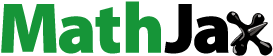
ABSTRACT
The present study used water-electrode plasma discharge to increase the effect of bacterial inactivation in water for bioengineering and biotechnological applications. The water-electrode plasma discharge system was fabricated using a newly designed plasma generator and a high-voltage power supply. Water contaminated with Escherichia coli was treated with water-electrode plasma discharge for 0, 1, 2, 3, 4, 5, 10, 20 and 30 min. As a result, the colony-forming units (cfu) of E. coli were reduced with plasma treatment time, reaching nearly complete inactivation after 30 min. In addition, rapid generation of H2O2 in the contaminated water was observed, which could mainly account for the effective bacterial inactivation. In conclusion, direct generation of reactive chemical species under water was successfully achieved by using a water-electrode plasma discharge system, which could be practically used to enhance bacterial inactivation in a variety of bioengineering applications.
Introduction
Water contamination due to pathogenic microorganisms and invasive species has become a global concern. Such contamination of water has a harmful influence not only on the environment, but also on human health [Citation1,Citation2]. Moreover, it has been reported as a critical issue in seawater that 7000 or more different microbial species are annually transported through ballast water, resulting in destruction and contamination of marine ecosystems [Citation3–5]. Many research studies have been undertaken in the search for effective approaches to treat contaminated water. Mori et al. [Citation6] and Olsen et al. [Citation7] suggested an ultra violet light-emitting diode (UV-LED) device as an effective method to sterilize water. Sinclair et al. [Citation8] conducted water sterilization using cellulose membrane filters grafted with silver nanoparticles and nanowires. Shahid et al. [Citation9] showed the sterilizing effect of hot gas bubbles by promoting the collision of biological species.
Plasma discharge has been suggested as one of the most effective methods to enhance the inactivation of microorganisms in water. When high-voltage plasma discharge is generated in water or on the water surface, highly reactive species (i.e. O, O3, OH, H+, H2O2), shock waves and UV light can be generated, which can affect the inactivation of microorganisms, especially bacteria, in water [Citation10–13]. However, the high conductivity of water prevents the direct generation of plasma discharge in water, which requires additional devices to transfer plasma-generated species into water [Citation14,Citation15]. Therefore, bacterial inactivation in water using conventional plasma discharge was achieved by transferring and dissolving the externally generated reactive species into water. Furthermore, the efficacy of the bacterial inactivation could be decreased, because most of the reactants generated by plasma discharge have a short half-life, showing a substantial decrease in concentration while travelling through water [Citation16–18].
As a way to overcome the aforementioned issues, the aim of this study was to develop a water-electrode plasma discharge system which could directly generate substances with a bactericidal effect in the contaminated water for enhanced inactivation of bacteria. The effect of the water-electrode plasma discharge system on bacterial inactivation was investigated using water artificially contaminated with Escherichia coli. The survival rate of E. coli was measured using heterotrophic plate counts (HPCs). The change in the concentration of hydrogen peroxide (H2O2) was analyzed, and the pH values of the contaminated water were also measured.
Materials and methods
Experimental setup
The experimental system was prepared to generate water-electrode plasma discharge in water, as shown in . The experimental system consisted of the contaminated water reservoir, plasma generator, power supply and air compressor. The contaminated water reservoir was fabricated using a transparent and dielectric acrylic material in order to visually confirm the stable generation of plasma. The plasma generator was connected to the contaminated water reservoir. A monomer cast nylon insulator was fabricated to prevent electrical contact between the plasma generator and water. The air compressor was connected to the plasma generator to introduce the air, and the air pressure was regulated using a pressure regulator. The power supply was connected to the plasma generator to deliver 200 W of energy with maximum voltage of 3 kV. A tungsten electrode was inserted into the reservoir to ground the water. The tungsten electrode was installed perpendicular to the air stream from the plasma generator to minimize the mutual interference between the air bubbles and the tungsten electrode.
Figure 1. Schematic diagram of the water-electrode plasma discharge system and a sectional view of a plasma generator. P.R., pressure regulator; H.V., high voltage power supply.
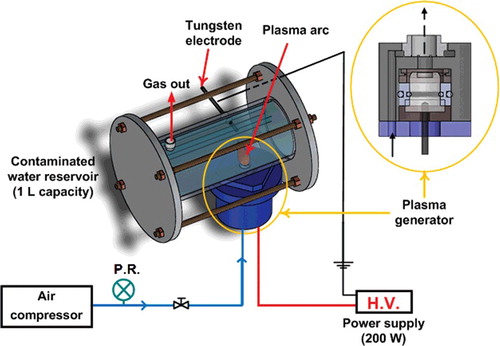
The mechanism of direct plasma generation in water can be described as follows. First, the current was induced to the electrode installed in the plasma generator. Second, air was introduced into the plasma generator, so that it could help with the generation of the plasma discharge between the electrode and water. Third, an increase in the electrical field inside the air bubble induced the generation of plasma discharge in the water.
