;67(3):283–335.
- Soltis DE, Soltis PS. The role of phylogenetics in comparative genetics. Plant Physiol. 2003;132:1790–1800.
- Xiang Y, Tang N, Du H, et al. Characterization of OsbZIP23 as a key player of the basic leucine zipper transcription factor family for conferring abscisic acid sensitivity and salinity and drought tolerance in rice. Plant Physiol. 2008;148:1938–1952.
- Bohnert HJ, Gong Q, Li P, et al. Unraveling abiotic stress tolerance mechanisms–getting genomics going. Curr Opin Plant Biol. 2006;9:180–188.
- Sato H, Todaka D, Kudo M, et al. The Arabidopsis transcriptional regulator DPB3-1 enhances heat stress tolerance without growth retardation in rice. Plant Biotechnol J. 2016;14(8):1756–1767.
- Liu J, Zhang C, Wei C, et al. The RING finger ubiquitin E3 ligase OsHTAS enhances heat tolerance by promoting H2O2-induced stomatal closure in rice. Plant Physiol. 2016;170:429–443.
- Hong Y, Zhang H, Huang L, et al. Overexpression of a stress-responsive NAC transcription factor gene ONAC022 improves drought and salt tolerance in rice. Front Plant Sci [ Internet]. 2016 [ cited 2016 Jul 2];7:4. Available from: http://journal.frontiersin.org/article/10.3389/fpls.2016.00004/full.
- Yoon DH, Lee SS, Park HJ, et al. Overexpression of OsCYP19-4 increases tolerance to cold stress and enhances grain yield in rice (Oryza sativa). J Exp Bot. 2016;67:69–82.
- Min HJ, Jung YJ, Kang BG, et al. CaPUB1, a hot pepper U-box E3 ubiquitin ligase, confers enhanced cold stress tolerance and decreased drought stress tolerance in transgenic rice (Oryza sativa L.). Mol Cells. 2016;39:250–257.
- Alam MM, Tanaka T, Nakamura H, et al. Overexpression of a rice heme activator protein gene (OsHAP2E) confers resistance to pathogens, salinity and drought, and increases photosynthesis and tiller number. Plant Biotechnol J. 2015;13:85–96.
- He X, Hou X, Shen Y, et al. TaSRG, a wheat transcription factor, significantly affects salt tolerance in transgenic rice and Arabidopsis. FEBS Lett. 2011;585:1231–1237.
- Kim SJ, Ryu MY, Kim WT. Suppression of Arabidopsis RING-DUF1117 E3 ubiquitin ligases, AtRDUF1 and AtRDUF2, reduces tolerance to ABA-mediated drought stress. Biochem Biophys Res Commun. 2012;420:141–147.
- Luo C, Guo C, Wang W, et al. Overexpression of a new stress-repressive gene OsDSR2 encoding a protein with a DUF966 domain increases salt and simulated drought stress sensitivities and reduces ABA sensitivity in rice. Plant Cell Rep. 2014;33:323–336.
- Wang L, Shen R, Chen LT, et al. Characterization of a novel DUF1618 gene family in rice. J Integr Plant Biol. 2014;56:151–158.
- Guo C, Luo C, Guo L, et al. OsSIDP366, a DUF1644 gene, positively regulates responses to drought and salt stresses in rice. J Integr Plant Biol. 2016;58:492–502.
- Schultz J, Milpetz F, Bork P, et al. SMART, a simple modular architecture research tool: identification of signaling domains. Proc Natl Acad Sci USA. 1998;95:5857–5864.
- Letunic I, Doerks T, Bork P. SMART: recent updates, new developments and status in 2015. Nucleic Acids Res. 2014;43(Database issue):D257–D260.
- Bailey TL, Boden M, Buske FA, et al. MEME SUITE: tools for motif discovery and searching. Nucleic Acids Res. 2009;37:W202–W208.
- Sievers F, Wilm A, Dineen D, et al. Fast, scalable generation of high-quality protein multiple sequence alignments using Clustal Omega. Mol Syst Biol [Internet]. 2011 [ cited 2016 Jul 2];7:539. Available from: http://msb.embopress.org/content/7/1/539.long.
- Yoshida S, Forno DA, Cook JH, et al. Laboratory manual for physiological studies of rice. Manila: International Rice Research Institute; 1976. p. 61–67.
- Livak KJ, Schmittgen TD. Analysis of relative gene expression data using real-time quantitative PCR and the 2(-Delta Delta C(T)) method. Methods. 2001;25:402–408.
- LaVallie ER, DiBlasio EA, Kovacic S, et al. A thioredoxin gene fusion expression system that circumvents inclusion body formation in the E. coli cytoplasm. Biotechnology (N Y). 1993;11:187–193.
- Wang X, Shi X, Hao B, et al. Duplication and DNA segmental loss in the rice genome: implications for diploidization. New Phytol. 2005;165:937–946.
- Kong H, Landherr LL, Frohlich MW, et al. Patterns of gene duplication in the plant SKP1 gene family in angiosperms: evidence for multiple mechanisms of rapid gene birth. Plant J. 2007;50:873–885.
- Dubouzet JG, Sakuma Y, Ito Y, et al. OsDREB genes in rice, Oryza sativa L., encode transcription activators that function in drought-, high-salt- and cold-responsive gene expression. Plant J. 2003;33:751–763.
- Ito Y, Katsura K, Maruyama K, et al. Functional analysis of rice DREB1/CBF-type transcription factors involved in cold-responsive gene expression in transgenic rice. Plant Cell Physiol. 2006;47:141–153.
- Su CF, Wang YC, Hsieh TH, et al. A novel MYBS3-dependent pathway confers cold tolerance in rice. Plant Physiol. 2010;153:145–158.
- Liu D, Lu Z, Mao Z, et al. Enhanced thermotolerance of E. coli by expressed OsHsp90 from rice (Oryza sativa L.). Curr Microbiol. 2009;58:129–133.
- Seo JS, Lee YM, Park HG, et al. The intertidal copepod Tigriopus japonicus small heat shock protein 20 gene (Hsp20) enhances thermotolerance of transformed Escherichia coli. Biochem Biophys Res Commun. 2006;340:901–908.
- Yeh CH, Chang PF, Yeh KW, et al. Expression of a gene encoding a 16.9-kDa heat-shock protein, Oshsp16.9, in Escherichia coli enhances thermotolerance. Proc Natl Acad Sci USA. 1997;94:10967–10972.
- Zhang H, Li LH, Ye TZ, et al. Molecular characterization, expression pattern and function analysis of the OsHSP90 family in rice. Biotechnol Biotechnol Equip. 2016;30:669–676.