A detailed sectional view of the plasma generator is shown in . Air with a constant pressure of 1.54 bar was continuously supplied to the bottom of the plasma generator during the operation of the air compressor (KP-ERIC-A2 1/6HP, ERIC K, Taiwan). Thermal plasma discharge was generated as soon as the air was introduced into the plasma generator due to high voltage. As the discharged plasma rotated through the internal spiral flow path, transition of the discharged plasma from thermal to non-thermal could be achieved. This simultaneous generation of both thermal and non-thermal plasma discharge has an advantage of generating higher concentration of reactive species for bacterial inactivation [Citation15,Citation17].
Sample preparation and experimental procedure
Sample water was prepared by mixing 1 L of distilled water and 37.5 g of salt (Advanced pro formula salt, Royal Nature, Israel) to provide the conductive characteristics of water. The solution was stirred sufficiently using an automatic stirrer (Eurostar/IKA, Staufen, Germany) at a constant speed of 500 r/min for one hour. Both the conductivity and the pH of the prepared artificial water were measured and maintained within the known ranges of natural water.
In order to investigate the influence of the plasma discharge on bacterial inactivation, E. coli was cultured at a concentration of 106 colony-forming units (cfu) per mL. The E. coli cell suspension was diluted in 1 L of the prepared water. The contaminated water was then placed in the water reservoir. The experiment began immediately to minimize the influence of bacterial inactivation by water itself [Citation19,Citation20]. The water contaminated with E. coli was treated with water-electrode plasma discharge for 0, 1, 2, 3, 4, 5, 10, 20 and 30 min. At each experiment, the contaminated water reservoir was rinsed with distilled water three times, and cleaned with ethanol to remove the remaining microorganisms.
Measurements of bacterial inactivation after water-electrode plasma discharge
After treatment of the contaminated water with water-electrode plasma discharge, 10 mL of the treated water in the reservoir was collected in a sterile tube. Then, 1 mL of the collected sample was taken using a sterile pipette, and it was spread onto a Luria–Bertani (LB) agar plate (Lennox L agar, Thermo Fisher Scientific, USA). The agar plates containing 1 mL of the plasma-treated samples were dried inside a clean bench for 30 min. After drying, the agar plates were incubated for 18 h at 37 °C. After incubation, heterotrophic plate counting (HPC) images were recorded with a camera, and the E. coli cell counts (cfu) were scored using a colony counter (Digital Colony Counter HYC-560, Hanyang Scientific Equipment, South Korea). All the experiments were repeated five times, and the results are presented as means with standard deviation.
Measurements of pH and H2O2
In order to investigate the mechanism of bacterial inactivation by water-electrode plasma discharge, both pH and the concentration of H2O2 were measured. After treatment of the contaminated water with water-electrode plasma discharge, 50 mL of the plasma-treated water was collected from the reservoir and the pH was measured using a pH meter (Orion Star A211, Thermo Fisher Scientific, USA). Prior to the pH measurement, the pH meter was calibrated using standard pH solutions (pH 4, 7 and 10) provided by the pH meter manufacturer. The generation of H2O2 in the contaminated water during water-electrode plasma discharge was measured using a H2O2 test strip (MQuant ™ Peroxide Test, Merck KGaA, Germany). Since the generated H2O2 has an extremely short half-life, an immediate measurement using a test strip was chosen instead of using a commercial H2O2 meter. The test strip was soaked into the plasma-treated water for three seconds, and the change in colour was observed. Both the salinity and conductivity were monitored while the contaminated water was treated with water-electrode plasma discharge for 0, 1, 2, 3, 4, 5, 10, 20 and 30 min. All the experiments were repeated five times, and the results are presented as means with standard deviations.
Results and discussion
After the contaminated water was treated with water-electrode plasma discharge for 1–30 min, the survival rates of E. coli were determined (). Prior to water-electrode plasma discharge, the initial concentration of E. coli in the contaminated water was 106 cfu/mL. After about 10 min of water-electrode plasma treatment, the cell counts steadily decreased to 295 ± 17 cfu/mL. This trend of cfu reduction was enhanced as a function of time, and after 30 min of plasma treatment, the E. coli was nearly completely inactivated.
Figure 2. Bactericidal effect of water-electrode plasma discharge on E. coli in water. HPC images and CFU values at 0–30 min of water-electrode plasma discharge treatment.
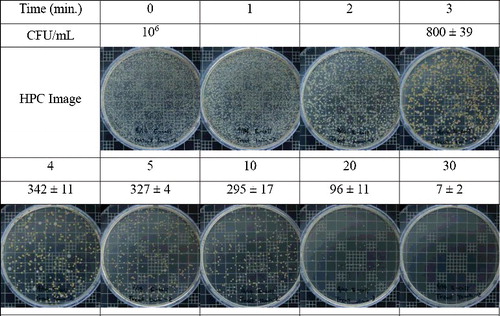
shows the concentrations of H2O2 in the contaminated water generated by water-electrode plasma discharge. The concentration of H2O2 increased steadily with treatment time from 0 to 5 mg/L for 10 min. When the plasma treatment was continued for more than 15 min, an exponential increase in the concentration of H2O2 was observed, which was inversely proportional to the reduction of the cell counts. No significant change of salinity and conductivity were observed. After an underwater chemical reaction was generated by water-electrode plasma discharge, H2O2, an excellent bactericidal agent with a relatively long half-life, could be generated. It is known that when water comes in direct contact with plasma discharge, the plasma can cause dissociation of water molecules and generation of reactive species. The chemical reaction by which the H2O2 was produced could be as follows [Citation17,Citation21–23]:(1)
(1)
(2)
(2)
(3)
(3)
(4)
(4)
(5)
(5)
Figure 3. Accumulation of hydrogen peroxide in contaminated water during 30-min water-electrode plasma discharge treatment.
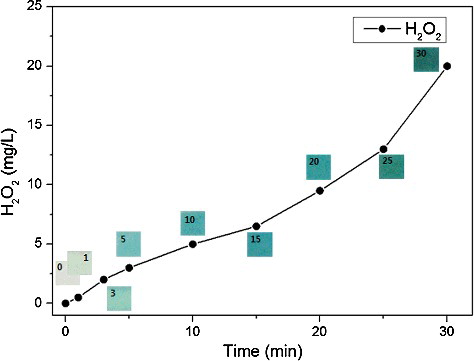
Thus, diverse radicals participating in the H2O2-generation process could also be produced, resulting in enhanced bactericidal effect in water. In addition, the contaminated water itself played a role as an electrode with high electrical conductivity, so that the electric field could be formed in the contaminated water, causing water electrolysis. The reactants produced by the electrolysis reaction could also occur in the contaminated water, enhancing the bacterial inactivation.
The concentration of H+ ions in the plasma-treated water might increase in the H2O2-generation process. The contaminated water became acidic as a result of water-electrode plasma treatment (). For five minutes of plasma treatment, the initial pH value of 7.91 was slightly reduced to about pH 7.44. Then, the pH showed a gradual decrease down to pH 7.18 for 30 min of plasma treatment. Since several studies report that the lower the pH of water is, the higher the survival rate of E. coli becomes within the pH range of 5.0–9.0 [Citation24–26], it could be speculated that, in our experimental setup, other factors besides the pH value most likely play a role in the bactericidal effect observed at 30 min plasma discharge. Some powerful bactericidal agents (e.g. H2O2, radicals, UV light and electrolysis) generated by water-electrode plasma discharge could be more effective than the pH change.
Figure 4. pH reduction in contaminated water during 30-min water-electrode plasma discharge treatment.
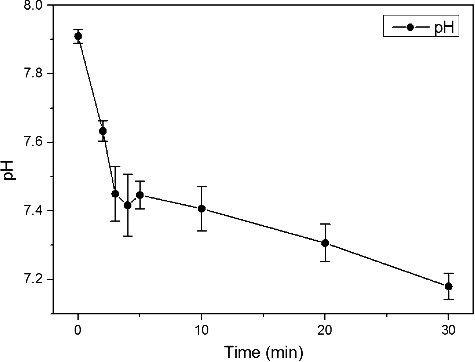
In this study, the possibility for water sterilization using a water-electrode plasma discharge system was successfully demonstrated. However, there are several limitations to our study. The suggested water-electrode plasma system needs to be quantitatively characterized in terms of the bactericidal effect of lasting H2O2, the bacterial inactivation impact of water conductivity, and environmental safety for practical application, which requires further investigation in future studies.
Conclusions
We investigated the effects of water-electrode plasma discharge on bacterial inactivation. After 30 min of plasma treatment, nearly complete bacterial inactivation was observed. The concentration of H2O2 was proportionally increased up to 20 mg/L for 30 min of plasma treatment. The water-electrode plasma discharge-driven direct production of H2O2 in the contaminated water most likely contributed to the inactivation of E. coli. In addition, during the H2O2-generation process, additional bactericidal agents could be generated in the contaminated water, causing enhancement of the bacterial inactivation. Therefore, water-electrode plasma discharge could provide consolidated effects for elimination of microorganisms in water, opening up opportunities for a variety of bioengineering applications.
Disclosure statement
No potential conflict of interest was reported by the authors.
Additional information
Funding
References
- Abd-Elnaby H, Abo-Elala G, Abdel-Raouf U, et al. Antibacterial and anticancer activity of marine Streptomyces parvus: optimization and application. Biotechnol Biotechnol Equip. 2016;30:180–191.
- Emmanuel E, Pierre MG, Perrodin Y. Groundwater contamination by microbiological and chemical substances released from hospital wastewater: health risk assessment for drinking water consumers. Environ Int. 2009;35:718–726.
- Seiden JM, Way C, Rivkin RB. Microbial hitchhikers: dynamics of bacterial populations in ballast water during a trans-Pacific voyage of a bulk carrier. Aquat Invasions. 2010;5:13–22.
- David M, Perkovič M. Ballast water sampling as a critical component of biological invasions risk management. Mar Pollut Bull. 2004;49:313–318.
- Hallegraeff GM. Transport of toxic dinoflagellates via ships ballast water: bioeconomic risk assessment and efficacy of possible ballast water management strategies. Mar Ecol Prog Ser. 1998;168:297–309.
- Mori M, Hamamoto A, Takahashi A, et al. Development of a new water sterilization device with a 365 nm UV-LED. Med Biol Eng Comput. 2007;45:1237–1241.
- Olsen RO, Hoffmann F, Hess-Erga O-K, et al. Ultraviolet radiation as a ballast water treatment strategy: inactivation of phytoplankton measured with flow cytometry. Mar Pollut Bull. 2016;103:270–275.
- Sinclair T, Zieba M, Irusta S, et al. High-speed water sterilization using silver-containing cellulose membranes. Nanotechnology. 2014;25:305101.
- Shahid M, Pashley R, Mohklesur RA. Use of a high density, low temperature, bubble column for thermally efficient water sterilization. Desalin Water Treat. 2014;52:4444–4452.
- Scholtz V, Pazlarova J, Souskova H, et al. Nonthermal plasma—a tool for decontamination and disinfection. Biotechnol Adv. 2015;33:1108–1119.
- Zheng C, Xu Y, Huang H, et al. Water disinfection by pulsed atmospheric air plasma along water surface. AIChE J. 2013;59:1458–1467.
- Hijosa-Valsero M, Molina R, Schikora H, et al. Removal of priority pollutants from water by means of dielectric barrier discharge atmospheric plasma. J Hazard Mater. 2013;262:664–673.
- Locke B, Sato M, Sunka P, et al. Electrohydraulic discharge and nonthermal plasma for water treatment. Ind Eng Chem Res. 2006;45:882–905.
- De Geyter N, Morent R. Nonthermal plasma sterilization of living and nonliving surfaces. Annu Rev Biomed Eng. 2012;14:255–274.
- Burlica R, Kirkpatrick MJ, Locke BR. Formation of reactive species in gliding arc discharges with liquid water. J Electrostat. 2006;64:35–43.
- Ikawa S, Tani A, Nakashima Y, et al. Physicochemical properties of bactericidal plasma-treated water. J Phys D Appl Phys. 2016;49:425401.
- Locke BR, Shih K-Y. Review of the methods to form hydrogen peroxide in electrical discharge plasma with liquid water. Plasma Sources Sci Technol. 2011;20:034006.
- Malik MA. Water purification by plasmas: which reactors are most energy efficient? Plasma Chem Plasma Process. 2010;30:21–31.
- Armon R, Kott Y. Bacteriophages as indicators of pollution. Crit Rev Environ Sci Technol. 1996;26:299–335.
- Duran A, Muniesa M, Mendez X, et al. Removal and inactivation of indicator bacteriophages in fresh waters. J Appl Microbiol. 2002;92:338–347.
- Oehmigen K, Hähnel M, Brandenburg R, et al. The role of acidification for antimicrobial activity of atmospheric pressure plasma in liquids. Plasma Process Polym. 2010;7:250–257.
- Oehmigen K, Winter J, Hähnel M, et al. Estimation of possible mechanisms of Escherichia coli inactivation by plasma treated sodium chloride solution. Plasma Process Polym. 2011;8:904–913.
- Traylor MJ, Pavlovich MJ, Karim S, et al. Long-term antibacterial efficacy of air plasma-activated water. J Phys D Appl Phys. 2011;44:472001.
- Rozen Y, Belkin S. Survival of enteric bacteria in seawater. FEMS Microbiol Rev. 2001;25:513–529.
- Padan E, Bibi E, Ito M, et al. Alkaline pH homeostasis in bacteria: new insights. Biochim Biophys Acta Biomembr. 2005;1717:67–88.
- van Elsas JD, Semenov AV, Costa R, et al. Survival of Escherichia coli in the environment: fundamental and public health aspects. ISME J. 2011;5:173–183.